Fig. 10.1
(a) Keloid of the lobule; (b) hypertrophic scar after burn injury
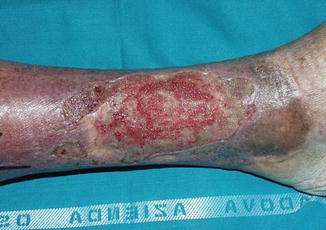
Fig. 10.2
An example of chronic skin ulcer
Hypertrophic scars and keloids represent fibrotic skin conditions which can often be difficult to treat and can lead to a functional impairment, especially in burn patients (Fig. 10.1). Clinicians define hypertrophic scar as a scar that does not grow beyond the boundaries of the original wound, whereas keloid scar grows horizontally (Table 10.1). Pathologists distinguish keloids from hypertrophic scar histologically on the basis of thick eosinophilic collagen bundles that are absent in hypertrophic scar. Hypertrophic scars improve naturally and gradually, although the full maturation process may take up 2–5 years, whereas keloids do not resolve naturally [1–3].
Table 10.1
Hypertrophic versus keloid scar
Hypertrophic scar | Keloid |
---|---|
In hypertrophic scar the collagen fibers appear as delicate filaments that are of fairly regular thickness and are arranged in definite nodular structures resembling those characteristics of fibromatosis such as in Dupuytren’s or Ledderhose’s diseases. Typical myofibroblasts rich in peripheral microfilament bundles are seen; they are associated with banded collagen fibers immediately adjacent to the cell surface. | In keloids the collagen fibers appear much thicker and more irregular than in normal dermis; they show a very coarse arrangement without any nodular or fascicular disposition. The fibroblast has a classical appearance with cisternae of rough endoplasmic reticulum; collagen fibrils are separated from the cell membrane of the fibroblast by an electron transparent substance surrounding the entire cell. |
Chronic wounds include different pathological conditions (Table 10.2), but have a common biological basis characterized by an entrapment in a constant inflammatory phase due to a failure to proceed through the subsequent stages of wound healing (Fig. 10.3). This inflammatory state leads to an abnormal cellular and biochemical microenvironment and a remarkable discomfort for the patient associated with chronic disability.
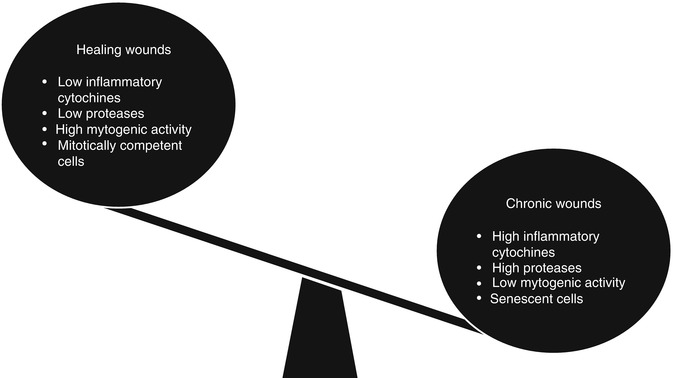
Table 10.2
Some important causes of skin ulcers
Arterial occlusive disease |
---|
Venous insufficiency/stasis dermatitis |
Pressure |
Vasculitis |
Cutaneous involvement by internal malignancy (e.g., lymphoma) |
Connective tissue diseases |
Skin malignancy (squamous and basal cell carcinoma) |
Primary skin infection (e.g., fungal—sporotrichosis) |
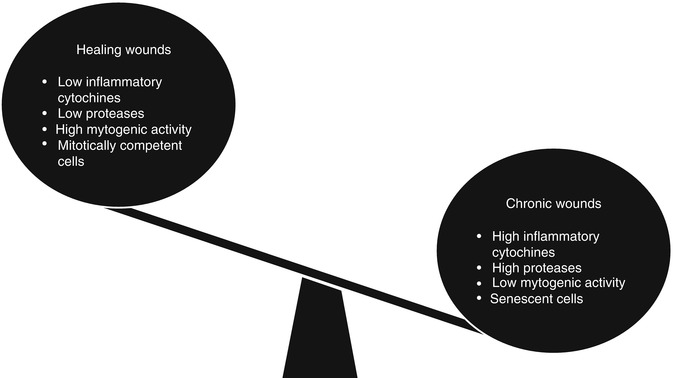
Fig. 10.3
Biochemical microenvironment is abnormal in chronic wounds and leads to an entrapment of the wound healing process
The aim of the treatment of the above pathological conditions using adipose-derived stem cells (ADSC) is to reestablish a physiological, biochemical, and cellular milieu; thanks to the adjunct of healthy undifferentiated cells that are able to secrete growth factors stimulating the restart of the orchestrate process of wound healing [4–6].
Nowadays the interest focused on the regenerative properties of the ASCs has particularly grown. This popularity derives from the multi-potency of these precursors which have the potential to differentiate into cells of the mesenchymal lineage like the bone, fat, cartilage, or muscle. The ASCs usually are located in the stromal-vascular fraction of enzymatically digested fat tissue, either from the whole fat or from lipo-aspirated fat, and they can be collected together after a series of filtration, collagenase digestion, and centrifugation. Once assembled, they could be used to promote the regenerative healing in order to stimulate angiogenesis and to produce cytokines. Moreover, it seems that ASCs can provide vascular stability and the possibility to intercommunicate with endothelial cells as pericytes. All these findings suggest that it is possible to use ASCs to improve a better healing [7–10].
10.2 Adipose-Derived Stem Cells to Treat Hypertrophic Scar
According to the American Burn Association, there are about half a million burns that require medical care in the United States each year. Early burn progression and inadequate systems of measurement complicate assessment of burn depth. Many deep partial-thickness burns are therefore treated without surgery. Left to heal without excision and skin grafting, burns often cause deep fibrotic scars are characterized by abnormal color, texture, thickness, and flexibility. When untreated burns are found in aesthetically sensitive areas, this can cause significant deformity. A number of topical and minimally invasive techniques have been used in an attempt to improve the quality and the appearance of scars caused by burns. Treatment options include silicone gel for topical use, pressure dressings, corticosteroids, and, more recently, autologous fat grafting [11–14].
Over the past decade, the tissue engineering community has come to recognize the regenerative potential of adipose tissue. In general, plastic surgeons have translated this revelation in clinical applications. Autologous fragments of fat have been used in a number of settings to improve the quality of the overlying tissues. In one study, the clinical improvement of hypertrophic burn scars was observed following autologous fat placed beneath the site [15–17].
Using a model of murine fat graft in combination with a previously described murine model of thermal injury, researchers determined the mechanism by which fat grafting can result in a change within a burn scar. To this end, the markers of neovascularization and fibrosis were compared in mice fats and treated with saline solution in the following two-point rate thermal damage [18, 19].
Fat or sham graft was performed 2 weeks after thermal injury using a previously described murine model of fat grafting. This time point was chosen because a firm eschar formed at the site of burning within 2 weeks after injury. The mice were anesthetized before the rear part of their dorsal skin was shaved and prepared aseptically. A small incision was made on the posterior midline of the back. Discarded human fat (IRB # H12756-01b) was prepared using the Coleman technique. Fat highest density has been divided into 1 mL syringes with a Luer-Lock transfer system (Luer-Lock LL—code LL12, Mentor, Santa Barbara, CA, USA). The fat was then infiltrated under the dorsal skin of the mouse using a style-1 Coleman infiltration cannula (code COL-17, Mentor, Santa Barbara, CA, USA). Each animal was diffusely infiltrated with 1.5 mL of fat. The incision was then closed with 4/0 suture nylon. Sham-grafted animals were treated identically, but 1.5 mL of sterile saline was injected in place of fat. The animals were allowed to recover under a heat lamp before being returned to the animal facility supervised [20–24]. CD31 staining 4 weeks after grafting demonstrated significantly higher vascular density surrounding the burn wound in fat-grafted animals compared with saline-treated animals. Eight weeks after grafting, the vascular density in the tissue surrounding the burn wound was again significantly greater in fat-grafted animals than saline-grafted animals [25–27]. Eight weeks after grafting, burn scars collected from animal fat grafts demonstrated a significantly lower rate of burn scars than those collected from saline-grafted animals.
Applying mouse model of fat grafting in the context of thermal damage caused accelerated revascularization of burn scar tissue and, ultimately, decreased fibrosis. A photography serial demonstrated an improvement in the consistency and flow rate of burn scars grafted with fat in place of the saline solution. Revascularization of the burn scar, as measured by scanning laser Doppler and CD31 staining, was significantly more advanced in animal fats grafted 4 weeks after grafting [28–31]. Eight weeks after grafting, saline-grafted animals were revascularized almost to the same extent as the fat-grafted animals, as demonstrated by scanning laser Doppler. Early revascularization in animal fat grafts demonstrated important, however, in the prevention of fibrosis in successive moments. Eight weeks after grafting animal fat, grafted fibrosis demonstrated significantly lower compared to saline-grafted controls. Significantly decreased markers of fibrosis have been found in both ELISA and PCR array, as well, corroborating this histological discovery (e.g., a 77 % decrease in expression of TGF-β mRNA).
To understand the clinical significance of early revascularization in a burn wound, it is essential to consider first burn pathophysiology. Following a burn, thrombosis in the microcirculation causes ischemia in an area known as the zone of stasis. In response, endothelial progenitor cells (EPCs) are mobilized from the bone marrow. EPCs are known to home to areas of ischemia and differentiate into endothelial cells, which are instrumental in the formation of blood vessels. The concentration of EPCs in circulation peaks about 24 h following a burn and falls significantly later, however, returning to baseline levels within 72 h. It has also been shown that burns of increasing severity result in the delayed release and decrease of EPCs in circulation. As a result, many burns revascularize slowly, and, in turn, mature hypertrophic burn scars demonstrate their functional changes in the microcirculation [32–35].
Microcirculatory abnormalities play an important role in scar architecture as low oxygen tension induces an upregulation of TGF-SS1. This factor is an important regulator of the production of collagen and, when unchecked, fibrosis. Moreover, hypoxia alters the way in which TGF-β1 interacts with the MMP, a family of proteins that regulate the remodeling of the collagen. It was therefore suggested that any action that improves vascularity and, by extension, oxygenation of a burn scar would similarly improve the quality of the scar and texture. Early revascularization of a burn scar can then protect the wound bed by upregulation of TGF-β1 and from disorders resulting in the synthesis of collagen in the initial stage of scar formation [36–39].
This study has several limitations that should be known: in the first place, additional experiments will be required to determine the precise mechanism by which the autologous fat accelerates revascularization in burns. The potential for adipose-derived stem cells to act as endothelial progenitor cells and promoting neovascularization has been described above, however. Secondly, a larger animal model (e.g., the red Duroc skin model, porcine or rabbit ear model) will be required to more fully explore the phenotypic effect of fat grafting in thermal injury. Finally, it should be noted also that the use of wild-type FVB mice (instead of immunocompromised animals) does not lead to an immune reaction against the grafts of human fat. This phenomenon is not well understood, and further studies were designed to address it.
Despite the limitations of this study, however, the mouse model chosen was sufficient to demonstrate the positive effect of fat grafting on markers of vascularization and fibrosis as a result of full-thickness thermal injury. This study, therefore, speaks of the potential for fat grafting as early intervention and minimally invasive option for severe burns left to heal by secondary intention or, potentially, for partial-thickness burns in which excision and skin grafting cannot be done, but do not seem to have the potential for hypertrophic scars. In these cases, early revascularization can produce a significant improvement in the possible quality of the burn scar. Fat grafting can be very useful, in addition to skin grafting in cases of severe burns [5, 17, 40–42].
Infiltration of transformed human lipoaspirate under subacute burns in mice led to significant changes in wound healing, in particular accelerated revascularization. In turn, this has led to the downregulation of the respiratory fibrosis with resulting improvements in terms of quality of the scar. Although these results should be reviewed models in larger animals, fat grafting can in fact be applied in the treatment of thermal injury in the future [43].
10.3 Adipose-Derived Stem Cells to Treat Chronic Wounds
Recently ASCs have been used in studies for wound healing with the aim to reconstruct soft tissues, all because of their potential to promote angiogenesis, to secrete cytokines, and to differentiate in various cells type, as mentioned above. Ebrahimian et al. showed that ASCs improve wound healing by their differentiation into keratinocytes and by the production of VEGF and KGF which are the main actors during the epithelial morphogenesis. Moreover they demonstrated that the treatment with ASCs is worth it for both irradiated and nonirradiated wounds on mice. Another role of adipose-derived stem cells was clarified by Kim et al. who described an augmented fibroblast proliferation and wound reepithelialization due to both the stimuli offered by the direct cell-to-cell contact between ASCs and the other cells involved in the healing process [44–46].
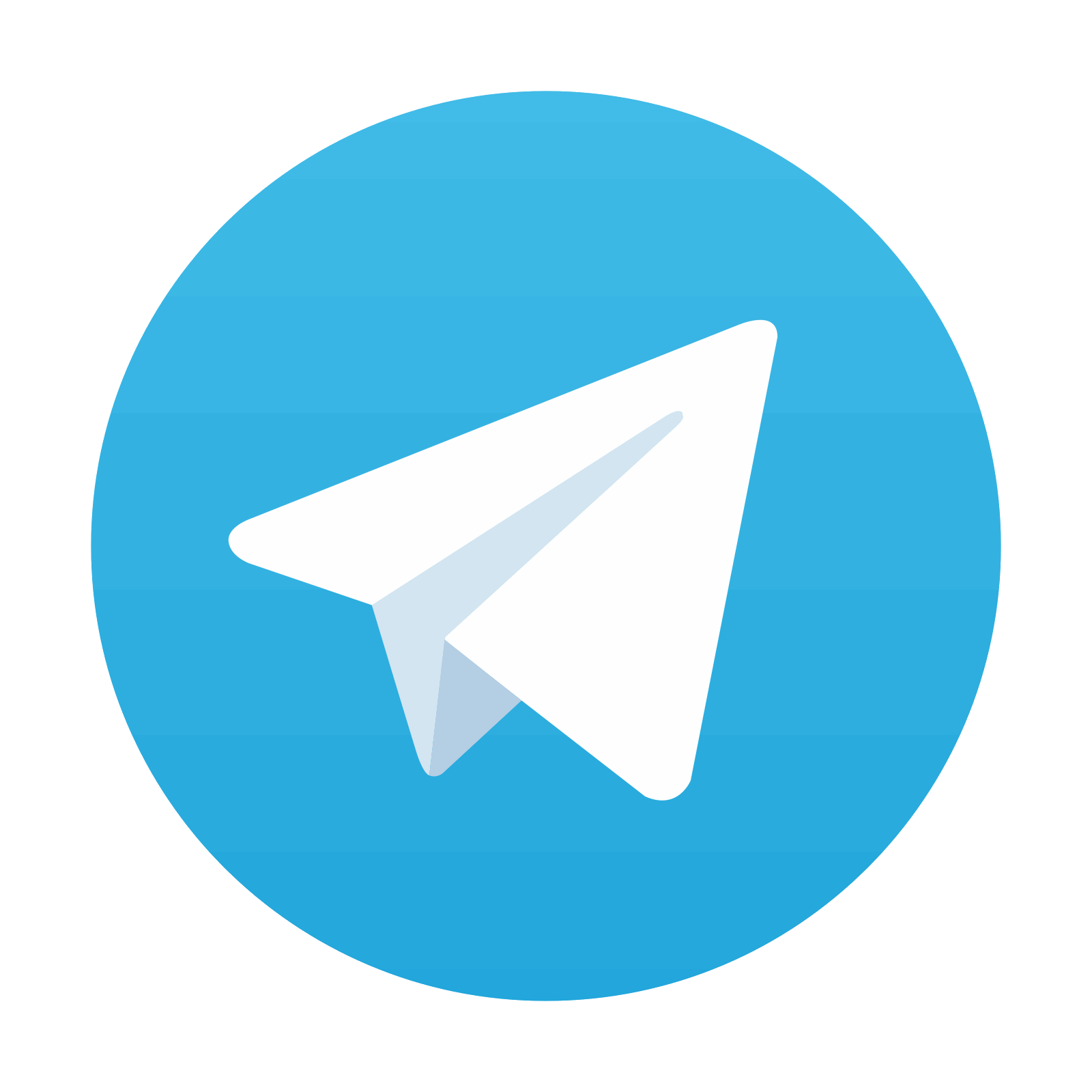
Stay updated, free articles. Join our Telegram channel

Full access? Get Clinical Tree
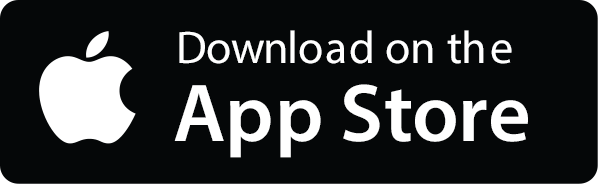
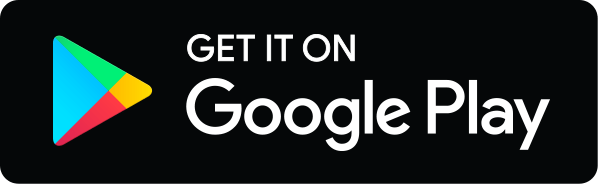