For the laser surgeon, the Er-YAG laser is an invaluable tool that delivers unsurpassed ablation efficiency, and with appropriate functionality (quasi long-pulse feature) provides sufficient tissue coagulation to remodel deep rhytids. As such, the 2940-nm wavelength is well suited for routine laser skin rejuvenation in full-field, fractional, and point-beam modes with additional benefits, including applicability to diverse skin types, short healing times, and a low likelihood of energy-related complications.
Despite alternative methods for skin rejuvenation, including nonablative fractional laser therapy, photorejuvenation, photodynamic therapy, medium and deep chemical peels, ablative fractional laser therapy, and other treatments, full-field (complete surface coverage) ablative laser therapy remains popular for patients seeking maximum improvement with a single treatment. Ablative laser skin resurfacing (LSR) wavelengths include 2790 nm (erbium:yttrium-scandium-gallium-garnet or Er-YSGG), 2940 nm (erbium:yttrium-aluminum-garnet or Er-YAG), and 10,600 nm (carbon dioxide or CO 2 ). Of these, the Er-YAG laser wavelength is an excellent choice for improvement of a variety of skin conditions and features of aging, including dyschromia, actinic photodamage, solar elastosis, acne and traumatic scarring, fine lines and mild to moderate rhytidosis, coarse skin texture, and skin laxity.
Similar therapeutic benefits, including skin tightening, are well established with the carbon dioxide and Er-YSGG wavelengths ; however, overall benefit, including rhytid effacement, skin tightening, and long-term improvement has long been considered by many physicians to be superior with the CO 2 wavelength. Even so, traditional multipass ablative full-field CO 2 LSR has declined in popularity owing to a number of factors, including higher risk of postresurfacing sequelae and complications, extended posttreatment recovery time, and increasing popularity and effectiveness of the alternative skin-resurfacing modalities enumerated previously. And when directly comparing full-field resurfacing lasers, it is the author’s opinion that the Er-YAG laser is an indispensible tool with an acceptably low risk-to-benefit ratio that, with appropriate functionality, enables physicians to effectively treat aging skin and other conditions in patients with a greater diversity of skin types.
The first Er-YAG laser was introduced commercially in 1997. In practice, the first Er-YAG skin-resurfacing lasers proved to be effective for soft tissue ablation but not for soft tissue coagulation. The initial excitement about the 2940-nm alternative wavelength diminished with the realization that the first-generation devices required multiple passes to ablate into the upper dermis and that on reaching this depth, significant bleeding caused difficulty with completion of treatments and also reduced the ablation efficiency of the device at that point during treatment. Second-generation Er-YAG lasers (circa 1999) avoided unchecked bleeding by adding a long-pulse or variable-pulse feature designed to provide significant soft tissue coagulation ability.
The flexibility to deliver Er-YAG laser energy for “pure” ablation or “pure” coagulation as well as a user-determined blend of ablation and coagulation to precise tissue depths led to recognition of these devices (those with long-pulse or variable-pulse modes) as extremely versatile. Full-field ablative Er-YAG LSR options that remain relevant more than a decade later (even with the interim development of many other useful treatment modalities) include superficial (partial epidermal), moderate depth (ablation into upper dermis), and deep skin peels (mid-papillary dermis or deeper) and combinations with other treatment modalities (eg, superficial peel and photorejuvenation).
The benefits of nonablative fractional laser treatment (including wavelengths of 1410, 1450, 1540, 1550, and more recently 1927 nm) profoundly influenced the approach to laser skin rejuvenation and also led to use of this novel type of energy delivery with the ablative laser wavelengths. Reduction of initial posttreatment recovery time (compared with traditional full-field LSR; dramatic for nonablative fractional resurfacing [NFR] and modest for ablative fractional resurfacing [AFR]), extension of these treatment modalities to additional skin types and conditions, widespread patient acceptance, and significant demand has legitimized this alternative approach.
Nonetheless, an area of current interest is the middle ground between pure AFR and pure full-field LSR, where the potential for very significant improvement, while preserving at least some of the benefits of AFR and of LSR, exists by combining these very different approaches to delivery of laser energy to the skin. The advantages of dual-wavelength (CO 2 and Er-YAG) LSR have been explored in detail in the past. By partial extension, a novel approach currently in use by the author and others involves concurrent dual-wavelength, dual-modality laser skin rejuvenation wherein CO 2 AFR deep dermal ablation immediately precedes or immediately follows full-field Er-YAG LSR.
Er-YAG laser tissue interaction
The Er-YAG laser wavelength has the highest absorption coefficient for water among the ablative laser wavelengths ( Fig. 1 ) with approximately 12 times and 5 times greater absorption in water versus CO 2 and Er-YSGG, respectively. During LSR, water serves as the primary tissue target wherein light energy is transduced into heat energy that is then quickly absorbed by the surrounding tissues, including collagen. The characteristic tissue interactions that define each of the ablative resurfacing lasers are related to their relative absorption by water but also several other factors. The continuum of responses to heating of biologic tissues includes hyperthermia, coagulation, carbonization, and vaporization and each of these may be observed during LSR. In general terms, higher water absorption is correlated with greater tissue ablation (vaporization), whereas lower water absorption is correlated with greater tissue coagulation.
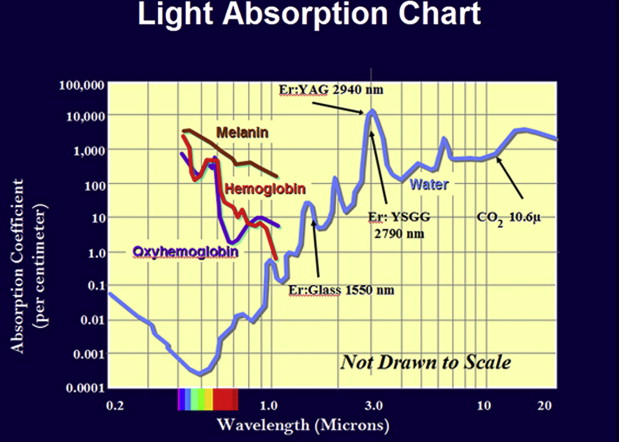
Distinct zones of tissue injury occur following cutaneous application of laser energy with ablative properties ( Fig. 2 ), including vaporization zone (superficial for full-field and central for microfractional techniques), irreversible injury zone (coagulation; intermediate for full-field and for microfractional techniques), and reversible injury zone (hyperthermia; deep for full-field and peripheral for microfractional techniques). Carbonization may also occur (most likely with CO 2 laser wavelength) if tissue heating continues beyond the coagulation stage and the tissue is not vaporized.
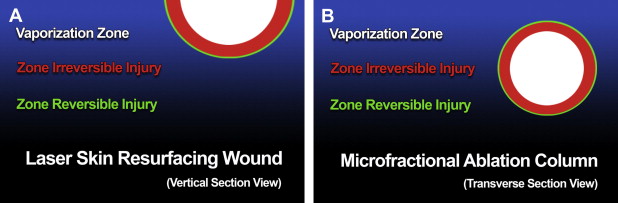
LSR tissue response is affected by wavelength (with tissue-specific absorption coefficient and optical penetration depth), tissue, and energy delivery factors. The optical penetration depth reflects that level in the tissue where laser irradiation has decreased by approximately 60% from its peak value incident at the tissue surface (or about 20 μm for the CO 2 and 1 μm for the ablative erbium lasers). Energy delivery factors include power (W or watts), spot size, pulse duration, radiant exposure (RE), and power density. RE (energy density or fluence) reflects energy delivered to a unit of tissue expressed in joules per centimeter squared (J/cm 2 ). Power density is determined by the ratio of fluence over exposure time. Increased fluence during short-pulse and long-pulse Er-YAG LSR increases depth of ablation and coagulation but correlation of device settings and histologic effect diminishes in long-pulse mode after the initial pass. Excessive fluence during Er-YAG LSR may be a major contributing factor for unfavorable outcomes with repeated passes using long-pulse mode.
Other tissue factors include thermal relaxation time and wavelength and tissue-specific ablation threshold. Thermal relaxation time refers to the time needed for 50% reduction of the achieved peak tissue temperature. Although skin thermal relaxation time of 1 millisecond is widely quoted in the literature, Ross and colleagues suggest that it may be significantly longer for the CO 2 laser (and by extension long-pulse Er-YAG laser). Ablation threshold, radiant energy required to vaporize tissue, is dependent on wavelength and tissue type. For human skin, the ablation threshold is much lower for the Er-YAG than for the CO 2 laser (1.6 vs 5.0 J/cm 2 ). In general, pulse durations shorter than thermal relaxation time and energy densities equal to or greater than ablation threshold limit thermal spread and related collateral tissue damage, whereas longer pulse durations and subablative laser fluences accomplish the opposite.
From a practical standpoint, the laser surgeon is able to modulate tissue response to desired effect by exerting control over energy density (reflecting selected parameters for power, pulse duration, and spot size) and energy delivery method. With short-pulse durations, the early Er-YAG skin resurfacing lasers were optimized (beyond the native properties inherent to this wavelength) for efficient tissue ablation but poor tissue coagulation. Increasing the fluence with these systems resulted in increased depth of tissue ablation but also resulted in significant bleeding upon reaching the microvasculature of the upper papillary dermis. The subsequent generation of Er-YAG skin-resurfacing lasers added a long or variable pulse feature with a low fluence to increase the coagulation effect and reduce intraprocedure bleeding. This “long” pulse effect was achieved by developing the ability to entrain or stack multiple subablative pulses close together that in aggregate resulted in a dramatic shift in tissue behavior with excellent coagulation. The ability to create nearly pure ablative or coagulative effects, as well as a user-defined (problem-specific) blend of these tissue effects with second-generation Er-YAG skin resurfacing lasers, brought this wavelength squarely to the forefront of skin rejuvenation technologies.
Er-YAG radiant laser energy may be delivered via a traditional scanning handpiece (eg, full-field skin resurfacing), fixed spot (eg, lesion ablation), or via fractional handpiece (also scanned). Fractional laser energy delivery was applied to the Er-YAG wavelength following introduction of AFR with the CO 2 wavelength and involves creation of multiple microscopic ablation columns at fixed distances apart and leaving normal, uninjured tissue in the intervening spaces. The same tissue effects (ablation, coagulation, ablation + coagulation) may be achieved; however, the orientation of effects is perpendicular to the target (vs planar with scanned full-field LSR) such that ablation is central, coagulation is paracentral, and reversible tissue injury is peripheral (see Fig. 2 ).
During Er-YAG AFR with the Sciton ProFractional XC handpiece (Sciton, Inc, Palo Alto, CA, USA), energy is delivered with a spot size of 430 μm with total surface area coverage of up to 11% with a single pass and with available treatment depth (up to 1500 μm) well beyond the typical depth achieved with traditional full-field ablative LSR. Increasing the pulse energy and/or stacking short pulses increases treatment depth in short-pulse mode, whereas use of long-pulse mode increases the width of the irreversible injury zone and enhances tissue remodeling. Figs. 3 and 4 highlight representative histology with variable treatment depth (see Fig. 3 , short-pulse Er-YAG AFR) and variable levels of irreversible thermal injury (see Fig. 4 , short-pulse and long-pulse Er-YAG AFR with fixed treatment depth).
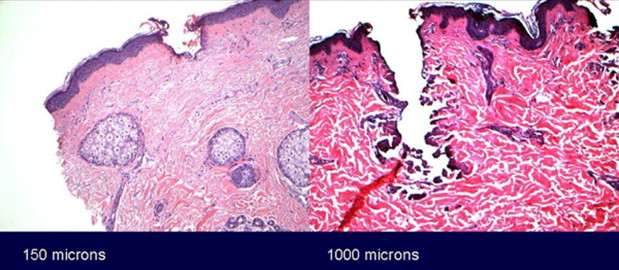
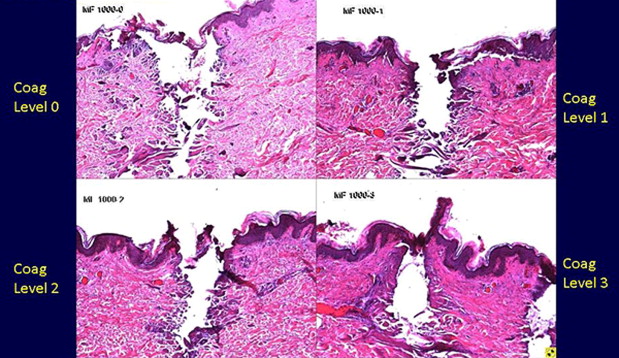
Indications—full-field LSR, spot treatment, and AFR
Contemporary indications for Er-YAG full-field ablative LSR include actinic photodamage, dyschromia, fine lines, mild to moderate rhytidosis, mild skin laxity, acne or traumatic scarring, and coarse skin texture. Er-YAG full-field ablative LSR indications extend primarily to Fitzpatrick skin types I and II; however, Fitzpatrick skin types III and IV may be successfully treated with the understanding that more conservative treatment parameters are generally indicated and that the potential for postinflammatory hyperpigmentation (PIH) following treatment is higher.
Spot treatment (eg, 2-mm spot) with the Er-YAG laser in pure ablate mode may be used for a variety of skin lesions with excellent outcomes among all skin types. Lesions amenable to removal using this approach include seborrheic keratoses, benign (including pigmented) moles, skin tags, small superficial intradermal cysts, and even solitary dilated pores or deep (eg, ice-pick) acne scars. Other conditions (eg, syringoma, xanthelasma) may require additional intervention, but ablation of the skin over these lesions using Er-YAG spot energy delivery is a very useful adjunct.
The general goal for Er-YAG spot treatment is to ablate just past the lesion(s) while sparing as much normal remaining tissue as possible to minimize the potential for scar formation. In contrast, treatment of a solitary dilated pore or deep acne scar involves essentially creating a circular ablation column through the entire thickness of the skin down to the subcutaneous fat. The superficial aspect of the transcutaneous ablation column may be closed with a 6-0 fast-absorbing gut horizontal mattress cutaneous suture or the wound may be left to heal by secondary intention. Secondary intention healing of the transcutaneous ablation column occurs rapidly with cicatricial wound contraction. The potential for dermal scarring may be further minimized by superficial closure, but healing and results have been very good with both methods.
Indications for Er-YAG AFR partially overlap those for ablative LSR. Single-session improvements with the Sciton ProFractional XC handpiece at maximum coverage (11%) are limited (even at maximum coverage with double pass, 22%), however, compared with full-field techniques with 100% coverage. AFR should not be used as a definitive therapeutic method for treatment of actinic keratoses. Similarly, patient expectations for resolution of dyschromias should be realistic for the partial skin surface coverage typical of a single AFR treatment. Improved single-session results may be obtained with preexisting diffuse dyschromia by combining AFR with a superficial laser skin peel (partial epidermal or transepidermal). Improved single-modality results with Er-YAG AFR alone may be obtained in a single session with multiple passes or via a series of treatments over multiple sessions. Er-YAG AFR may be performed for skin types I through VI. PIH following fractional laser skin rejuvenation may occur with ablative and nonablative wavelengths and has been correlated with skin type (more likely with darker skin) and increased density but not depth of microfractional ablation/coagulation columns/zones.
Indications—full-field LSR, spot treatment, and AFR
Contemporary indications for Er-YAG full-field ablative LSR include actinic photodamage, dyschromia, fine lines, mild to moderate rhytidosis, mild skin laxity, acne or traumatic scarring, and coarse skin texture. Er-YAG full-field ablative LSR indications extend primarily to Fitzpatrick skin types I and II; however, Fitzpatrick skin types III and IV may be successfully treated with the understanding that more conservative treatment parameters are generally indicated and that the potential for postinflammatory hyperpigmentation (PIH) following treatment is higher.
Spot treatment (eg, 2-mm spot) with the Er-YAG laser in pure ablate mode may be used for a variety of skin lesions with excellent outcomes among all skin types. Lesions amenable to removal using this approach include seborrheic keratoses, benign (including pigmented) moles, skin tags, small superficial intradermal cysts, and even solitary dilated pores or deep (eg, ice-pick) acne scars. Other conditions (eg, syringoma, xanthelasma) may require additional intervention, but ablation of the skin over these lesions using Er-YAG spot energy delivery is a very useful adjunct.
The general goal for Er-YAG spot treatment is to ablate just past the lesion(s) while sparing as much normal remaining tissue as possible to minimize the potential for scar formation. In contrast, treatment of a solitary dilated pore or deep acne scar involves essentially creating a circular ablation column through the entire thickness of the skin down to the subcutaneous fat. The superficial aspect of the transcutaneous ablation column may be closed with a 6-0 fast-absorbing gut horizontal mattress cutaneous suture or the wound may be left to heal by secondary intention. Secondary intention healing of the transcutaneous ablation column occurs rapidly with cicatricial wound contraction. The potential for dermal scarring may be further minimized by superficial closure, but healing and results have been very good with both methods.
Indications for Er-YAG AFR partially overlap those for ablative LSR. Single-session improvements with the Sciton ProFractional XC handpiece at maximum coverage (11%) are limited (even at maximum coverage with double pass, 22%), however, compared with full-field techniques with 100% coverage. AFR should not be used as a definitive therapeutic method for treatment of actinic keratoses. Similarly, patient expectations for resolution of dyschromias should be realistic for the partial skin surface coverage typical of a single AFR treatment. Improved single-session results may be obtained with preexisting diffuse dyschromia by combining AFR with a superficial laser skin peel (partial epidermal or transepidermal). Improved single-modality results with Er-YAG AFR alone may be obtained in a single session with multiple passes or via a series of treatments over multiple sessions. Er-YAG AFR may be performed for skin types I through VI. PIH following fractional laser skin rejuvenation may occur with ablative and nonablative wavelengths and has been correlated with skin type (more likely with darker skin) and increased density but not depth of microfractional ablation/coagulation columns/zones.
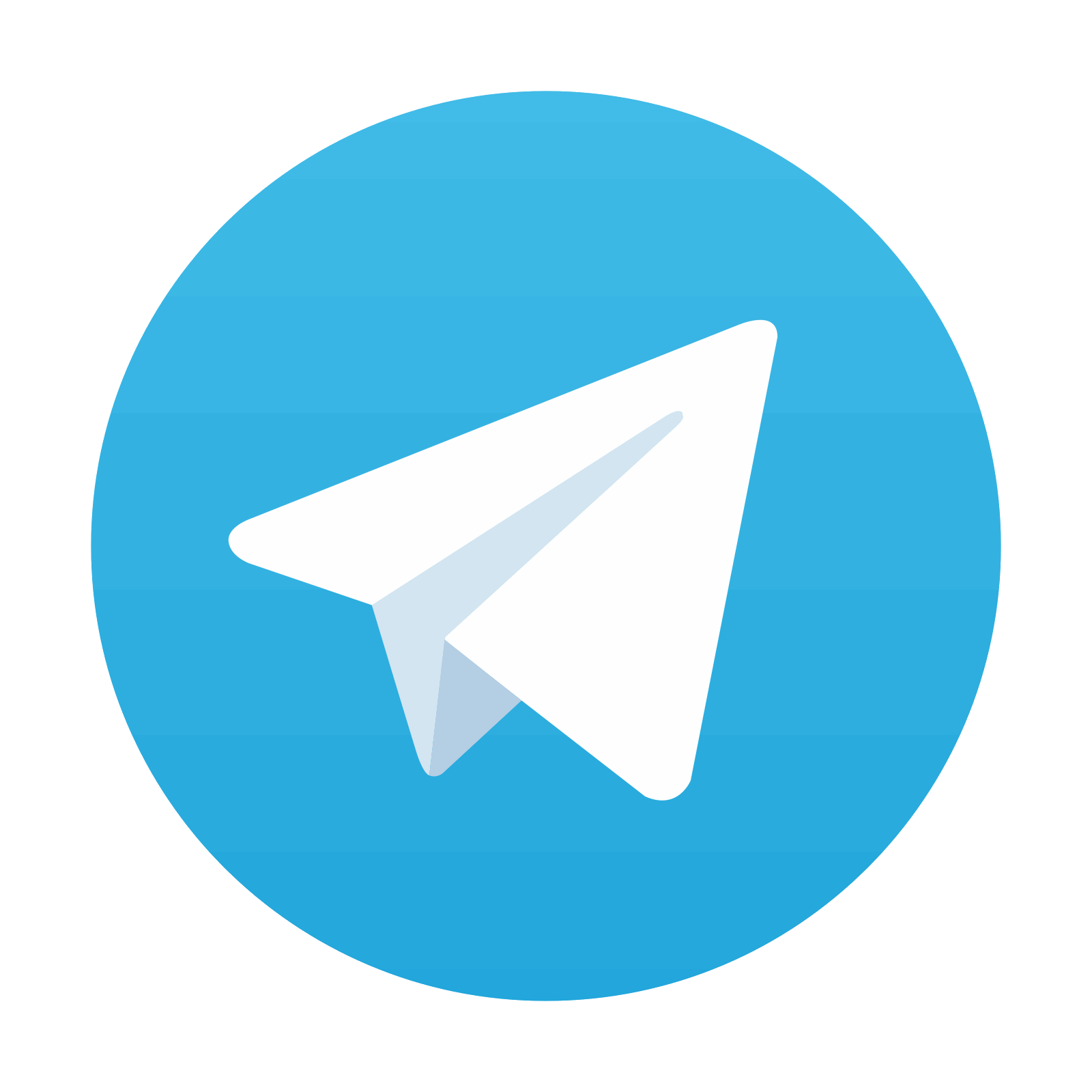
Stay updated, free articles. Join our Telegram channel

Full access? Get Clinical Tree
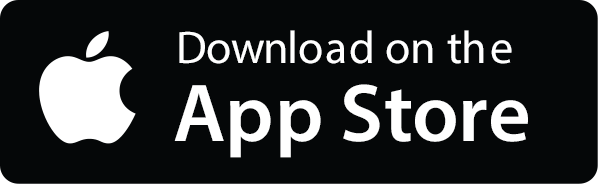
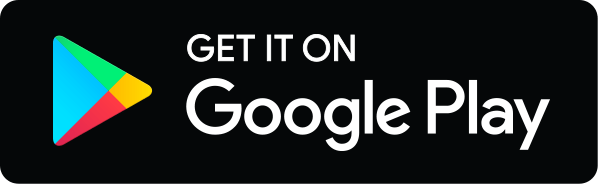