Fig. 11.1
Differential localization of sweat pores of eccrine glands in relation to skin folds and ridges. (a) In glabrous skin, such as fingertips, sweat pores open at the ridges under baseline conditions. (b) In hairy skin, such as forearm, those open at the folds under baseline conditions. (c) Upon thermal stimulus, those in the hairy skin also open at the ridges. Sweat droplets are visualized as small holes corresponding to each sweat pore in the impression mold technique (IMT), as described later
Eccrine sweat glands can respond to both core (internal) temperature and peripheral (skin) temperatures, with the former being much more efficient for both sweat induction and sweating rate [7]. Cholinergic sweat secretion is the major route and also accounts for >70% of sweating capacity in isolated human sweat glands analyzed in vitro [7]. With cholinergic stimulation, there is increased secretion of sweat. Thus, a pilocarpine sweat test is widely used for evaluating the ability to produce or deliver sweat to the surface of the skin in the presence of appropriate stimuli [9]. In addition to thermoregulation, an additional function of eccrine sweat glands is to maintain skin surface hydration by secreting moisturizing factors, such as lactate, urea, and potassium [10]. Recent studies have further demonstrated that sweat glands secrete water including several antimicrobial peptides, including dermcidin (DCD) that can contribute to the first line of defense against invading pathogens by building a constant barrier that overlies the epithelial skin [11]. Additional studies have also demonstrated that sweat contains proteolytic enzymes, IL-1α, IL-1β, ΙL-6, ΙL-8, ΙL-31, TNF-α, and epidermal growth factors [12–15].
Dysfunction of one or more of the processes in normal regulatory mechanisms of sweat production and secretion could be reflected in the development of anhidrosis or hypohidrosis [9]. It could result from diverse causes: they include absence or atrophy of the sweat glands, a direct damage to the glands by trauma or inflammation of the skin, and portal occlusion or dysfunction of sympathetic nerves in neuropathies [8, 9, 16].
11.3 Measurement of Sweat Output
Research on the regulation of sweating responses under various physiologic and pathologic conditions has been hampered by the paucity of reproducible and quantitative evaluation methods, although a variety of methods for sweat testing have been devised [17]. In this regard, we have recently established impression mold technique (IMT) modified from the previously reported plastic impression method (Fig. 11.2) [17–20]: it allows an accurate quantification of individual sweat glands/ducts actively delivering sweat to the skin surface and the volume of sweat they produce over time [18–20]. In the IMT, emerging sweat droplets can be visualized as small holes corresponding to the individual sweat pores. This method is useful for determining the relation between the sweat pores and the surface topography of the skin (Fig. 11.1).


Fig. 11.2
IMT for the assessment of sweating responses. (a) The silicone material is mixed with a water solution and spread onto the skin. (b) As the silicone hardened, it retained the impression of the sweat droplets as they emerged from the sweat ducts and pushed up into the mold. Impression molds hardened in 3–4 min, and they were then removed. (c) The resulting sweat droplets were counted using a dissecting microscope and quantified by number and size per area over a 1 cm2 region. Air bubbles, which were smaller than sweat droplets, can be differentiated from sweat droplets with relative ease
The degree of sweating can be also determined by measuring the skin surface hydration (SSH) at different time points after starting stimulus: measurements of the SSH can be performed before and after stimulus. This method seems to be superior to the IMT in terms of the easiness of obtaining the time course of sweating responses, while the IMT is superior to the SSH in terms of quantitative evaluation of sweating occurring in a well-defined location. To investigate whether sweating responses would be different depending on the localization of sweat pores relative to skin folds or ridges, the IMT is the only informative measure.
Sweating responses can be induced by different means: they include intradermal injection of cholinergic agents, physical exercise, exposure to increased temperature, and immersion of legs in warm water. According to our unpublished studies, immersion of legs in warm water was found to induce most efficient sweating responses in the glands/ducts located either at the folds or ridges, when sweating responses were evaluated by the IMT. In the IMT, sweating responses can be evaluated by both the number of sweat droplets per square centimeter, either at the folds or ridges, and the volume of sweat per square centimeter obtained by measuring the mean diameter of the outline of each droplet (Fig. 11.2).
11.4 Insensible and Sensible Sweating
There are two types of sweating [21]. The first is referred to as “insensible” sweating: in this type of sweating, an individual can secrete sweat under quiescent baseline conditions before stimulus, and water evaporates through the epidermis in an unrecognized fashion. Sweat droplets mainly detected at the folds under quiescent or “ basal” baseline conditions can be regarded as basal levels of sweating or “insensible” sweating (Fig. 11.3a). Because there is a significant positive correlation between the SSH status and sweat droplet numbers detected at the folds, but not those at the ridges, under baseline conditions, sweat excreted from the sweat glands/ducts at the folds would serve to maintain skin hydration under quiescent baseline conditions. For most people and physicians, however, no or little attention has been focused on this type of sweating, despite its critical role for maintaining skin hydration. Perspiration by “insensible” sweating can be estimated to be <600–800 mL through the skin [21, 22].


Fig. 11.3
Sweating responses in the thigh of a healthy subject evaluated by IMT before (a) and 30 min after thermal stimulus. (a) “Insensible” sweating can be detected at the folds. (b) “Sensible” sweating can be preferentially induced at the ridges, which is associated with the increase in size
In contrast, sweating induced by physical exercise or exposure to hot and humid environment is called “sensible” sweating [21, 22]. This type of sweating has received increasing attention over the last 10 through 20 years because it is well known that this type of sweating could function as a major thermoregulator. According to our recent unpublished observations, this type of sweating is mainly mediated by sweat produced by sweat glands/ducts at the ridges (Fig. 11.3b). Sweating responses in sweat glands/ducts at the ridges might also serve as a backup to impaired function of those at the folds, because in AD, compensatory hyperhidrosis was exclusively detected in those at the ridges, as described later [20]. Thus, measurement of sweat output depending on the surface topography of the skin, either the folds or ridges, is useful for elucidating how sweating defects could contribute to the pathogenesis of inflammatory skin diseases.
11.5 Sweating Defects in AD
There have been conflicting data regarding whether sweating responses are impaired, normal, or enhanced in patients with AD. Sulzberger et al. initially reported that there is sweat retention similar to that seen in miliaria in AD skin [23]. Lobitz and Campbell reported normal sweating responses to intradermal injection with acetylcholine and epinephrine in AD patients [24]. Other investigators soon joined the controversy. Rovensky [25] and Warndorff [26] independently reported that AD patients displayed increased sweating responses to cholinergic stimulation, while others demonstrated normal sweating responses [27]. Varying results were obtained depending on whether cholinergic or adrenergic stimulation was used. In this regard, Kiistala reported that sweating responses to either cholinergic or adrenergic stimulation were significantly decreased in AD skin and that sweating disturbances were more obvious in dry-appearing skin [28]. Other quantitative studies showed that a decreased sweating response to moderate thermal stimulus was common in either dry-appearing or normal-appearing skin [29]. Later quantitative study with the use of sudomotor axon reflex test showed that sweating responses to cholinergic stimulation as evaluated by the latency time and sweat volume were retarded and impaired, respectively, in AD patients as compared with those in nonatopic controls [30]. A consensus has emerged from these studies that sweating responses are impaired in AD patients, particularly in dry-appearing skin, and retarded. Unfortunately, however, such conflicting data would have diverted the research interest away from investigating the role for sweating responses in the pathogenesis of AD. In light of such conflicting reports, we were interested in quantitatively determining the capacity of individual sweat glands/ducts to produce and deliver sweat to the skin surface by using IMT, by which sweating responses can be quantitatively evaluated in a well-defined location.
One possible explanation of previous contradictory findings on sweating defects in AD could be that this may have been a function of the time point in the stage progression when sweating tests were performed. To investigate how disease process and sweating defects could interact with each other and progress from early asymptomatic stages to the onset of clinically apparent disease, we suppose that sweating responses in AD patients should be carefully investigated and analyzed depending on the clinical phenotypes and stages. All patients analyzed by us had mild-to-severe disease with typical eczematous skin lesions and elevated serum IgE levels. Thus, patients with adult-type AD were categorized into two groups, based on the clinical phenotypes and stages, acute and chronic. Adult patients with active dermatitis characterized by scaling erythematous papules, plaques, lichenoid papules, and crusting were evaluated as acute AD: most of them had exudate lesions in the flexural areas, usually within 5 years after onset. In contrast, patients characterized by systemic dry skin and relative sparing of eczematous lesions in the flexural areas were evaluated as chronic AD. There was no significant difference in the severity of disease assessed by scoring atopic dermatitis (SCORAD) index between the two groups at the time of enrollment. The mean age of acute AD was significantly younger in acute AD (n = 12, 23 ± 1.2 years) than in chronic AD (n = 15, 35.5 ± 2.3 years). Twelve unrelated, sex- and age-matched, healthy subjects with no history or family history of AD were enrolled in our study and served as healthy controls.
Sweating responses were induced by two means: the first means was immersion of both legs for 30 min in a water bath maintained at 43 °C. The other means was the injection with 500 μL of acetylcholine (Ach). Sweating responses thus induced were evaluated by the IMT, as described previously [17–20]. Sweat droplets in healthy controls under baseline conditions before thermal stimulus were distributed evenly and usually occupied each dermal fold in the forearm and abdomen, in which no significant difference can be found. Because there was an intimate association between the SSH levels and numbers or sizes of sweat droplets detected at the folds, but not those at the ridges, it is likely that the SS|H levels under quiescent baseline conditions would be determined by these basal levels of sweating (insensible sweating). These results suggest that dry skin in AD patients would largely result from the impaired “insensible” sweating in the ducts/glands at the folds. After thermal stimulus, sweat droplets were also detected at the ridges and increased in number and size with time.
In the normal-appearing uninvolved skin of acute AD, the number of sweat droplets observed at the folds was dramatically decreased not only before thermal stimulus (Fig. 11.4a) but also 15–30 min after thermal stimulus: this trend was evident even at the earliest acute stage, in which skin surface structure as evaluated by the surface topography of the skin was not disrupted yet by the disease process (Shimoda Y. et al. manuscript submitted). In the involved skin of acute AD, the number of sweat droplets at the folds was also profoundly decreased, while the size of sweat droplets was larger, particularly those at the ridges, than those in healthy controls: larger sizes of the droplets could be interpreted as implicating compensatory hyperhidrosis to maintain thermoregulatory function. The compensatory hyperhidrosis became most apparent at the ridges in the uninvolved skin sites of acute AD. In contrast, sweat droplets observed in chronic AD were much less numerous in number and smaller in size than those in acute AD and controls. The severity of disease as assessed by SCORAD was positively related to the number of sweat droplets at the ridges in acute AD, while that was inversely related to those at the folds in chronic AD. Thus, hyperhidrosis could be preferentially observed in the sweat glands/ducts at the ridges in acute AD, while systemic hypohidrosis observed either at the folds or ridges in chronic AD could contribute to the progression to the full development of chronic AD characterized by systemic dry skin.


Fig. 11.4
Insensible sweating responses in the forearm of the earliest asymptomatic stage of acute AD evaluated by IMT before and after treatment of 3 FTU of a moisturizer. (a) The number of sweat droplets at the folds before treatment, i.e., insensible sweating responses, is profoundly decreased despite no significant changes of the surface topography. (b). One week after treatment with 3 FTU of a moisturizer, the number of sweat droplets located at the folds, i.e., insensible sweating responses, is markedly increased
We next asked whether decreased sweating in AD patients could be due to their inability to produce sweat or to deliver sweat to the skin surface. According to our previous studies [18, 19], the presence of sweat in the skin outside the sweat glands/ducts can be immunohistochemically identified by staining the specimens with anti-DCD Abs. In healthy controls, DCD antigen was solely detected in the cytoplasm of dark cells in the sweat glands and sweat. In the acute AD lesions, DCD antigen was also detected in the cytoplasm of dark cells in the sweat glands of the involved skin of acute AD at much higher intensity than those in healthy controls, excluding the possibility that decreased sweating responses in AD patients could be due to their inability to produce sweat. We reasoned that if it were possible to detect the presence of DCD in the dermis or epidermis outside sweat glands/ducts after thermal stimulus, then the leakage of sweat from the sweat glands/ducts could be the cause of both the profound decrease in sweating responses and inflammation. To test the possibility, we immunohistochemically identified DCD antigen in the skin lesions of AD after thermal stimulus. Surprisingly, DCD antigen was detected not only within the glands/ducts but also in the dermal tissue adjacent to the glands/ducts (Fig. 11.5), indicating leakage of sweat either from the glands or ducts into the dermis. These findings could be interpreted as indicating that in AD patients sweat, instead of pouring out and being removed from the skin surface, may diffuse in the dermal tissue around the grands/ducts and that, as a result, the ability to deliver sweat into the surface could be impaired. Because sweat has been shown to contain various inflammatory cytokines [12–15, 31–33], it is likely that leakage of sweat into the dermis could initiate a complex sequence of events that promotes eczematous inflammation. In view of a recent observation that the areas of anhidrosis on thermoregulatory sweat testing correspond to their symptomatic areas such as itching and strange sensation [34], itching and strange sensation frequently detected in AD lesions could now be explained by leakage of sweat into the dermis associated with anhidrosis, rather than by small-fiber neuropathy.


Fig. 11.5
DCD expression can be detected not only in the cytoplasm of dark cells in the sweat glands of an AD patient but also in the dermal tissues around the glands
Sweating responses to Ach were also significantly decreased in sweat glands/ducts at the folds in acute AD as compared with those in healthy controls, a finding similar to those after thermal stimulus. These results suggest that sweating defects observed in AD patients would be due to dysfunction of sweat glands/ducts themselves. Our finding indicate that leakage of sweat into the dermal tissue around sweat glands/ducts was most evident in the involved skin of acute AD and that this leakage of sweat may represent the actual early events that presumably occur well before patients have typical dry skin: the sweat duct fragility may be related to putative defective function of tight junction (TJ), which can prevent the free diffusion of macromolecules.
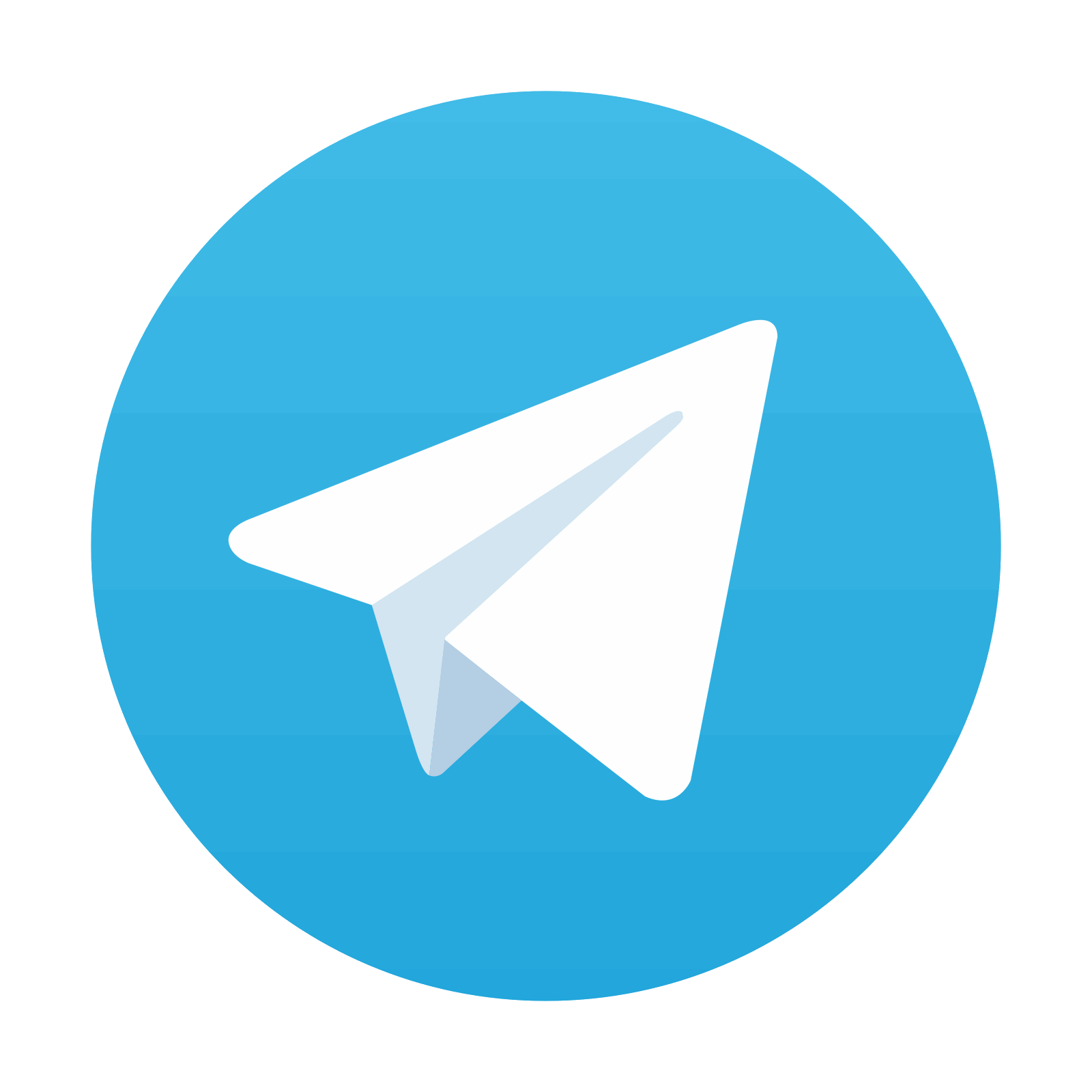
Stay updated, free articles. Join our Telegram channel

Full access? Get Clinical Tree
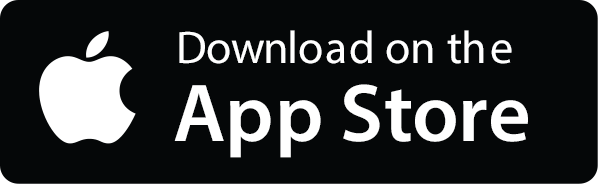
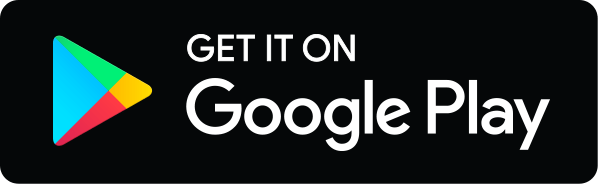