Fig. 30.1
Cross section of alveolar wall. Small double arrow is the thin side of the alveolar wall. Oxygen will travel from the alveolar space through the wall of the type I epithelium → interstitium (formed by the fused basal laminae of the epithelial and endothelial cells) → capillary endothelium → plasma in the alveolar capillary → cytoplasm of the red blood cell. Long double arrow represents the thick side of gas exchange where the space between the alveolar epithelium and the alveolar-capillary endothelium has been thickened by accumulation of elastin, collagen, and matrix materials
Understanding the structure of the bronchial tree and the lung and its gas exchange units will help to clarify the determinants of ventilation, blood flow, and gas exchange within the lung and how we measure them.
30.1.1 Spirometry, Lung Volumes, and Diffusing Capacity
Total lung capacity (TLC) represents the maximal volume within the lung after maximal inspiration (Fig. 30.2). The vital capacity (VC) is the volume of gas that can be forcibly exhaled by a person from total lung capacity. The volume that remains after the VC is exhaled is called the residual volume (RV). During normal breathing, the volume of air that leaves the lung is called the tidal volume (VT). The functional residual capacity (FRC) refers to the volume that remains in the lung during normal expiration, unlike the RV which is the volume that remains in the lung after maximal expiration. Figure 30.2 also indicates the inspiratory reserve volume (IRV) and the expiratory reserve volume (ERV). IRV is the maximum volume of air that can be inhaled after a normal tidal volume inhalation. ERV is the maximum volume of air that can be exhaled after a normal tidal volume exhalation.
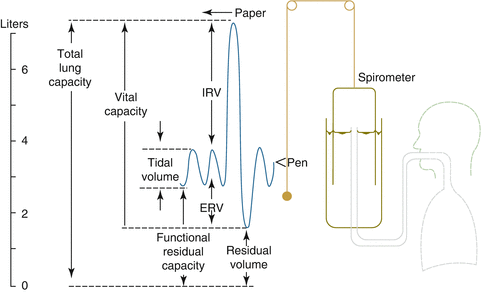
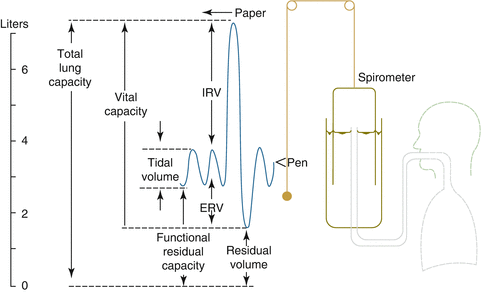
Fig. 30.2
Total lung capacity (TLC) represents the maximal volume within the lung after maximal inspiration (IRV inspiratory reserve volume, ERV expiratory reserve volume)
Spirometry is a safe and simple assessment of lung function. The inability to follow the technician’s instructions as a result of altered mental status, unwillingness, or physical limitations, i.e., small mouth opening or muscular dystrophies and active pain and nausea may all affect the quality and reproducibility of the measurements.
Spirometry can measure the forced vital capacity (FVC) (the maximal volume of air forcefully exhaled after a maximal inspiration) and forced expiratory volume in 1 s (FEV1) (the amount of air exhaled during the first second of a FVC maneuver). The FVC maneuver also generates two other values, the forced expiratory flow between 25 and 75 % of the FVC (FEF25 %–75 %) and peak expiratory flow (PEF), which is the maximum flow achieved during forced exhalation. Reductions in FEF25 %–75 % may represent small airway dysfunction, but this value is rarely used alone as it is highly variable and dependent on exhalation time which is not solely dependent on small airways. The PEF can also be measured with a handheld peak flow monitor often used at the bedside or at home with asthmatic patients and reflects the diameter of the large airways. PEF is effort and technique dependent and less reliable than FEV1 [1].
Spirometry is used to determine if there are reductions of the FVC, FEV1, and the FEV1/FVC ratio. The first step in the interpretation of pulmonary function tests is always the FEV1/FVC ratio; if this is reduced below the lower limit of normal, then one can make a diagnosis of airway obstruction. The severity of airflow obstruction can further be delineated by the reduction of FEV1 % predicted. Of note, the FEV1/FVC ratio decreases with age, where at the age of 20 years old, a normal value for the FEV1/FVC ratio is 85 %, by age 80 the mean value for the FEV1/FVC ratio is 73 %. The changes in airflow seen with aging are more pronounced at lower lung volumes and the decrement in the FEV1/FVC ratio is primarily due to a loss of elastic recoil in the lung.
In restrictive lung diseases, the FEV1/FVC ratio is preserved. The diagnosis is made by total lung capacity (TLC) below the fifth percentile of the predicted value. Spirometry cannot determine TLC, so the FVC is often used as a surrogate, and a normal FVC can eliminate restrictive ventilatory impairment as a diagnosis with 95 % accuracy, but a low FVC cannot reliably diagnose restriction. This is because the vital capacity can be reduced (despite a normal TLC) when inspiratory or expiratory efforts are submaximal, if there is air trapping associated with small airway closure or noncommunicating bullae within the lung parenchyma, thus elevating the RV [1].
Bronchodilator testing is a useful adjunct to spirometry to determine if a subject has significant airflow obstruction that can be reversed with bronchodilators. The test is performed by administering two puffs of a short-acting bronchodilator such as albuterol. A positive bronchodilator response is defined as an increase in FEV1 and/or FVC ≥12 % and 200 mL of the baseline value.
Spirometry can measure the maximum voluntary ventilation, which is the largest volume of gas that can be breathed voluntarily into or out of the lungs in 1 min. The MVV is used as a marker for respiratory muscle strength, pulmonary and thoracic compliance, airway resistance, and neural control mechanisms. In a young healthy male adult, the average MVV is 170 L/min and 110 L/min for young healthy women. MVV will decline with chronic obstructive pulmonary disease, advancing age, and neuromuscular weakness.
Spirometry can also be used to evaluate for an extrathoracic upper airway obstruction such as tracheomalacia; vocal cord abnormalities (paralysis, edema, neoplasms, and vocal cord dysfunction); thyroid tumors; laryngeal edema (angioedema, post-extubation, burns); epiglottitis, tonsillitis, and retropharyngeal abscesses; and foreign bodies. The flow volume loop, which is derived from spirometry, is more valuable than the other measurements for diagnosing upper airway obstruction, as the FEV1 and FVC may remain unchanged as the diameter of the airway exponentially narrows. The inspiratory loop will be flattened with extrathoracic upper airway obstruction, while the expiratory look will be flattened with intrathoracic upper airway obstruction (Fig. 30.3).
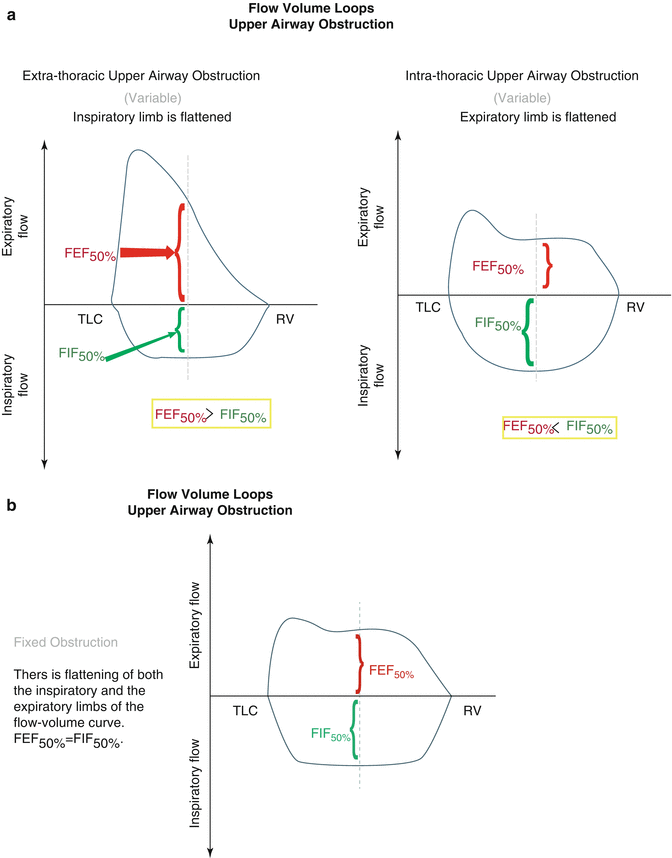
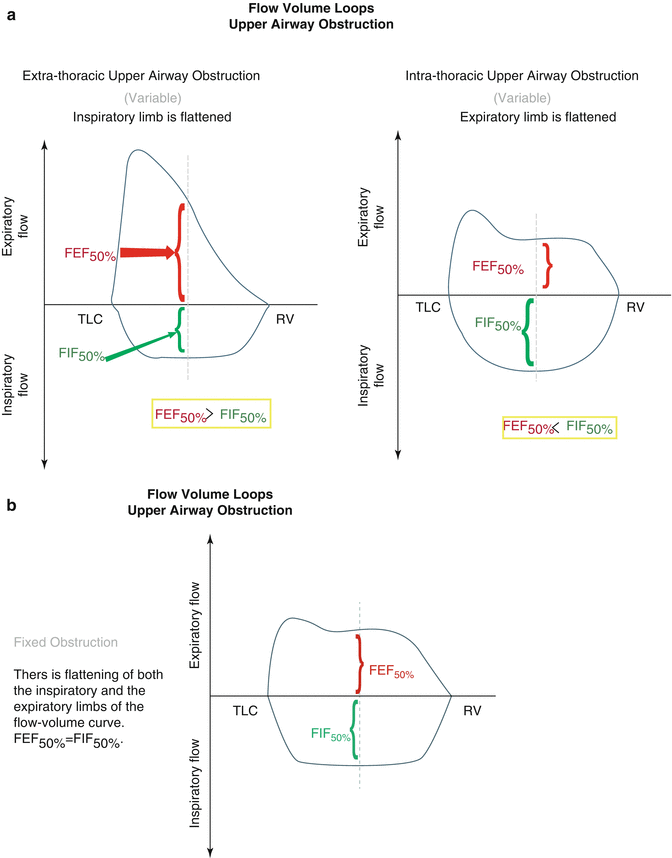
Fig. 30.3
(a) Flow volume loops with extra- and intrathoracic upper airway obstruction. (b) flow volume loops with fixed upper airway obstruction
Although useful, the diagnosis of upper airway obstruction often requires confirmatory tests such as a CT scan of the chest and neck and laryngoscopy or bronchoscopy for direct visualization.
Spirometry cannot measure the FRC, RV, and TLC because there is not a means of determining the volume remaining in the lung after maximal exhalation (RV). The FRC can be measured via two techniques that are based conceptually on Boyle’s law:
(P = pressure, V = volume, and k = a constant value representative of the pressure and volume of the system, and as long as the temperature remains constant, the amount of energy given to the system and the value of K should be constant.)

For a fixed amount of an ideal gas kept at a fixed temperature, pressure and volume are inversely proportional. So for a container filled with gas, if you decrease the volume, the pressure will correspondingly increase and vice versa.
The FRC can be measured by the helium dilution technique or by using a body plethysmograph. The helium dilution technique involves connecting a subject to a spirometer of known volume that contains a known concentration of helium. The subject then rebreathes until the helium concentration in the spirometer and in the lungs is the same. This is determined by collecting all the exhaled carbon dioxide (absorbed by soda lime) and adding oxygen as needed to maintain a constant total volume. Once equilibration has been achieved, the concentration of helium before (C1) and after can be measured (C2) as the total amount of helium is assumed to be unchanged. Because helium is very insoluble, it can be assumed no helium is removed by the blood.
The patient is connected to the system after a normal breathe out at which point the volume of gas left in the lung represents the FRC (functional residual capacity). The FRC can be derived by using the equation C1 × V1 = C2 × (V1 + V2). V1 is the volume gas in the spirometer and V2 is the total gas volume (FRC + volume of spirometer (V1)). A limitation of the helium dilution method is that in patients with obstructive pulmonary diseases, this technique is less reliable because of incomplete equilibration of the helium in all areas of the lungs; in such cases, the body plethysmography may be more accurate.
The body plethysmograph employs an airtight box that the subject sits in and at the end of a normal expiration, a shutter closes the mouthpiece, and the subject is asked to breathe in. As the subject tries to inhale, the gas in the lungs expands, lung volume increases slightly, and the pressure in the box rises slightly because its gas volume decreases. Boyle’s law (pressure times volume is constant at constant temperature) can then be used to calculate the change of volume of the plethysmograph. The equation is P1V1 = P2 (V1–ΔV), where P1 and P2 are the box pressures before and after the inspiratory effort, V1 is the box volume before inspiration, and ΔV is the change in the volume of the box (or lung).
If the mouth pressures before P3 inspiratory effort is measured and after P4, Boyle’s law can be applied to the lung, and the FRC can be derived using the equation:
V2 is the functional residual capacity.ΔV was measured previously.V2 is the only unknown.

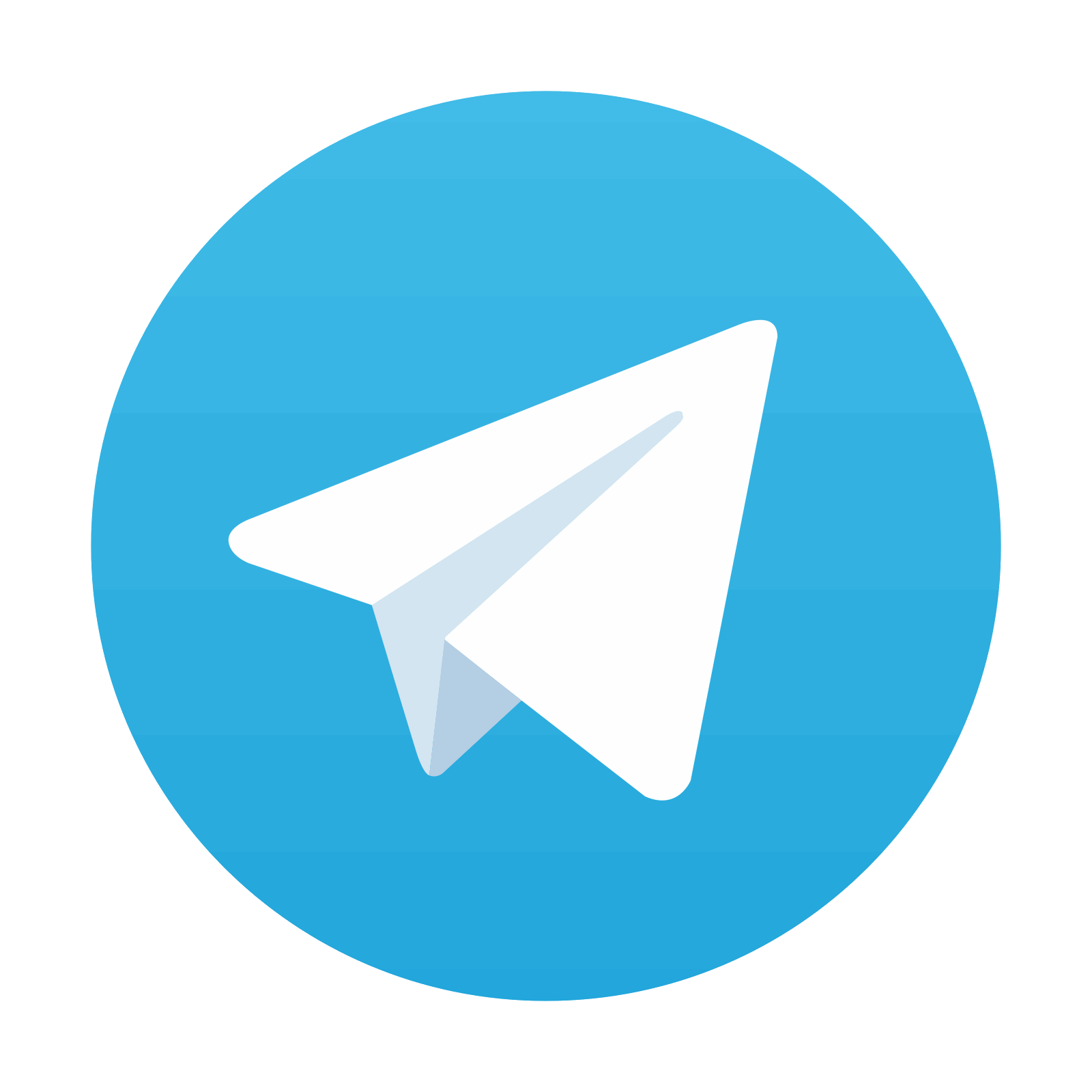
Stay updated, free articles. Join our Telegram channel

Full access? Get Clinical Tree
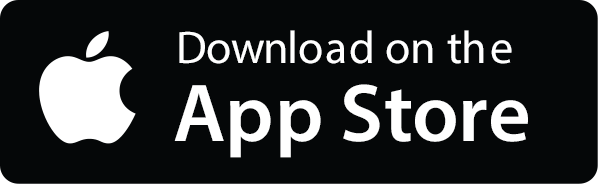
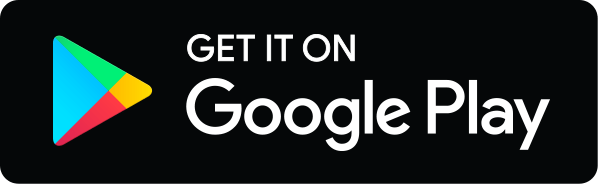