Key Points
- ▪
The lymphatic system has multiple functions including body fluid homeostasis, immune cell trafficking, and transport of lipids.
- ▪
Anatomical lymph drainage occurs from superficial and deep conduits through both in the extremities and lymph egress travels a distal to proximal route.
- ▪
Fluid absorption occurs at the interface of lymphatic capillaries and the interstitium, and once fluid enters the lumen it is termed ‘lymph’.
- ▪
The branched lymphatic network is a unidirectional, blind-ended conduit comprised of lymphatic capillaries, precollectors, collecting ducts, and collecting vessels that drain in that specific sequence.
- ▪
In addition to the immunological function, lymph nodes can regulate lymph transit via lymphovenous connections.
Introduction
The lymphatic system functions as an integral component of both the circulatory and the immune systems. The functions of the lymphatic circulation include: the prevention and resolution of edema; maintenance of interstitial fluid homeostasis, immune traffic (the regulated transit of antigen-presenting cells to the lymphoid organs), and lipid absorption from the gastrointestinal tract. In order to accomplish these various, intricate physiological functions, the lymphatic system relies upon a complex anatomic configuration of vascular conduits that is under exquisite physiological control.
The lymphatic system is a unique and specialized organ system that has historically been poorly understood and to many remains enigmatic. Historically, the closely related vascular system has drawn more attention from a research, anatomic, and clinical perspective. Nevertheless, early investigations of the anatomy and physiology of the lymphatic system, its function, and implications related to cancer have propelled technology and research to elucidate many of the enigmatic characteristics of the lymphatic system. Although Gasparo Aselli is credited with discovering the lymphatic system in 1627, Hippocrates reported “white blood” in nodes and Aristotle described fibers containing colorless fluid observed between blood vessels and nerves. In time, the basic premise of the lymphatics acting as conduits for draining toxins was debunked as cellular, molecular, developmental, and structural biologic studies have shed light on the multiple physiologic functions of the lymphatic system.
Lymphedema, although recognized in some disease processes, is largely overlooked in modern medical and surgical treatment. The science of lymphology is growing rapidly with recent advances in imaging techniques and novel surgical approaches to the treatment of patients with lymphedema. In order to advance the techniques and treatment of such a complex system, it is imperative to understand the basic anatomy and physiology of a normally functioning lymphatic system. This chapter is aimed to provide the readers with fundamental aspects of the architecture and function of the human lymphatic system from a systemic, anatomic, and cellular standpoint.
Lymphatic Embryogenesis
The development of the lymphatic system parallels that of blood vascular structures. It begins after arteriovenous vasculogenesis (vascular development) and differentiation follows. Lymphangiogenesis occurs nearly one month following the development of these structures during embryogenesis. Recently, Srinivasan et al. corroborated Sabin’s proposal that the lymphatic network originates from the embryonic veins. Specifically, maturation and differentiation from the venous system and mesenchyme characterizes the development of the complete lymphatic system. The most elemental unit of the undifferentiated lymphatic system is the lymphatic endothelial cell (LEC), which is morphogenically similar to the blood vascular endothelial cell. Until recently these two cell types were believed to share the same cell markers. However, lymphatic vessel endothelial hyaluronan receptor 1 (Lyve-1) and transcription factor prospero-related homeobox 1 (Prox-1) have been identified as markers of LECs and can be utilized as immunochemical markers of the lymphatic vasculature, i.e., potential markers of lymphangiogenesis. Under the specific influence of growth factors and other stimuli, the lymphatic networks proliferate to form an organized, branched network of lymphatic capillaries (no valves), precollectors (containing valves), collecting ducts (containing valves), and collecting lymphatic vessels (containing valves).
Lymphatic Vessel Structural Anatomy
As a vasculature, healthy lymphatics provide a unidirectional, blind-ended conduit of fluid from the tissue interstitium to the central venous circulation. The initial lymphatics, the most afferent components of the lymphatic vasculature, are in direct contiguity with the intercellular matrix, where fluid gains entry into the lymphatic vasculature. The transition from interstitial matrix to lymphatic space is delineated by the presence of an endothelial lining in the latter; once the fluid gains entry into the lymphatic endothelial-lined cavity of the initial lymphatics, it can be termed ‘lymph’. These initial thin-walled lymphatics can be equivalently termed ‘lymphatic capillaries’ and have a caliber of 30–80 μm. Lymphatic capillaries lack a basement membrane and smooth muscle cells (SMC), solely relying on the elastic fibers of the extracellular matrix for initiation of lymph drainage to reach the precollecting and collecting vessels. The specialized LECs have a distinct oak-leaf shape adorned by unique button-like junctions that characterize these the interfaces among cells and comprise the flap-like portals of entry into the lymphatic capillary. Conformational changes based on fluid and pressure dynamics of the extracellular matrix facilitate the opening and closing of these junctions. These large potential openings facilitate the entry of immune cells and larger structures into the lymph. Pericytes are absent from these circulatory conduits. The endothelial cells of the initial lymphatics are attached to the collagen fibers of the extracellular matrix through specialized anchoring filaments ( Figure 4.1 ). As a result of absent basement membranes and SMCs, they solely rely on the elastic fibers of the extracellular matrix for entry of fluid into the lymphatic lumen.

Egress of lymph proceeds from the lymphatic capillary to precollectors. The initial lymphatics interconnect through a network of precollectors ( Figure 4.2A ). At this level, there is meager coverage with SMCs. The precollectors coalesce into collecting ducts with vasomotor activity. The collecting ducts have thick walls (0.50–0.75 mm diameter), but continue to demonstrate the monolayer of endothelial cells that surrounds the lumen ( Figure 4.2C ). The transition to the collecting vessels, located deeper within the dermis, is demarcated by the presence of a distinct periendothelial smooth muscle cell layer with the intima and media in axial and oblique configuration, a basement membrane, continuous interendothelial junctions and regularly, recurrent, intraluminal bi-leaflet valvular structures ( Figure 4.3 ). The latter separate the lymphatic collecting vessel into distinct functional units called lymphangions. In a healthy lymphatic system, these valvular structures prevent the backflow of lymph.


Drainage Patterns within the Extremity
Within the extremities, lymphatic drainage occurs in parallel through the superficial subdermal network that collects lymph from the skin and subcutaneous tissue, and the deeper subfascial network system that serves muscle, bone, and deep blood vessels. In the upper extremity, these two networks merge in the axilla; comparably, this occurs in the pelvis of the lower extremities. However, while communications along the extremity from the superficial and deep networks have historically been reported, recent studies have failed to elucidate these communicating lymphatics. These parallel pathways for lymph flow are not entirely independent, suggesting that communication along the extremity of superficial and deep systems may be plausible. For example, in states of lymphatic obstruction, the deep system will participate in the lymph drainage of the cutaneous structures. The subdermal lymph transport is markedly more efficient than subfascial flow. When pathology is limited to the deep system, the superficial system does not always manifest clinical signs of lymphedema. A combination of factors may contribute to this phenomenon, including: efficient subdermal drainage, the inability for fluid to accumulate in the deep compartment secondary to the contribution of skeletal muscle contraction, and the likelihood of multiple sites of communication between both systems along the extremity. The deep and superficial system should not be considered as independent systems, since pathology in one can affect the other.
Composition of Lymphatic Fluid and the Lymphatic Pump
Interstitial fluid becomes lymph upon entry into the initial lymphatics. The relative protein content of lymph varies in relationship to the tissue of origin. In many of the body’s parenchymae, the interstitial fluid protein concentration is approximately 2 g/dl; however, the mesenteric protein concentration is closer to 3–4 g/dl, and even this is exceeded by hepatic lymph. The central lymph of the thoracic duct represents an admixture, and therefore approximates protein concentrations of 3–5 g/dl. Under resting conditions, daily lymph formation is approximately 2–3 liters per day.
Entry of interstitial fluid into the lymphatic capillary is governed chiefly by the prevailing interstitial fluid pressure, which in the steady state is typically subatmospheric. At pressures below 6 mmHg, lymph flow becomes negligible. Lymph flow becomes maximal when interstitial pressure is slightly higher than atmospheric pressure. Inasmuch as steady state pressure gradients do not, in fact, favor fluid entry into the lymphatic capillary, it is postulated that dynamic, cyclical changes in the pressure gradients transiently promote the necessary fluid flux into the lymphatic space.
In distinction to the local pressure gradients that favor initial uptake of interstitial fluid by the lymphatic capillary, there exist systemic driving forces for the basal propulsion of lymph. Flow within the lymphatic collectors is dependent primarily upon intrinsic, rhythmic contraction of the lymphangions (the segments of the lymphatic vessel demarcated by two adjacent sets of valves).
The intrinsic lymph pump relies upon the coordinated, rapid strong contractions generated by lymphatic muscle cells. These contractions produce a rapid reduction of the lymphatic diameter, a decrease in lymphatic compliance, an increase in the local lymph pressure, closure of the upstream valve, opening of the downstream valve, and the ejection of lymph into the next, downstream lymphangion. The phasic contractions are initiated by the activity of an electrical pacemaker located within the muscle layer of the lymphatic wall.
In addition to the intrinsic forces that govern lymph flow, the rate of lymph transport can also be substantially altered by physical and humoral factors that affect the rate and amplitude of the intrinsic contractions. Beta-adrenergic agonists decrease the frequency and force of spontaneous contractions in bovine mesenteric lymphatic vessels. Reactive oxygen species and endothelium-derived nitric oxide can reduce lymphatic phasic contractions. Lymphatic contractility and flow will augment in response to tissue edema (the edema safety factor), positional increases in hydrostatic pressure, exercise, massage and pneumatic compression, and temperature changes. Noncoincidentally, many of these factors are modulated in the conservative therapeutic approach to reducing lymphatic edema.
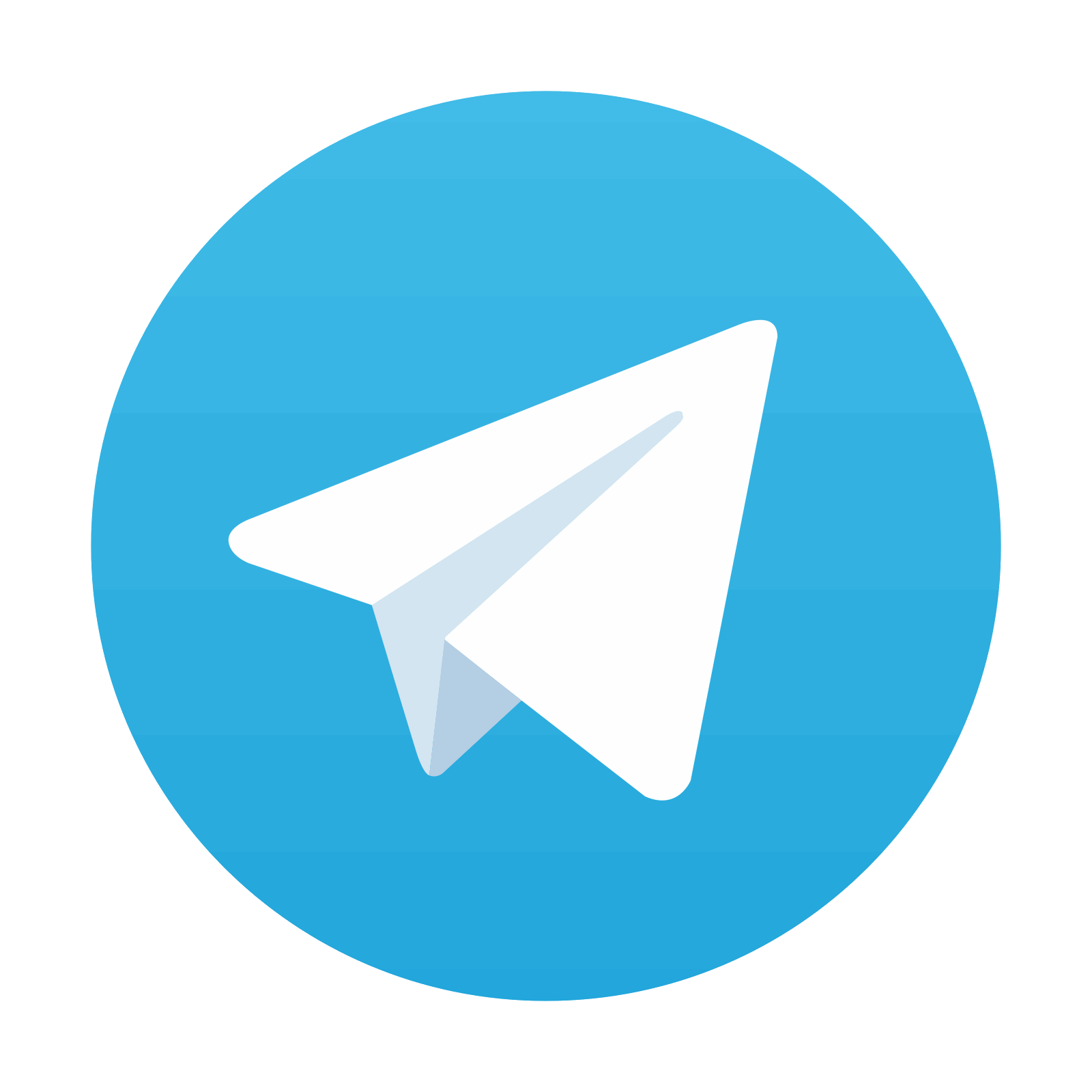
Stay updated, free articles. Join our Telegram channel

Full access? Get Clinical Tree
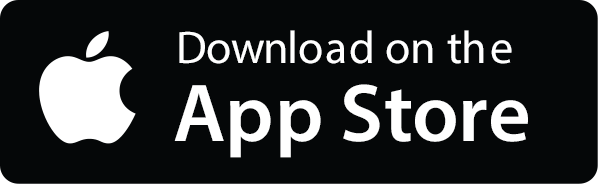
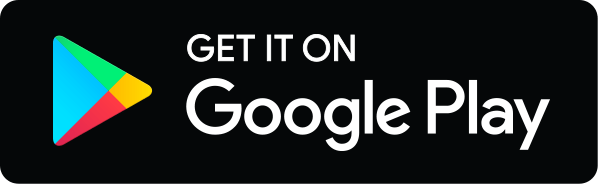