2 Wound Healing
Introduction
Cutaneous wound repair involves a complex yet coordinated series of biological events. The facial plastic surgeon must understand the physiology and stages of wound healing to achieve optimal cosmetic and reconstructive outcomes. Virtually all full thickness skin incisions will result in visible scars; even when the healing process is normal, functionally disabling or disfiguring scars may occur. Abnormal wound healing may involve hypertrophic scarring, keloid formation, or contracture which further compromise appearance and function. Accordingly, the surgeon must approach an acquired or iatrogenic wound carefully, considering the factors involved in wound repair. This chapter reviews cutaneous anatomy, phases of wound healing, and skin substitutes and discusses factors affecting wound repair and scar formation.
History to Present Day
Wound healing has been a concern throughout recorded history. The Edwin Smith Papyrus (1600 BC) represents the first documentation of wound care and healing. 1 War and the treatment of its casualties account for most of the information recorded in ancient literature. Nearly 150 descriptions of wounds are included in Homer’s account on the siege of Troy by the Greeks (The Iliad, 1000 BC). The use of so-called sovereign medicines was noted in the treatment of these wounds, along with a high mortality rate. By the time of Hippocrates (460 to 370 BC), established wound management included removal of foreign bodies, cleansing and drying of the wound, suturing (with crude techniques), and application of clean, dry dressings. Celsus (25 BC to 50 AD) appears to be the first to discuss inflammation, but Galen (130 to 200 AD), in his treatment of Roman gladiators and soldiers, developed the doctrine of laudable pus (suppuration) as an important aspect of wound healing. Unfortunately, this notion was generally accepted until the 14th century when Henry de Mondeville (1260 to 1320) advised that wounds be cleaned and suppuration avoided. His doctrines were considered unacceptable by many but eventually prevailed.
With the advent of gunpowder in the 14th century, a new type of wound was established. Cauterization with hot oil was the treatment of choice, with little reverence for cleanliness. Only when French surgeon Pare (1520 to 1590) found his oil supply exhausted and was forced to apply milder compounds (e.g., egg yolk, oil of roses, and turpentine) did this inhumane, ineffective treatment end. 2 Wound treatment has continued to evolve since the 16th century, emphasizing optimal conditions for wound repair and, more recently, the use of skin substitutes. Advances include debridement of necrotic tissues and foreign bodies, wound edge apposition, and use of aseptic technique (the Lister principle). Of comparable validity are the following tenets of Halsted that should be known and respected: gentle handling of tissue, aseptic technique, sharp anatomical dissection of tissue, careful hemostasis, obliteration of dead space, avoidance of tension, and reliance on rest. 3
Historical precepts of debridement and optimal surgical technique remain indispensable, and these are now supplemented by a variety of new tools for the poorly healing wound. In the 1970s, wound dressings were designed almost exclusively to keep the wound moist to enhance epithelialization. This approach remains relevant today and has been expanded upon with the advent of bioengineering and nanotechnology advances. Innovative strategies aim at inducing growth by recapitulating the regenerative milieu encountered in an acute wound and/or restoring the microenvironment a healthy wound bed. The approval of growth factor products by the U.S. Food and Drug Administration (FDA) heralded a new era for approaches that “jump-start” healing of surgical wounds. 4 Over the past decade, there has been rapid proliferation of biological and clinical tools to promote cellular replication and healing.
Anatomical Considerations
Knowledge of the basic anatomical components of skin is essential to understanding wound healing. 5 The epidermis and the dermis play complementary roles in wound healing. The epidermis provides a protective, waterproof layer characterized by color, texture, and, unfortunately, scar tissue. The dermis provides most of the thickness and toughness of the skin. The basal, or germinal, layer continually replicates, pushing dying keratin to the surface. Destruction of this superficial layer by an abrasion or burn allows tissue fluid weeping and exposes the wound to outside substances, such as bacteria or medications. Because the basal layers remain intact in such injuries, healing generally occurs without scarring. However, given that melanocytes are found in the epidermis, these injuries may result in discoloration.
Because the epidermis contains no collagen fibers, the wound strength of a newly reepithelialized wound is minimal. The epidermis does, however, have considerable growth potential, enabling rapid epithelial cell migration to seal fresh wounds within a few hours. The epidermis rests on the dermis in an irregular papillary surface interface (papillary ridges) that makes the two parts difficult to separate. Collagen, the most important component of this layer, is produced as a fibrous protein. Through extensive cross-linking, it provides intrinsic strength to this layer. The process of laying down the collagen requires much more time than the rapid process of epithelial closure.
The dermis also contains the blood supply to the skin and various epithelial-lined skin appendages, such as hair follicles and sebaceous glands (pilosebaceous units). These units found in hairbearing skin play a critical role in the regenerative capacity of skin after injury, and they have rich vascularity and innervation ( Fig. 2.1 ). 6 Molecular studies have provided important insights into the pivotal role of stem cells in skin regeneration and healing after damage. 7 Blimp1, a transcriptional repressor found in progenitor cells in the sebaceous gland, has been shown to influence cellular fate. Its loss leads to elevated expression of the oncogene c-myc, increased cell proliferation, and hyperplasia of the sebaceous gland. 8 The Lgr6 protein was recently identified as a key marker of sebaceous gland–associated skin progenitors that can differentiate down several skin cells lineages during wound repair. 9 These discoveries highlight the vital role of pilosebaceous units in healing and help explain the impressive resilience of hairbearing versus non–hairbearing skin after laser resurfacing.

Wound Healing Phases
Wound healing is accomplished in multiple phases that occur over time ( Fig. 2.2 ). Whether an injury is secondary to an abrasion, a burn, a contusion, or a laceration, an immediate vascular and inflammatory response occurs. Local vasoconstriction is the initial event and lasts 5 to 10 minutes.

Inflammatory Phase
With endothelial cell injury, the coagulation cascade is activated, resulting in platelet adhesion and aggregation into clots or plugs. Activated platelets release a variety of biologically active substances, including prostaglandins and vasoactive materials (i.e., serotonin, histamine, proteases, and thromboxane) that further affect vascular tone. Various chemotactic and proliferative factors are also released, including platelet-derived growth factor (PDGF), vascular endothelial growth factor (VEGF), epidermal growth factor (EGF), insulin-like growth factor (IGF-1), transforming growth factor–β (TGF–β), activin, and fibroblast growth factor (FGF-2), which activate their target epithelial, endothelial, and fibroblast cells. 5 , 10 , 11 , 12 , 13 Table 2.1 lists several of the key cytokines involved in wound healing.
After the initial vasoconstriction, active vasodilation takes place, partly secondary to histamine release from mast cells, and circulates serotonin. These factors, along with proteolytic enzymes that activate kallikrein, significantly increase vascular permeability by allowing endothelial cell separation. Microvascular permeability changes increase for the first 48 to 72 hours, allowing the inflammatory phase to persist. 5 The cellular response in the inflammatory phase lags slightly behind the vascular changes. Fibronectin appears to be a major component of granulation tissue because it promotes the migration of neutrophils, monocytes, fibroblasts, and endothelial cells into the region. Cross-linking between the clot and fibronectin provides a temporary matrix by which epithelial cells and fibroblasts may proliferate in the wound. 13 , 14 Polymorphonuclear leukocytes (granulocytes) and monocytes appear shortly after injury.
Granulocytes are stimulated by chemotactic factors and act primarily to remove bacteria and debris from the wound. These shortlived cells disappear rapidly in the uncontaminated wound. In the contaminated wound, however, granulocytes persist and prolong the inflammatory phase. This extended inflammation may account for more severe endstage scarring. Accordingly, it is important to progress from this stage as quickly as possible so that the wound may close and subsequent collagen deposition may begin. 5 In contrast, healing will proceed in the clean wound without the presence of granulocytes if there is normal macrophage, fibroblast, and endothelial cell function. 15 Perhaps the most important component of the cellular response of the inflammatory phase is the macrophage. 16 This becomes the predominant cell type by day 3 or 4. 5 Phagocytosis and tissue debridement are an important function of macrophages; but, release of chemotactic and growth factors, including TGF–β, basic FGF, EGF, TGF–α, and PDGF, for endothelial cell and fibroblast proliferation may be the most significant functions. 17 , 18 , 19 In the context of poor macrophage function, granulation tissue formation, fibroplasia, and collagen production are impaired, as well as overall wound healing. 20
Lymphocytes produce TGF–β, interferons, interleukins, and tumor necrosis factor, which also interact with the macrophage during the inflammatory process, linking the immune response to wound repair. 21 The transition from inflammation to subsequent conditions illustrates the complexity and overlap of the concept of successive stages in wound healing. Interaction of the vascular and cellular responses prepares the wound for formation of granulation tissue, epithelial repair, and collagen deposition. In the first few days after injury, wound strength is minimal and relies primarily on fibrin clot and early epithelialization. 3 , 5 Only when inflammation subsides does collagen deposition begin, with subsequent increases in tensile wound strength.
Proliferative Phase
The next major aspect of wound healing is the proliferative phase, which includes epithelial regeneration, fibroplasia and collagen formation, wound contraction, and neovascularization. Epithelial regeneration plays a critical role in the wound repair process. With the completion of this process there is reestablishment of a barrier to protect underlying tissues from bacteria and foreign material. The process of reepithelialization begins within 24 hours of injury involving migration of epithelial cells from surrounding wound margins or from deeper adnexal structures, such as hair follicles or sebaceous glands. Basal epithelial cells undergo mitosis, differentiate, and separate from the underlying basement membrane and dermis to migrate across the wound. 5 , 17 , 22 , 23 Careful attention to wound care, including removal of excessive scab tissue and avoidance of wound desiccation, are important measures to minimize the risk of depressed scar formation ( Fig. 2.3 ).

The advancing front of epithelial cells continues until it comes into contact with similar epithelial cells. Contact inhibition of migration occurs, followed by further mitotic differentiation leading to epithelial stratification. Simultaneously, basal cells next to the wound and in the injured skin appendages begin mitotic activity, thus assisting in thickening the new epithelial layer. 22 This extended epithelial growth is maximal at 48 to 72 hours and is promoted by EGF and TGF–α. These polypeptides are mitogens for epithelium and stimulants for fibroplasia and granulation tissue. 17 , 24 , 25 EGF enhances early wound healing, particularly in partial-thickness wounds. 26 Chalones, glycoproteins that inhibit epidermal replication, are temporarily absent in an open wound, and their absence appears permissive of epidermal proliferation. 27 In wounds closed primarily, epithelialization may be completed in 24 to 28 hours. Healing wounds by secondary intention take far longer, especially if the wound is full thickness or on a poorly vascularized bed. In these cases, minimal epithelial migration usually continues for 3 to 5 days until a granulation bed has been established. 5
It has long been understood that a moist wound surface enhances normal wound healing and that wound desiccation significantly impairs healing. 28 When a crust or scab forms, the underlying dermis becomes desiccated and partially necrotic. Accordingly, the migrating epidermis takes a longer, less efficient route, delaying epithelialization. In occluded moist wounds, migration is direct and unimpeded, providing more rapid wound coverage. 13 , 29 As reepithelialization proceeds, granulation tissue forms beginning 3 to 4 days after injury. It consists of inflammatory cells, new blood vessels, and fibroblasts in a bed containing fibrin, glycoproteins, newly formed collagen, and glycosaminoglycans. 13 Granulation tissue persists until reepithelialization is completed. Fibroblasts appear in the wound as early as day 2 or 3 and play an important role in healing.
Fibroblasts are critical in several key wound healing processes, including breaking down fibrin clot, producing extra cellular matrix and collagen, and supporting wound contraction. 30 Fibroblasts migrate and replicate in response to mediators (C5a, fibronectin, PDGF, FGF-2, and TGF–β). 4 Fibroblasts are also important in the production of elastin, fibronectin, glycosaminoglycans, and collagenase, which is important in the later maturation and remodeling phase. 31 Fibroblasts, along with perivascular mesenchymal cells, differentiate into myofibroblasts, which allow for wound contraction. 32 Glycosaminoglycans are complex polysaccharides that are important to the early granulation tissue response. Hyaluronic acid initially predominates but is replaced by chondroitin 4-sulfate, dermatan sulfate, and other proteoglycans. 13 Together these make up the ground substance that is important for subsequent collagen formation. 22 Although collagen is present in the wound as early as day 3, the rate of synthesis increases dramatically by day 4. It is commonly believed that the fibroblastic aspect of the proliferative phase begins at this time. 33 Collagen molecules, or tropocollagen, are aggregated into filaments, which in turn are woven into fibrils via complex intermolecular bonding. These fibrils combine in a ropelike fashion to form collagen fibers. 22
In the early period of fibroplasia, type III collagen predominates. As the scar matures, type I collagen becomes the major component of scar tissue. 17 Wound tensile strength is minimal in the first few days, and there is a gradual increase in strength corresponding to the amount of collagen in the wound. At the end of the inflammatory phase, ∼5 to 7 days, the wound has only ∼10% of its final tensile strength. Collagen synthesis and degradation reach a maximum ∼3 weeks after injury. 5 A period of maturation follows, during which collagen formation subsides and fibroblasts decrease in the scar.
Contraction is a key feature of the proliferative phase, since wounds must be closed before other longterm healing processes take place. This contraction is centripetal, mediated by the myofibroblast, and maximal at 10 to 15 days. If a wound is left open for a prolonged period, contraction may become severe, especially if significant inflammation is present. 5 In the presence of skin grafts, wound contraction is less marked than with splitthickness grafts or open wounds, but contraction is still a concern—particularly near mobile structures such as the eyelid or lip. Even with fullthickness skin grafts, 20% contraction generally occurs. 34
Neovascularization is another important event for the healing wound. Two forms of neovascularization occur: angiogenesis (the formation and differentiation of blood vessels from local endothelial sprouting) and vasculogenesis (de novo development of blood vessels driven by bone marrow–derived circulating endothelial progenitors). 35 Endothelial cell migration through the basement membrane is followed by angiogenesis, which is stimulated by macrophages, platelets, lymphocytes, and mast cells. These cells release numerous growth factors, including FGF, PDGF, TGF-α and β, and vascular endothelial growth factor. Vasculogenesis reflects a more systemic response to injury. Low oxygen tension in the wound may stimulate angiogenic factors from macrophages, leading to the formation of new vascular channels. 36 As oxygenated blood reaches the wound, this factor decreases stimulation of neovascularization, leading to an eventual decrease in vascularity of the scar. 17
Maturation or Remodeling Phase
The final stage of wound healing is the maturation or remodeling phase. During this period, the scar becomes stronger and shows a decrease in its dimensions and erythema. The histological appearance of normal skin versus scar is shown in Fig. 2.4 . Skin becomes pale in color, softer, and less protruding. Post inflammatory hyperpigmentation begins to regress as remodeling and reorganization get under way. Type III collagen is gradually replaced by type I collagen. Water resorption around the scar allows further organization of the collagen fibers. 13 Although these fibers are initially in disarray, they gradually line up in a more parallel fashion when a wound heals normally, increasing tensile strength and improving appearance. Likewise, neovasculature regresses and the final scar is relatively avascular. Completion of the remodeling phase may take 12 to 18 months. At this time, the scar has ∼70 to 80% of the tensile strength of unwounded normal skin.

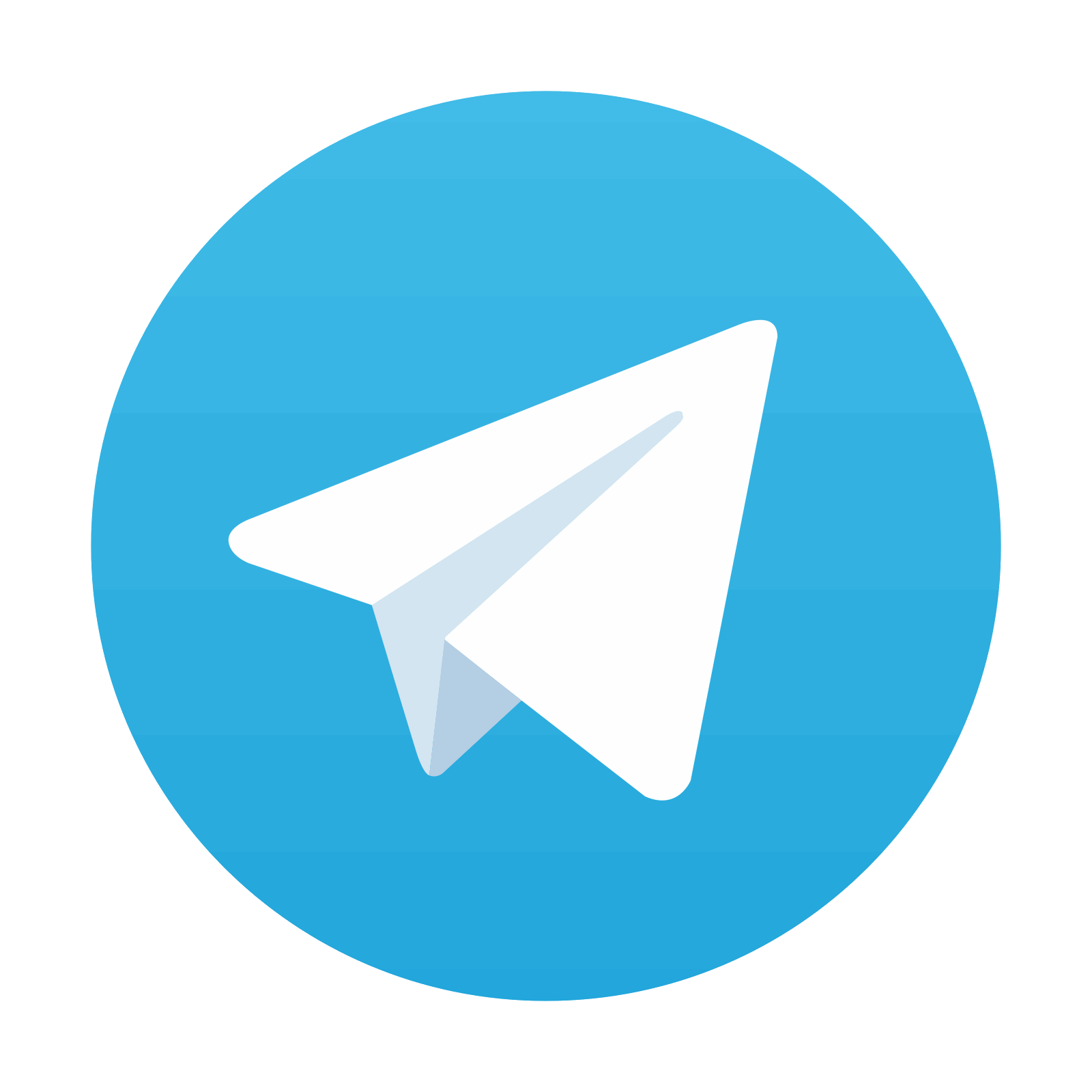
Stay updated, free articles. Join our Telegram channel

Full access? Get Clinical Tree
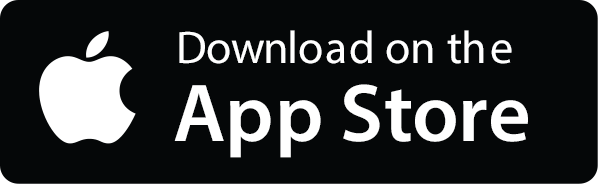
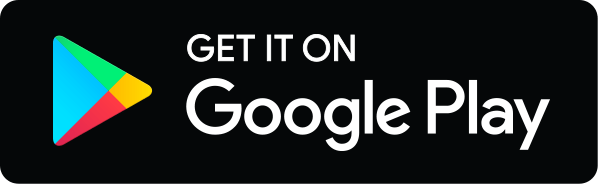
