5. Energy-Based Treatments for Facial Aging
5.1 Introduction
The growth in use of energy-based devices to treat facial aging has been accelerating, in part because of the minimal or absent recovery time and more limited skill and experience required to render these treatments. The expanding ability to produce patterns of tissue injury and remodeling that cannot be matched by any other technique, however, makes an understanding of energy-based devices essential for anyone who wants to be a master at treating the aging face in the twenty-first century. Several types of energy are in widespread use, deployed by multiple manufacturers in dozens of clinically approved devices. These devices are being applied in the labeled indications and extensively in off-label indications as well. Given this morass of technology, it is impossible to discuss each device both individually and specifically. Instead, we will try to explain the principles that underlie the current treatments and the clinical concepts essential to applying them safely and effectively.
5.2 A Warning about Parameters
We endeavor in this chapter to present the tissue effects produced by the devices rather than give specifics about the laser or energy parameters. In some instances, these are provided for accuracy or completeness. These parameters relate to the individual device by the individual manufacturer that was used to produce them and may not be cross-applied to a different brand of device, even one using the same wavelength of laser light or the same general strategy of treatment, for example, erbium:yttrium aluminum garnet (Er:YAG) fractional resurfacing. Just as each brand of botulinum toxin has been given a unique generic name since it is viewed by the U.S. Food and Drug Administration (FDA) as being an individual unique pharmaceutical, energy devices increasingly possess unique delivery regimens and patterns of tissue effect that are distinctly different from those of alternative brands in the same class of product. For this reason, there is often no generic alternative to a given device. Use of parameters appropriate for one device can routinely produce disastrously adverse outcomes if applied with an alternative competing device. For this reason, it is imperative to receive specific training in appropriate parameters for each clinical indication from the manufacturer of the specific devices you purchase or use or from a physician experienced in using the device.
5.3 Indications for Energy-Based Treatments in Facial Aging
5.3.1 Cutaneous
Multiple cutaneous indications are currently treated with energy-based devices. These include skin surface conditions, largely epidermal, such as pigmentary changes from accumulated photodamage and texture or roughness. Treating deeper, into the dermis, allows rhytids and vascular changes to be addressed.
Solar lentigines and pigment variation are readily addressed using a variety of energy-based strategies. A substantial improvement in appearance can be obtained just from treating this single issue.
Telangiectasias, angiomas, and rosacea can be treated using energy-based devices. Rosacea, which is never cured but only controlled, can be treated with a combination of coagulative treatments and pharmacotherapy.
Laser resurfacing has become the treatment of choice for rhytids in our opinion because of the tremendous precision and control of depth of effect and very low complication rates. These treatments can extend from full-field superficial resurfacing of the epidermis to deep full-field dermal resurfacing to fractional treatments, which allows grading of the aggressiveness of the treatment to tailor to individual patient needs based on depth and severity of rhytids and patient limits on acceptable recovery time. Newer devices allow combination therapy for even better results with limited downtime.
Nonsurgical devices for treating skin laxity have been in use over the last decade. Although the FDA has not accepted any workable definition of skin tightening, there are clearances for skin or facial lifting. These treatments provide modest improvement in laxity in a significant portion of patients and appear to work more effectively in younger patients with more robust wound-healing responses. The downside to these treatments is the wide range of efficacy, including a sizable percentage of nonresponders. Whereas these treatments are in no way a substitute for surgical facialplasty, they represent a legitimate option for many clinical circumstances and are likely to expand substantially in the future because of patient demand and technological advances.
Another area where devices can potentially help the aging face, which is not widely discussed, is changing skin quality and thickness. After surgical lifting with facialplasty, the patient is still left with the photodamaged elastotic skin. Both resurfacing and coagulation injury and remodeling treatments result in increased skin thickness, tissue remodeling of collagen to a more organized and highly dense state, and in the case of resurfacing, removal of a portion of the actinic tissue. Upregulation of various cytokines and enzymes, such as matrix metalloproteinases from thermal and low-level light effects, has been demonstrated experimentally, although the clinical significance of these changes is unknown at this time. The extent to which these changes produce a skin that behaves and looks more like younger skin is unknown but results are promising.
Finally, devices can be used to reduce postsurgical sequelae, such as edema, ecchymosis, erythema in scars, and hypertrophic scarring and to blend and fade postsurgical scars. Surgeons have taken little advantage of these capabilities, in part because of poor dissemination of information about the techniques and in part because of economic barriers to including these services simply as an added value in the postoperative care. Still other devices are capable of promoting expression of proangiogenic growth factors, which may accelerate healing or help treat an impending or actual flap loss complication. 1
5.4 Types of Energy Sources
Various forms of energy are delivered by devices performing the types of treatments indicated above. Light energy and radiofrequency (RF) energy are the most common, alone or in combination, but ultrasound and other sources are used as well. Light-based devices are divided into those delivering light at a single wavelength and those delivering a range of wavelengths, such as intense pulsed light (IPL) sources. Although the mechanics and optics of the devices are beyond the scope of this chapter, an understanding of the energy parameters emitted by the devices and the effects they have on treated tissue is essential to applying these devices productively and safely.
5.5 Energy–Tissue Interaction
Again, a detailed discussion is beyond the scope of this chapter, but several concepts are essential to understanding the tissue effects that are created by each individual device. Energy-based devices, particularly lasers, produce unmatched precision in the tissue effects created. This does not necessarily translate into a superior result clinically, but a detailed understanding of tissue effects allows the user to take advantage of this precision rather than discard it by improper parameter selection. The user interface on some modern devices is configured so as to put user input settings in terms of type and depth of tissue effect rather than energy parameters, providing a more clinically intuitive way of setting up each treatment. Basically, parameter selection and device configuration allow selection of the size, shape, depth, and spacing of the tissue effect created as well as the peak temperature produced. A separate category of devices does not work by using a photothermal mechanism but rather uses low levels of light to activate various biological pathways. The mechanism of such inductive treatments, as well as the clinical significance, is currently a matter of debate. The following discussion focuses on light–tissue interaction because it relates to the largest number of available devices, but similar issues relate to other forms of energy, although the specific rules work differently.
Each wavelength of light will be absorbed to a greater or lesser degree by each type of body tissue. Pigments or absorbing chromophores in the tissue define the degree of absorption. Modern light-based therapies work by selecting wavelengths with high absorption coefficients for a specific chromophore, such as water, hemoglobin, or melanin, found in high concentrations in the target tissue or structure. When the tissue is exposed to high-intensity light, the energy is preferentially absorbed by the target chromophore and converted to heat, which can coagulate or vaporize the target tissue or structure (e.g., telangiectatic blood vessel or solar lentigo), depending on the amount of energy input, the time course of the laser pulse, and the degree of absorption. Modern energy treatments are delivered in short pulses, which confine the heating to the exposed area with a minimum of collateral thermal damage (damage to nontarget tissue). This exposed area will be defined by the depth to which the light penetrates and the spot size of the light exposure. The depth will be defined by the wavelength and amount of absorbing chromophore and depth of absorbing chromophore in the skin. For example, melanin absorbs certain wavelengths of laser light very heavily and is located at the surface of the skin in the epidermis with little melanin content in normal dermis. Epidermis and dermis contain little hemoglobin, but underlying blood vessels will absorb more heavily a wavelength of light located at a hemoglobin absorption peak. How deep into an absorbing medium the light penetrates depends on the concentration of the chromophore, with more chromophore producing less penetration. To produce a confined thermal effect, the pulse of light must be shorter than the time it takes to conduct heat to the surrounding tissues, and the time between pulses must be quite a bit longer. Otherwise, thermal buildup will occur.
In laser resurfacing treatments of skin, for example, a pulse of laser light is absorbed by intracellular and extracellular water in the skin, which is heated to the point of vaporization. A crater of a certain size will be formed where water has boiled, creating steam and ejecting a small layer of surface tissue as laser plume. Surrounding this crater will be a zone of necrotic tissue, or what could also be called irreversibly thermally altered tissue. This dead tissue will slough or must otherwise be removed from the body. Surrounding the necrotic zone will be a zone of somewhat coagulated tissue or what could also be called reversibly thermally altered tissue. Here, cells may have some damage but are not dead. Some proteins and extracellular matrix components may be thermally altered (denatured). Tissue remodeling will take place in this zone, and various cytokines will be released. This remodeling effect probably takes place over several months. 2 Both the tissue removal and the tissue remodeling effects are essential components of maximizing the clinical results (Fig. 5.1). Current and emerging hardware and software configurations in the devices allow tremendous parameter flexibility in mixing and matching the amount of coagulation injury and tissue removal that is obtained both within a given crater and across the treatment area on the face.

5.6 Energy Strategies
This chapter is organized around the clinical strategies or tissue effects used to treat various conditions in the aging face. This organization allows grouping of similar technologies for comparison and global discussion of management. It also focuses attention on tissue effects and biological response, which will make it easier to understand future additions to the technology marketplace and where they fit.
5.6.1 Photocoagulation for Tissue Vaporization: Skin Resurfacing
These technologies include resurfacing, treatments that remove either epidermis or epidermis along with a variable depth of dermis, which may be removed at time zero through energy exposures that reach the point of vaporization with a variable mix of coagulation underlying from almost none to substantial. Such exposures leave an open wound with recovery time. Even if the energy exposures are not confluent, a substantially confluent effect is obtained in the clinically targeted area of skin, which is called a full-field treatment. Coagulated tissue at the base of the wound is sloughed, and the epidermis is reconstituted with cells that propagate from adnexal structures such as sweat glands and hair follicles in the zone of treatment. Alternative treatments use a fractional approach, which creates small columns of either coagulated or vaporized tissue with large skip areas in between. In a typical treatment, 15 to 40% (although with caution and specially selected parameters, up to 70%) of the total surface area in the treated zone may be exposed in these so-called fractional or fractionated treatments. The diameter of such exposures is typically 100 to 150 µm, although certainly less than 250 µm with substantial spacing between exposures. These are wounds whose biological behavior is different from that of full-field wounds. The depth of the exposure is typically at least 300 µm and can be up to 1.5 to 3 mm. A full-field wound this deep would heal with a scar routinely, but the safety profile of these fractional exposures is substantially better than that of full-field treatments. The source of healing cells is the large reservoir of unexposed tissue surrounding each exposed zone. Even coagulative injuries (nonablative fractional resurfacing) created using this technique produce a resurfacing effect with a replacement of the full thickness of epidermis and removal of coagulated dermis, which is translocated through the epidermis and ejected as debris. This effects resurfacing in much the same way as ablative resurfacing, just over the 2- to 3-week healing interval after a fractional nonablative treatment rather than at time zero. Such nonablative treatments are typically done as a series of treatments rather than as a single exposure. Despite the lack of an open wound, the patient may have substantial edema and erythema for a few days after the treatment, which will necessitate some downtime.
Indications
Skin resurfacing is indicated primarily for the treatment of rhytids. Antecedent alternatives include chemical peels and dermabrasion. We strongly consider laser resurfacing to be the gold standard against which all other resurfacing treatments must be measured. The precision and reproducibility of the tissue effects give these treatments a level of predictability that is unparalleled by any other option, particularly in terms of complication rates. The learning curve to become proficient at these treatments is also significantly less steep than that for the alternatives. Mastery of resurfacing technologies, allowing the surgeon to offer a range of treatments depending on rhytid severity, is essential to anyone who is serious about facial rejuvenation. Although a slight improvement in rhytids in the cheeks is obtained by redraping and lifting skin in facialplasty, minimal, if any, improvement is obtained in the perioral and periorbital area in terms of rhytids with a facialplasty procedure. Resurfacing by some means is the necessary option to address these areas meaningfully.
Improvements in skin texture and clearance of surface pigment such as solar lentigines are a secondary benefit. Nonablative fractional resurfacing also reduces redness, including rosacea and postsurgical redness in scars. Ablative and nonablative fractional resurfacing is indicated for blending of surgical scars and treatment of hypertrophic scars. Although a small degree of skin laxity reduction is obtained with aggressive skin resurfacing, we think this is never an indication for the treatment. If nonsurgical laxity reduction is desired, a device specifically cleared for this indication should be used.
Technologies
All resurfacing lasers use intracellular and extracellular water as the absorbing chromophore. Full-field devices remove 100% of the area being treated, whereas fractional devices create an array of injury and treat a portion of the skin surface, as previously mentioned. The principal ablative resurfacing modalities are Er:YAG (2,940 nm) and carbon dioxide (CO2; 10,600 nm). There are significant differences in the water absorption of each of these wavelengths. The lower water absorption of CO2 produces a significant mix of ablation and coagulation. Er:YAG technologies can be largely ablative (Fig. 5.2 a) or can use a mix of ablative pulses followed by subablative pulses in a pulse train to add in variable degrees of coagulation. A third wavelength, 2,790 nm, produced by the Er:yttrium scandium gallium garnet (YSGG), has an intermediate water absorption between that of CO2 and Er:YAG. There has been considerable debate in the past concerning whether CO2 lasers are more efficacious than Er:YAG lasers or vice versa. Depth of injury appears to be a more important predictor of efficacy and healing times than wavelength, but the safety profile of erbium full-field devices has made full-field CO2 resurfacing almost obsolete.

Fractional laser resurfacing originated with nonablative wavelengths in a variety of wavelengths (1,550 nm, 1,440 nm, 1,470 nm, and so forth) (Fig. 5.2 b) but quickly added ablative fractional resurfacing options with CO2, Er:YAG (Fig. 5.2 c), and Er:YSGG devices. A new device has recently been introduced offering a hybrid approach of both ablative and nonablative treatment in the same target spot, giving the potential advantage of greater efficacy with little, if any, modulation in downtime.
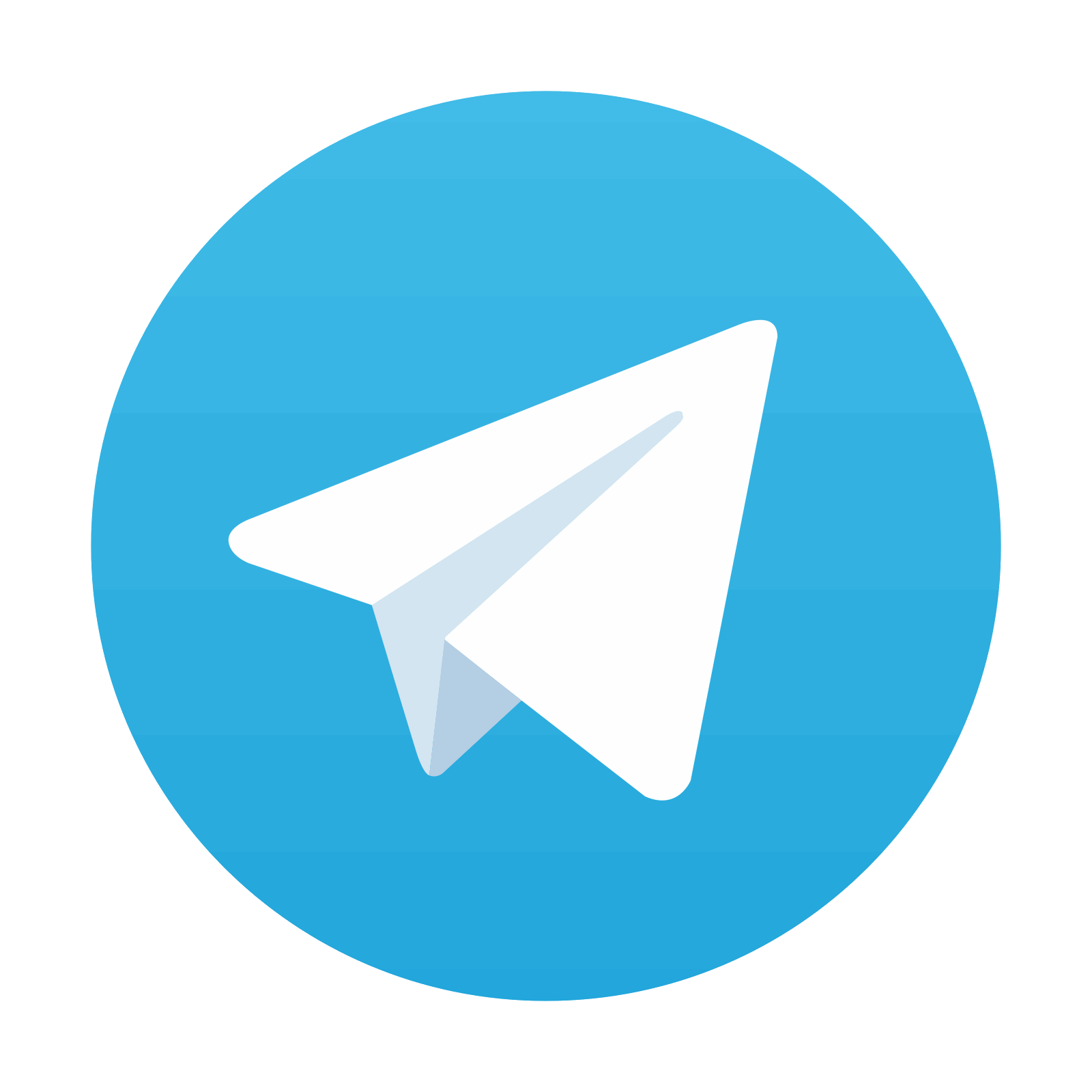
Stay updated, free articles. Join our Telegram channel

Full access? Get Clinical Tree
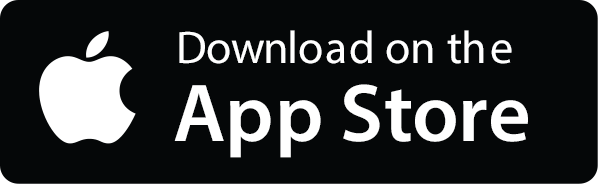
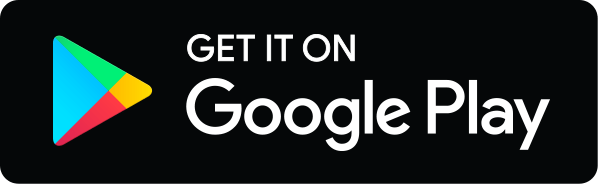
