This article discusses adipose-derived stem cell (ASC) biology, describes the current knowledge in the literature for the safety and regulation of ASCs, and provides a brief overview of the regenerative potential of ASCs. It is not an exhaustive listing of all available clinical studies or every study applying ASCs in tissue engineering and regenerative medicine, but is an objective commentary of these topics.
Key points
- •
Human adipose tissue is now a widely accepted source for stem cells in regenerative medicine, and has been the subject of preclinical studies and clinical studies directed toward numerous applications.
- •
The nonlipid cell population isolated from adipose tissue is heterogeneous and contains adipose-derived stem cells (ASCs), which can be isolated and cultured.
- •
ASCs are of mesenchymal lineage and manifest features that are attractive for regenerative therapy approaches, including multipotency and release of growth factors that can induce tissue healing.
- •
These beneficial characteristics have the potential to affect cancer growth and this issue is still being investigated in preclinical and clinical studies.
- •
From a regulatory perspective, adipose therapies may be regulated under the category of human cells, tissues, and cellular and tissue-based products by the US Food and Drug Administration in Title 21 Code of Federal Regulations, part 1271, or as a biologic drug under section 351 of the Public Health Services Act, depending on the specific use and the degree of processing.
- •
To date, 129 active clinical trials are listed in the US National Institutes of Health Web site ( www.clinicaltrials.gov ), spanning a broad range of applications including arthritis, intervertebral disc degeneration, autism therapy, cell-enriched fat grafting, pulmonary disease, and numerous clinical targets.
Isolation and characterization
Adipose-derived stem cells (ASCs) are prevalent surrounding the blood vessels and within the connective tissue of human adipose tissue. These non–lipid-laden stromal cells can be isolated from either suction-aspirated adipose tissue or excised human fat by enzymatic collagenase digestion. Passenger erythrocytes can be lysed selectively, and numerous descriptions for cell isolation methods appear in the literature. The freshly isolated cell pellet is highly heterogeneous and is named the stromal vascular fraction (SVF). Further discussion of the SVF components is featured later. If the SVF cells are placed in culture, the ASCs adhere to the surface of an untreated tissue culture flask after 6 to 8 hours’ incubation at 37°C and 5% CO 2 . Once ASCs have adhered to the culture flask surface, nonadherent populations, representing 7% to 15% of the SVF and representing mainly hematopoietic origin cells, are washed away with sterile phosphate buffered solution and/or fresh culture media. Fig. 1 shows a widely accepted protocol for SVF and ASC isolation. A commonly used ASC expansion medium consists of a Dulbecco’s Modified Eagle Medium (DMEM) and DMEM/F12 media combination, with 10% serum, antibiotic (eg, penicillin, streptomycin), and a small amount of dexamethasone to prevent differentiation to another mesenchymal lineage. Once in culture, specific growth factors or other additives can be applied to direct the differentiation to a specific phenotype, such as adipose, bone, cartilage, or muscle.
SVF is attractive therapeutically because it may be obtained from tissue within 60 to 90 minutes, and the isolation can be performed in a clean room near an operating room, or even in an operating room using an automated device. Collagenase digestion results in approximately 2 × 10 5 to 5 × 10 5 nucleated SVF cells per gram of adipose tissue. However, the complete ASC isolation process takes 20 to 24 hours and requires cell culture facilities. Reasons to culture the cells include the ability to expand the cell number, select for specific subpopulation, or control the microenvironment for directed differentiation or induction of adherence to a scaffold material. Flow cytometry characterization of the surface markers on freshly isolated and cultured adipose-derived cells can be performed, and shows the presence of early progenitor markers such as cluster of differentiation 34 (CD34) and CD90.
Nonenzymatic cell isolation has been a topic of interest, driven by a potentially less restrictive regulatory pathway. This strategy has focused on mechanical forces such as ultrasound. However, there is no strong evidence to suggest that this is equivalent to enzymatic digestion. There has also been interest in whether there are viable ASCs in the aqueous portion of the liposuction aspirate without exposure of the tissue to enzymes. Although some cells may be present, the quantity is so low as to preclude clinical utility. Because the ASCs are firmly embedded within the connective tissue, enzymatic digestion is necessary to release them in significant quantity.
Preadipocytes were first described in 1976 by Dardick and colleagues, beginning with rat models then isolated from human tissues. Isolated preadipocytes were used to study adipocyte biology in vitro, leading to recognition of different anatomic locations and adipose depots to express different biological characteristics, such as adipocyte size and lipolytic potential. In 2001, Zuk and colleagues first discussed the differentiation plasticity of preadipocytes and eventually the term ASC was adopted to encompass the characteristics of self-renewal, asymmetric division, and multipotency. Although ASCs proliferate rapidly in culture in vivo, growth factors are necessary to induce lineage-specific differentiation. Differentiation of ASCs to other mesenchymal phenotypes has been well established both in vitro and in vivo. ASC differentiation to cell lineages of the ectodermal and endodermal germ layers has also been successful in several studies. Gerlach and colleagues described adipogenesis within a hollow fiber–based, three-dimensional, dynamic perfusion bioreactor in which ASCs are differentiated and maintained as functional, mature adipocytes long term ( Fig. 2 ).
The SVF population (the freshly isolated population) derived from adipose tissue has diverse patterns of cell surface markers as identified by flow cytometry. Zimmerlin and colleagues identified several similar cell surface markers in ASCs (the cultured population) compared with bone marrow–derived stem cells (BMSCs) and these are described in Table 1 . Li and colleagues reported 4 subpopulations of interest that can be isolated and cultured ( Fig. 3 ). The first is CD31 + /34 – , which is classified as mature endothelial, with the endothelial marker of CD31, lacking the progenitor marker of CD34. The second subpopulation is classified as “endothelial stem,” and CD31 + /34 + . A third subpopulation, CD34 +/ 31 – is classified as the adipose stem cell group. The fourth subpopulation represents a pericyte group and is characterized by surface markers CD146 + /90 + /31 – /34 – . These cells reside adjacent to the blood vessel walls, and are shown by immunostaining. Similar to BMSCs, ASCs do not express major histocompatibility complex (MHC)-II and inhibit proliferation of activated peripheral blood mononuclear cells, suggesting a role for modulating the immune system in inflammatory disorders or allogeneic transplantation.
ASCs | BMSCs | |
---|---|---|
Negative | CD38, CD45, CD106, HLA-DR, DP, DQ (MHC class II), CD80, CD86, CD40, and CD40L (CD154) | CD34, CD38, CD45, and fox antigens involved in immunologic signal transduction such as HLA-DR, DP, DQ (MHC class II), CD80, CD86, CD40, and CD40L (CD154) |
Positive | CD13, CD29, CD34, CD44, CD73, CD90, CD105, CD166, MHC class I, HLA-ABC | CD13, CD29, CD44, CD73, CD90, CD105, CD166, MHC class I, HLA-ABC |
Isolation and characterization
Adipose-derived stem cells (ASCs) are prevalent surrounding the blood vessels and within the connective tissue of human adipose tissue. These non–lipid-laden stromal cells can be isolated from either suction-aspirated adipose tissue or excised human fat by enzymatic collagenase digestion. Passenger erythrocytes can be lysed selectively, and numerous descriptions for cell isolation methods appear in the literature. The freshly isolated cell pellet is highly heterogeneous and is named the stromal vascular fraction (SVF). Further discussion of the SVF components is featured later. If the SVF cells are placed in culture, the ASCs adhere to the surface of an untreated tissue culture flask after 6 to 8 hours’ incubation at 37°C and 5% CO 2 . Once ASCs have adhered to the culture flask surface, nonadherent populations, representing 7% to 15% of the SVF and representing mainly hematopoietic origin cells, are washed away with sterile phosphate buffered solution and/or fresh culture media. Fig. 1 shows a widely accepted protocol for SVF and ASC isolation. A commonly used ASC expansion medium consists of a Dulbecco’s Modified Eagle Medium (DMEM) and DMEM/F12 media combination, with 10% serum, antibiotic (eg, penicillin, streptomycin), and a small amount of dexamethasone to prevent differentiation to another mesenchymal lineage. Once in culture, specific growth factors or other additives can be applied to direct the differentiation to a specific phenotype, such as adipose, bone, cartilage, or muscle.
SVF is attractive therapeutically because it may be obtained from tissue within 60 to 90 minutes, and the isolation can be performed in a clean room near an operating room, or even in an operating room using an automated device. Collagenase digestion results in approximately 2 × 10 5 to 5 × 10 5 nucleated SVF cells per gram of adipose tissue. However, the complete ASC isolation process takes 20 to 24 hours and requires cell culture facilities. Reasons to culture the cells include the ability to expand the cell number, select for specific subpopulation, or control the microenvironment for directed differentiation or induction of adherence to a scaffold material. Flow cytometry characterization of the surface markers on freshly isolated and cultured adipose-derived cells can be performed, and shows the presence of early progenitor markers such as cluster of differentiation 34 (CD34) and CD90.
Nonenzymatic cell isolation has been a topic of interest, driven by a potentially less restrictive regulatory pathway. This strategy has focused on mechanical forces such as ultrasound. However, there is no strong evidence to suggest that this is equivalent to enzymatic digestion. There has also been interest in whether there are viable ASCs in the aqueous portion of the liposuction aspirate without exposure of the tissue to enzymes. Although some cells may be present, the quantity is so low as to preclude clinical utility. Because the ASCs are firmly embedded within the connective tissue, enzymatic digestion is necessary to release them in significant quantity.
Preadipocytes were first described in 1976 by Dardick and colleagues, beginning with rat models then isolated from human tissues. Isolated preadipocytes were used to study adipocyte biology in vitro, leading to recognition of different anatomic locations and adipose depots to express different biological characteristics, such as adipocyte size and lipolytic potential. In 2001, Zuk and colleagues first discussed the differentiation plasticity of preadipocytes and eventually the term ASC was adopted to encompass the characteristics of self-renewal, asymmetric division, and multipotency. Although ASCs proliferate rapidly in culture in vivo, growth factors are necessary to induce lineage-specific differentiation. Differentiation of ASCs to other mesenchymal phenotypes has been well established both in vitro and in vivo. ASC differentiation to cell lineages of the ectodermal and endodermal germ layers has also been successful in several studies. Gerlach and colleagues described adipogenesis within a hollow fiber–based, three-dimensional, dynamic perfusion bioreactor in which ASCs are differentiated and maintained as functional, mature adipocytes long term ( Fig. 2 ).
The SVF population (the freshly isolated population) derived from adipose tissue has diverse patterns of cell surface markers as identified by flow cytometry. Zimmerlin and colleagues identified several similar cell surface markers in ASCs (the cultured population) compared with bone marrow–derived stem cells (BMSCs) and these are described in Table 1 . Li and colleagues reported 4 subpopulations of interest that can be isolated and cultured ( Fig. 3 ). The first is CD31 + /34 – , which is classified as mature endothelial, with the endothelial marker of CD31, lacking the progenitor marker of CD34. The second subpopulation is classified as “endothelial stem,” and CD31 + /34 + . A third subpopulation, CD34 +/ 31 – is classified as the adipose stem cell group. The fourth subpopulation represents a pericyte group and is characterized by surface markers CD146 + /90 + /31 – /34 – . These cells reside adjacent to the blood vessel walls, and are shown by immunostaining. Similar to BMSCs, ASCs do not express major histocompatibility complex (MHC)-II and inhibit proliferation of activated peripheral blood mononuclear cells, suggesting a role for modulating the immune system in inflammatory disorders or allogeneic transplantation.
ASCs | BMSCs | |
---|---|---|
Negative | CD38, CD45, CD106, HLA-DR, DP, DQ (MHC class II), CD80, CD86, CD40, and CD40L (CD154) | CD34, CD38, CD45, and fox antigens involved in immunologic signal transduction such as HLA-DR, DP, DQ (MHC class II), CD80, CD86, CD40, and CD40L (CD154) |
Positive | CD13, CD29, CD34, CD44, CD73, CD90, CD105, CD166, MHC class I, HLA-ABC | CD13, CD29, CD44, CD73, CD90, CD105, CD166, MHC class I, HLA-ABC |
Use of adipose-derived stem cells to enhance fat grafting
To improve results in fat transfer, a clinical strategy has been developed in which autologous SVF cells are admixed with the fat graft to promote graft volume retention and neovascularization. Named cell-assisted lipotransfer (CAL), the appeal of ASC incorporation in lipoinjection is mostly because of growth factor release of the stem cells, especially angiogenic growth factors such as vascular endothelial growth factor. Another hypothesis supporting ASC addition to the fat graft includes the concept that ASCs provide a scaffold on which additional stem cells can organize and differentiate. This strategy has further appeal because the SVF can be isolated at the point of care.
The first randomized controlled study to show the efficacy of this strategy in humans was published in 2013 by Kølle and colleagues. Human volunteers received 2 fat grafts, one in each upper extremity, both with and without supplemental stem cells. The grafts admixed with stem cells showed significantly higher volume retention. This study provided proof of principle in a clinical trial, but had several notable criticisms. First, the cell dose was exceedingly high, with 2 × 10 7 cells admixed with each milliliter of fat injected. Second, the ability to obtain such a high cell dose required expansion of the harvested SVF in cell culture, which involves high cost and regulatory hurdles beyond the use of SVF alone. In addition, culturing cells requires a separate fat harvest procedure. Third, the fat graft was administered as a 30-cm 3 bolus injection, rather than as a fanning technique that disperses the fat graft within the recipient bed. The concept of CAL is being used for aesthetic and reconstructive applications across the body. The question of cell dose, or the minimum quantity of nucleated SVF cells that needs to be added to each milliliter of graft for clinical effect, remains an area of study.
Interactions between adipose-derived stem cells and tumor cells
The same properties of that make ASCs useful for tissue healing and regeneration also create the potential for the stimulation of tumor cell growth when the cells are used for cancer reconstruction. Characteristics frequently shared between tumor progression and successful regenerative therapies intended to restore functional tissue include cellular homing, immunosuppression, revascularization, and tissue growth promotion.
In addition to the direct secretion of angiogenic growth factors, which could induce a nourishing vascular supply to tumors, ASCs secrete large quantities of leptin and adipsin. Leptin increases angiogenic and proliferative signaling in estrogen receptor–positive and estrogen receptor–negative breast cancer cell lines. Adipsin has been proved to increase the concentration of acylation-stimulating protein, which is another adipose-derived hormone that regulates triglyceride synthesis.
Zimmerlin and colleagues reviewed the mesenchymal stem cell (MSC) secretome and regenerative therapy after cancer, outlining direct and indirect secretome properties in connection with MSC paracrine effects and differentiation, and summarized MSC–tumor cell interactions. These properties were not known when autologous fat grafting was first pioneered at the end of the nineteenth century.
With an in vitro coculture model, Zimmerlin and colleagues confirmed an enhancing effect of ASCs on the proliferation of breast cancer cells (proliferating but not dormant cells), confirming the results of several other studies proving MSC presence can stimulate tumor cell growth. In addition, more evidence has accumulated suggesting that clonogenic tumor cells share many characteristics with adult tissue stem cells, including self-renewal.
In their review article analyzing risks of regenerative therapy after cancer, Donnenberg and colleagues distinguished between 2 potentially tumorigenic populations, resting (dormant) and active (proliferating) tumor cells, and the parallels that may be associated with adult tissue stem cells. They hypothesized that the driving stimuli pushing dormant stem–like cancer cells into an active tumorigenic state are distinct from the stimuli that favor the survival and proliferation of active progenitorlike tumor cells. Such a hypothesis guides the concept that introducing proangiogenic autologous MSCs after tumor resection when the region is clinically disease free would not contribute to local recurrence and, if increased local vascularization at the tumor site was an independent risk factor for recurrence, it would become more clear after observing patients reconstructed with well-vascularized tissue flaps. Immediate autologous tissue flap reconstruction has not been associated with heightened recurrence rates. Therefore, stem cell–based therapies for reconstruction after cancer would be safe if the patient is clinically disease free.
Although in vitro studies suggest that ASCs stimulate tumor growth, the situation of high concentrations of stem cells in close proximity with tumor cells ex vivo may not accurately model the clinical scenario of CAL for breast reconstruction, and the clinical significance of these studies remains unclear. The question will best be answered in large clinical trials. To date, large patient series of autologous fat transfer for breast reconstruction, representing the application of both adipocytes and native adipose stem cells to a tumor bed, show either no increased rate of recurrence or a relationship only in a subgroup of young patients with highly aggressive tumors.
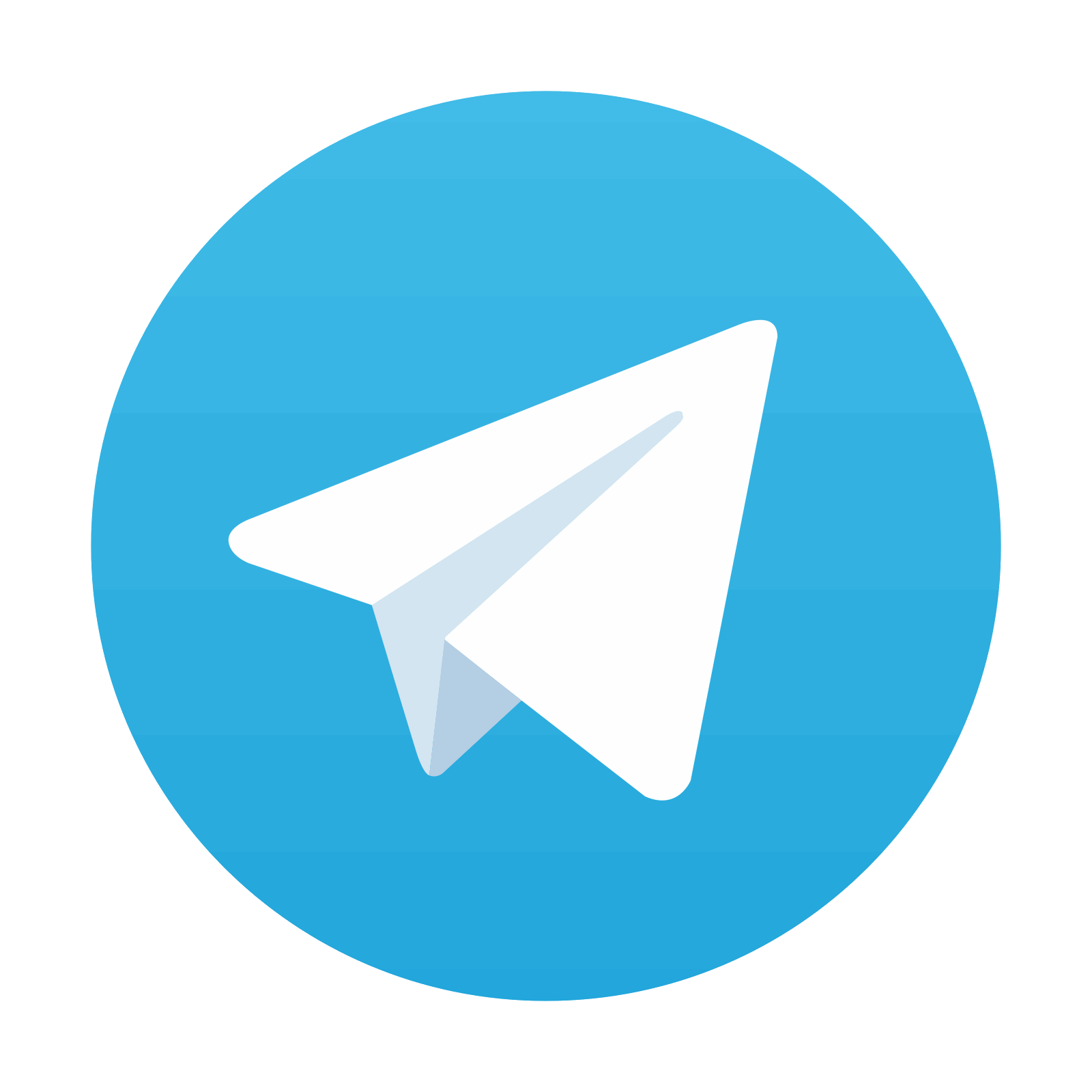
Stay updated, free articles. Join our Telegram channel

Full access? Get Clinical Tree
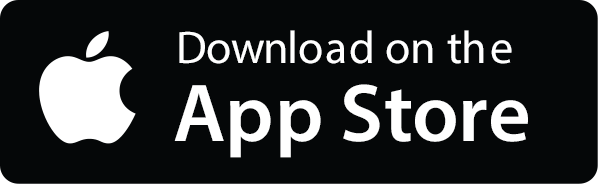
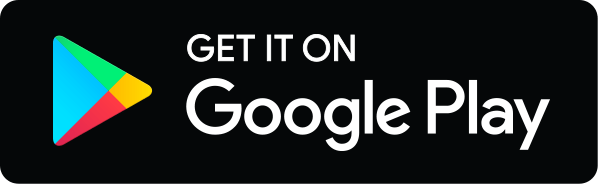