Chapter 2 The epidermal appendage previously referred to as the pilosebaceous unit, and renamed the folliculopilosebaceous unit (FPSU), is an extraordinarily complex organ (Figure 2.1). It has still not given up all its secrets, and this chapter provides a summary of what is known of the normal FPSU, and what influences our numerous FPSUs to swing them from their normal paths, creating the acnes. Figure 2.1 The follicular unit consists of the intraepidermal acroinfundibulum (A), the intradermal infrainfundibulum (B) that extends to the top end of the isthmus (C), and then continues as the pilar unit (D) containing the hair papilla and follicle. The sebaceous unit is composed of all the sebaceous glands that join the isthmus (C) at the sebofollicular junction (E). It is a 360° structure that wraps around the isthmus. The sebocytes in the sebaceous glands are the sebum-producing units of the FPSU. These glands are found most densely populating the shawl distribution over the convex surfaces from the vertex of the scalp to the horizontal nipple line of the upper trunk, reflecting their role of providing the prime lubricant in the process of parturition (birth and delivery) [1]. Their concentration elsewhere is related to the somewhat variable coverage of the remainder of the trunk and limbs by hair-bearing FPSUs. The number of sebaceous lobules attached to the base of the follicle at the sebofollicular junction varies from zero to a cluster of perhaps six. The number of FPSUs is likely genetically determined, and the size of the lobules reflects both genetics and the quantity and quality of hormonal stimulation. With age, the sebaceous gland activity fades gradually as hormone levels drop. Significantly, where the prime function of these glands dictates the highest population, over the face and scalp, oiliness persists into old age, especially in those areas that enjoy a lifelong testosterone stimulus. The hands and feet are spared this oily population, likely on a survival basis. A slightly moist grip and plantar surface is better suited to fight or flight than a greasy one. A few sebaceous glands may be found around several orifices, ectopically on the labial vermilion of the lips and the genital labia minora, and naturally in the external auditory canal, in the nasal vestibule, and modified as Meibomian glands in the eyelid margins. The double strands of DNA that carry the genes we inherit from our parents determine whether or not we are likely to suffer from acne, which kind, and probably how bad it can be. If you have acne, the genes had to come from somebody, and even if you figure out who gave you the acne genes, it is too late to change your parents now. You must do the best you can with the genes you were dealt. It is just like playing poker. On the other hand, if you do not have acne, that does not mean you do not have the genes. The problem is that some genes are fully expressed in all the people who have them (like brown eyes), while other genes (like for freckles) only show up (are “fully expressed”) if the conditions are right. For freckles, you need to add sun exposure. For acne, you need to add hormones. And if you add the hormones without having the genes for acne, you will not get acne. Lucky you! So let’s get a little deeper into this. If neither of your two parents had acne, it does not mean that they are not your parents. The rules of heredity are complicated, and if your mother had just enough acne genes to give you a “half dose,” then she could easily have had no acne as a teen. The same may be true with your father, but with two half doses, one from each parent, you could get a single full dose of the genes required to give you acne. You just got unlucky. To use an analogy that you will see again, you were born with a loaded gun. You will need to avoid anything that wants to pull the trigger. A recent study from Italy rates the risk of acne, if one has affected first-degree relatives, at 3.41. So if you have one parent or sibling with acne, the risk of getting acne is almost 3 1/2 times that of the rest of the population who is your age but does not have affected relatives [2]. Just about every patient I see with acne vulgaris has a positive family history. Acne is not always present in other family members, but when the family history is positive, it is both variable and complex. For instance, I am aware of a family with two daughters. One of them has never been troubled by acne. The other one had acne that was so bad at age 11 that she required a course of isotretinoin. The father and his side of the family have almost no acne, and the mother also had very little. The girls’ maternal uncle, on the other hand, suffered terribly with acne on his face and back as a teenager, and still has problems as a senior. Although their mother was not affected, she seems to have carried the gene, whatever it (or they) might be, through to the younger daughter. There are some very good studies on genetics and acne, the best being those that studied twins. It’s not a surprise to me that there is a relationship, but it is worth noting that the expression of some genes rests upon environmental exposures long after the genes are provided by the parents. New babies are born with unfreckled skin but, under the influence of sunlight, one will develop freckles later, and another will not. There are related markers, such as red hair, that can predict which one will likely develop freckles, but there’s no guarantee. There are redheads who have flawless “peaches and cream” complexions that never freckle. This is a very rough illustration of the direct influence of our environment on the actual expression of our genes. It is simple and straightforward activation of the genes that were set at the time of conception. There is another, more complicated influence that needs consideration, a more subtle process that is hidden in the background, and about which we are only now learning. This is the problem of our genes’ exposure to various chemical and other influences after conception, during gestation (pregnancy), during early life, and during the process of sexual development and maturity. This field of study is called epigenetics. More about that in Section 2.3. Meanwhile, for clarification, the “instruction set” that you are born with, located in the DNA within your genes, is called your genotype. What those instructions actually turn out as a product (what you actually look like) is called the phenotype (from the Greek word pheno meaning “to show, to appear, or to display”). A genotypic freckled redhead (someone who has the genes to make red hair and freckles) who is never exposed to the sun will never be troubled with freckles. His or her genotype will not be expressed as a freckled phenotype unless there is a certain environmental exposure, in this case sunlight, but the red hair will be there whether there is sunlight or not. I raise this point because some people simply do not seem capable of getting acne. While this makes life a lot easier for them than their pimply brothers and sisters, it does make life difficult for researchers experimenting on the cause of acne. Some study subjects are simply incapable of developing acne. Including such individuals in a study designed to trigger acne would give inaccurate results, because the results of the studies would always be negative in such persons. Only persons who can get acne should be included in dietary studies on acne, for instance. Lucky people with flawless complexions just won’t get acne. This is a small percentage of our Western population, but other populations may be different. Over a decade ago, Loren Cordain described two tribes on different sides of the world that did not have acne, both tribes in their aboriginal habitat. One group inhabits the island of Kitava, one of the Trobriand Islands in Papua New Guinea’s archipelago. The other, the Aché, live in the jungles of Paraguay. Both groups are hunter-gatherers with predominantly vegetarian diets. The Trobriander people have been thoroughly studied by social anthropologists and human ethnologists, but medical reports have been few. As isolated groups, the tribes’ genetics were stable and the environmental influences from person to person within the tribe were likewise pretty much the same, particularly their diet. Lindeberg et al. reported, “The residents of Kitava lived exclusively on root vegetables (yam, sweet potato, taro, tapioca), fruit (banana, papaya, pineapple, mango, guava, water melon, pumpkin), vegetables, fish and coconuts. Less than 0.2% of the caloric intake came from Western food, such as edible fats, dairy products, sugar, cereals, and alcohol” [3]. In eastern Paraguay, the Aché tribal diet contains wild, foraged foods; locally cultivated foods; and 8% Western foods (mainly pasta, flour, sugar, yerba tea, and bread). Cordain’s study reported that members of these tribes had no problems with acne whatsoever [4]. The diets, very low in processed food and generally presenting a low glycemic load [5], were given credit for this lack of acne. At the time, the paper was criticized for attributing the lack of acne to the diet because it was thought that these populations might have been genetically incapable of developing acne. It was even suggested that these populations be subjected to a Western diet to see whether or not they would develop acne [6], a reasonable scientific approach in an ethics-free world. Following up this question in discussion with Prof. Cordain, I learned that some of these individuals, when exposed to a so-called Western diet, do indeed develop acne, which certainly suggests that some of them really do harbor the acne genotype. That certainly has been the pattern in other previously isolated groups. Further testing with dietary challenges would be needed to determine the frequency of the gene(s) for acne in that population, work that would challenge ethnologic ethics. There are other populations that had essentially no acne before the advent of the Western diet. Acne is now endemic among the previously clear-skinned Inuit in the Canadian Arctic [7]. The Japanese and others have been the subjects of several papers [8]. It seems, therefore, that the genetic tendency toward acne is present in most human populations, probably all of them. The likelihood is that the genetic background is expressed only when the FPSUs are challenged by a specific stimulus, and it is generally accepted that a change in the hormonal environment has that effect. This theme and its mechanism will be fully developed elsewhere. (See Section 2.8.) It is not only aboriginal populations that develop acne when genes and diets meet. The multicenter study in Italy quoted above [2] has shown acne positively correlated not only with family history, but also with dairy intake and mild obesity. In the population studied, three factors stood out. In addition to an increased risk of 3.41 times triggered by a family history of acne in parents or siblings, being overweight increased the risk of acne by 1.3 to 3.3 times. Third, milk consumption (three 250 mL glasses or more per week) increased risk in the entire population by 1.78 times for any kind of milk and 2.20 times for skim milk [2]. There was no significant correlation to menses and a negative correlation to one serving of fish per week. It is important to consider that these dietary risks apply to the entire population studied. That includes, at one end of the curve, the subjects who may have no genetic risk of acne at all. They bring down the risk artificially because they have no innate risk of developing acne no matter what diet they consume. At the other end of the curve are those whose acne will be triggered by only small amounts of milk. Remember these results when you get to Section 2.8. The discussion above relates mainly to acne vulgaris, the acne that is common among 85–90% of North American teenagers. With regard to acne rosacea, many of my rosacea patients report that their parents, in their middle and later years, also had acne rosacea. There is little doubt in my mind that this is also genetically influenced, but early work done on this facet of this disease is only beginning to surface. It is said that “genes rule.” While there is little doubt that they load the gun, there are many different influences that can pull the trigger. One study suggests that of the numerous inflammatory mediators that are active in acne, some are heritable as single genes that often control enzymes, neuroendocrine transmitters, and cytokines found in the pathways that lead to acne rosacea’s signs and symptoms [9]. A specific genetically determined defect in the skin’s ability to handle reactive oxygen species (small, chemically active substances that cause damage) has been correlated with rosacea [10]. On the other hand, in a study of twins, only one of a pair of homozygous (identical) twins developed acne rosacea, suggesting that a nonheritable variable made the difference and was the trigger [11]. It would certainly be no surprise to learn that, ultimately, heredity and environment interact. Just like the freckles and the sun we started with. That this disorder is hereditary to some degree is accepted. A positive family history occurs in 35–40% via autosomal dominant inheritance. That means that each child has a 50:50 chance of inheriting the disorder, and that if you had 100 children then 50 of them would likely inherit the gene (provided only one parent is a carrier). But Mother Nature can be a devious parent at times and it is possible to have four to six involved (or uninvolved) children in a row. Explanations other than random selection and luck are lacking. The family history in AI is often less well defined than in acne vulgaris because family members do not always report the existence of their disease. It is somewhat hidden from view, it is often undiagnosed, and its existence is often denied. Indeed, without the trigger, the genotype would not show up as the phenotype. Nevertheless, I have treated many mother–daughter (and even one granddaughter) groups with this disorder, and a few father–daughter families have shown up over the years. Interestingly, the father–daughter pairs shared a very disfiguring and scarring nodular form of facial acne as well as the acne inversa. And last year a mother showed up with her four-and-a-half-year-old son, who has a history of small “boils” on his buttocks. The mother has mild AI/HS herself. So did her father. And his father. Four generations. Details of the molecular genetics are emerging. For example, two novel mutations of the nicastrin gene have been associated with the disorder in two Chinese families [12], but it will be a while before we know exactly how they are related to expression of the disorder. Meanwhile it appears that this same problem is not common among AI patients. How the pieces of this giant puzzle fit together remains a mystery. I met Meghan when she was 28. She had had acne since she was 12, and was getting more than a little tired of it. Although there was some variation with her periods, the problem persisted and was active pretty much all the time. She was not sexually active, had never been on any hormonal contraceptives, and was generally content with her life, with minimal stress. She presented during the early years of my practice when I was collecting dietary data. Meghan had moderately severe nodular acne involving both cheeks, particularly the jawline and under the chin. She had tried numerous treatments; had been on antibiotics, particularly tetracycline, for years; and was pretty fed up. During the “family history,” she told me that she was an identical twin, and her sister had never had so much as a single “spot” for as long as she had known her. When I got to asking her about her dietary intake, and in particular her dairy intake, her face lit up. Her eyes widened, and she said to me, “Oh, my goodness, it must be the cream in the milk.” She told me that when she grew up in Scotland, the milk arrived on the doorstep in bottles, and was not homogenized. That meant that the cream had separated, was sitting on top of the milk, and would pour off first when the lid was removed from the milk bottle. She told me that she always wanted to be the first to bring in the milk bottles in the morning, because she loved to use the cream from the top of the bottle. Her sister, quite to the contrary, would drink milk only when forced, and only from the bottom of the bottle. Meagan agreed to continue with her standard therapy, which at that time consisted of oral tetracycline and topical retinoic acid cream, but to discontinue all dairy products. She started to clear within 2 months, at the end of 6 months she was able to discontinue the tetracycline, but it took a full year before I could convince her to let go of the retinoic acid. I did not ask her to return to her dairy, and I never saw her again as a patient. She was one of my first successes in dietary management involving twins. Fortunately, it was not necessary to “double blind” the study, because her identical twin sister had already served as a control. Asking a pair of twins to decide which one of them should go off dairy and which should stay on dairy presents problems not only in interpersonal relationships but also in ethics. This is especially true in private practice, where patients present themselves for the best care possible, and are not expecting to be “guinea pigs.” This is a relatively new field of study. We know that DNA-based genes set the basic rules for reproduction, growth, and function, but there are ways in which the guidance provided by your original genes can be modified. One of the influences on the development of acne may be through epigenetic changes mediated by diet. The sum total of all our genes (what we now call our genome) governs our genotype, basically our genetic typeset. It is represented as a long string of code made up of four letters, typed in on a keyboard that has only four keys—C, A, T, and G. These four letters spell out the DNA code for everything our 23,000 genes can possibly do. Your DNA has instructions for thousands of individual biological reactions. They can be combined in an almost infinite number of ways to direct the many things you do every day, like making oil in your oil glands or growing nails and hair. Others are things you do only once in a while, like heal a cut or have a baby. And there are things your body may never do, like have a bad reaction to a certain drug. The instructions are there in your DNA, waiting. At conception, your parents load your genome with your special customized mix of Mom DNA and Dad DNA. Your personal unique genotype produces you as a unique person. What is produced (hair and eye color and acne tendency) is your phenotype. The pheno part, as mentioned, comes from a Greek word meaning “to show.” So your genotype is what is hidden in your genes and your phenotype is what shows. For DNA to direct a specific task, a mirror copy of a small part of your DNA is made of RNA. This bit of RNA is the messenger that tells your body what protein or other body chemical to make, to do whatever is needed. It is called, logically enough, messenger RNA or mRNA. Epigenetics adds to the mix by causing some very small changes in the DNA. One such change is the addition of a methyl group to cytosine (the C) or adenine (the A) in the DNA itself, a chemical change in DNA called methylation. Another is the formation of “bookmarks” in the chromatin. These (and several other mechanisms) can lead to certain genes in your DNA being turned on (or off) so they are allowed to make (or are prevented from making) a certain RNA. This changes the DNA’s message from doing a certain thing to not doing it, like an on-off switch. In actively reproducing cells, these changes may continue throughout the life of that cell line. There is evidence that, if these tiny changes impact the DNA in sperm and ova, they may be hereditary and can be passed along to offspring. Think of epigenetic change as a “program update” downloaded from your environment onto part of your genome. The source may be a drug, a toxin, or even a dietary change [13]. Unfortunately, we have no effective protection against such changes, triggered by influences as different as a low intake of folic acid or a high intake of arsenic. Foods, alcohol, drugs, hormones, heavy metals, cigarette smoke, and occupational chemicals—all are suspect. The list is growing, but so far there are no specific studies in acne. As an illustration, however, it has been shown that feeding royal jelly to create queen bees from worker bees (both of whom have the same genome) seems to be mediated by some 550 methylation differences between the queens and the worker bees [14]. These direct the remarkable changes, based on diet alone, that produce a new queen. It is not difficult to imagine that changes in dietary hormonal intake over a period of time can make significant changes. In one Harvard study, preteen boys who drank milk were about one inch taller than those who did not [15]. There was no other significant difference in their diets, and a recent study confirms this dietary influence on linear growth [16]. This raises the question of the average increase in height that is apparent throughout the entire population in North America, compared to the population in China or other societies consuming less or no dairy. Is this just better nutrition in general, the effect of increased amounts of insulin-like growth hormone 1 from drinking milk, or is this the result of an epigenetic influence? “You are what you eat” may boil down to “You are what your dietary epigenetic influences tell you to be.” My father was a dermatologist, and I joined his practice when I completed my training. He had been caring for a patient from upstate New York, a farmer’s son, with terrible acne. At that time, there was a “digestant” on the market in Canada that contained bromide salts and there is a disorder called bromoderma that looks like acne. My father had seen a couple of cases, and he wondered whether there might be a link between bromides and this teenage boy’s acne. As it happened, there had been some contamination of the well on the farmer’s property, and methyl bromide was at fault. It had been used in the agricultural process of soil fumigation and had gotten into the well. Suspecting that this was the cause of the acne, and being unable to obtain blood levels of bromine at that time, my father attempted to “wash out” the bromide by the pragmatic prescription of sodium chloride tablets to tolerance. Achieving no significant results after a month of this, he asked me for some assistance with the case. I had just begun working with milk and other dairy products as a possible cause of acne, and I went through this young man’s dietary history for a few minutes. It turns out that the farm was a dairy farm, and the lad consumed roughly two quarts of unpasteurized whole milk daily. He was 17 at the time, and was accompanied to the visit by his 15-year-old brother as well as their parents. The younger brother was likewise involved with acne, over his face, neck, shoulders, and trunk. He was also a very aggressive consumer of his cows’ product. At that time, isotretinoin was just becoming available but these young lads were from over the border in New York State, and so I could not prescribe it for them. Instead, I simply asked them to discontinue their entire intake of dairy products, continue on the tetracycline that was the standard at that time, reduce it if possible, and check back in a few months. Winter intervened, but they did show up the following spring, and their active lesions were essentially clear. A couple of years later, I got a telephone call from the boys’ mother. Their 11-year-old brother was now 13, and he was starting to develop acne. What should she do? Could she bring him over to see me? I was surprised to learn that, despite the fairly solid relationship between dairy withdrawal and her older sons’ clearance, the youngest son was still drinking lots of raw farm milk. I suggested that this be discontinued, she agreed, and that was the end of the story. Some years later, the mother saw me for an unrelated problem and confirmed that once the youngest brother stopped the dairy, they never needed my services. The epidermal appendages, true to their Latin roots, “hang down from” the epidermis. They start life in the fetus at an early age, during the fourth month of gestation. They need time to grow because they have work to do in assisting the fetus to eventually leave its watery home and enter the outside world. The first sign of the FPSU is a small collection of cells budding down into the dermis from the thin fetal epidermis. As the bud grows, it extends deeper into the dermis, during the fourth and fifth months, as a cord or column of undifferentiated epithelial cells. Those destined to become sweat glands head deeper into the fetal dermis. The cord that is the FPSU-to-be develops a collection of cells at its deepest extent that becomes the hair papilla, and this generates the fine fetal lanugo hair, starting at five months. Outgrowths near the top of the FPSU become differentiated into sebaceous glands; the cords forming eccrine sweat glands elongate and coil in the deeper dermis; and the cords destined to become mammary glands sit quietly in their designated and appropriate locations awaiting adrenarche and pubarche (the two phases of puberty). The FPSUs get to work almost immediately, producing both the fatty, oily lipid called sebum and the keratinocytic part of the slippery, complex, and multifunctional vernix caseosa that covers us as newborns. Among the several postulated functions of vernix, the most important, in my opinion, is lubrication. I quote (with a blush) the only published reference relating the hormonal stimulation of the sebaceous glands’ prime function to their subsequent role in the problem of acne: To understand the function of sebum and by extension the reason for our sebaceous glands, one needs to look at the beginning of our lives. We enter the world through a marginally accommodating passage that, under the pressure of the birthing process, is stretched beyond its capacity to provide its own lubrication. Substantial secondary lubrication must be supplied and it needs sufficient substantivity to avoid being squeezed out by the pressures involved. The extra lubrication is supplied by the sebaceous glands that cover the very surfaces that require it, namely those convex surfaces in direct contact with the birth canal. These include the vertex itself and the scalp anteriorly over the forehead and nose to the lower jawline and posteriorly over the shoulders and upper aspect of arms and chest to the extent of what has been called the “shawl distribution.” Evolution has provided fewer sebaceous units inferior to the jawline, in the axillae, on the lateral chest surfaces, and on both the flexural forearm surfaces and the medial aspects of the upper arms — all sites that normally require no such lubrication, having no “trail-breaking” role in a normal vertex presentation and delivery. Evolution has also provided us with apparent proof of this explanation, in the form of an absence rather than the presence of a clinical disorder. Although there does exist a strain of sebaceous gland-deficient (asebia) mice, and although congenital atrichia and anhidrosis are compatible with life in human beings, evolution appears to have selected out as incompatible with efficient reproduction any gene that would lead to what would be termed “asebia totalis congenita,” if it existed. Physical examination of newborns, once one achieves the firm but gentle grip required to evade the lubricating function of the vernix caseosa, often reveals a very prominent population of hyperactive sebaceous glands over the cutaneous convexities (Figure 2.2). Indeed, although never subjected to measurement, the ratio of sebaceous gland mass to body mass by simple observation is doubtless greater at the time of birth than at any subsequent point in human life. How is it that these little oil glands achieve such prominence just before delivery? It is likely because of the concurrent stimuli of two placental products, the 300 mg/d of progesterone piped directly to the fetus through the umbilical cord and the somatomammotropin known as placental lactogen. Whether the latter should be renamed placental sebolactogen will not be debated here, but this molecule has two targets (fetal sebaceous glands and maternal mammary glands) that share both their embryologic origins and their need for maximal stimulation at this point in the reproductive story. Later in life, a different somatomammotropin, growth hormone, acts synergistically with progesterone’s more potent androgenic relatives to re-stimulate these same sebaceous glands and plague us with acne. I would like to suggest that the lowly sebaceous glands’ crowning achievement is to actually achieve “crowning.” This is the penultimate act of the birthing sequence and heralds the arrival of a new life in our beautiful but troubled world. Thanks to our sebaceous glands, the voyage is less traumatic than it would be otherwise. [1] Figure 2.2 We have more natural sebaceous gland activity at birth than at any other time of life. The sebum and water mix called vernix caseosa is what lubricates our way into the world. Acne is classically referred to as a disorder of the pilosebaceous apparatus. The problem with that designation is that acne is not a disorder of the pilar unit. And acne is not a disorder of the sebaceous gland unit, except secondarily. It is actually a disorder of the function of the sebo follicular junction (See Figures 0.21 and 2.1.) To discuss its intricacies and its disorders, we need a new name for this multifunctional, multitasking, and multipotential cutaneous appendage. For reasons that will be more obvious and relevant in the discussion on acne inversa, I use the term folliculopilosebaceous unit, or FPSU. (See the Introduction.) The follicular part of the FPSU is classically called the infundibulum, and there is no reason to change that. It is basically a tube made up of layers of concentric maturing cells. If you cut it in cross-section, it looks like the layers of an onion or the rings of growth in a tree. The actively growing cells start in the outside basal cell layer and move inwards. With time and as a result of metabolic changes, they turn into thin flattened ductal keratinocytes. These are pretty much the same as normal skin cells on the surface of the skin, the thin, flat, layered little fellows called epidermal keratinocytes. Their last living function is to separate from each other, a process called terminal differentiation. Once these cells are fully differentiated, which is a complex metabolic process, the links between them are dissolved by a protease and they separate from each other and are then free to move in the duct. Together with the hair and the sebum, they flow up the central hollow channel to the surface. This hollow cellular tube is wrapped in a closely fitted layer of supporting tissues. The first layer just outside the basal cells is actually the extension of the basement membrane. That is the thin layer of tissue between the epidermis and the dermis of our surface skin. Beneath the epidermis, its job is to anchor the bottoms of the basal cells of the epidermis above to the dermis below (Figure 2.3). It then extends down from the epidermis and wraps around the various components of the “pilosebaceous unit,” now the FPSU. The horizontal surface basement membrane turns and extends downwards to become the vertical glassy or vitreous layer that wraps around the follicle and the rest of the FPSU. It is continuous because both are produced under the influence of the keratinocyte basal cells above them (Figure 2.4). This glassy or vitreous layer, although it is generally equivalent to the basement membrane, is thicker where it wraps around the pilar unit, lending support. It has a somewhat glassy appearance, hence its name. It is visible on light microscopy with the usual stains but is best demonstrated by using a special technique, the periodic acid–Schiff (PAS) stain. This makes certain substances show up a bright pink (PAS-positive) under the microscope. It makes them easy to see and allows us to estimate or measure the thickness of the basement membrane and its relatives. Figure 2.3 The periodic acid–Schiff positive (PAS+) structure that separates the epidermis above from the dermis below is generally homogeneous and well formed, as is the similar material investing vascular and other appendageal structures. Figure 2.4 Note the well-defined periodic acid–Schiff positive (PAS+) support to the right wall, with no inflammation. On the left, there is marked destructive inflammation from 6 to 9 o’clock, where a sebaceous gland has only a small stump left. The glassy or vitreous basement membrane layer merges into the fibrous root sheath. It is also PAS + and is composed of thick collagen bundles. This sheath wraps around and contains the follicular portion of the FPSU like a vinyl glove, and extends down to wrap around the pilar and the sebaceous parts as well. This layer performs two very important jobs. First, the tightness of the wrap provides very significant support to the otherwise weak tubular cellular structures. This wrap limits their expansion and under normal conditions prevents them from overexpanding or simply bursting. The thickness of the wrap is particularly obvious in the eccrine sweat glands and their ducts—they are only one cell thick and would easily burst without this support (Figure 2.5). They have very prominent PAS + basement membranes. The same is true of the apocrine sweat glands and their ducts (Figure 2.6). Figure 2.5 Despite the destructive inflammation applied to the sides of the upper portion of this pilar structure, just below the isthmus, there is little to no involvement of the eccrine ducts on the right—hiding effectively behind their periodic acid–Schiff positive (PAS+) protective wrap. Note that the sebaceous glands have been reduced to stumps. Figure 2.6 These apocrine glands exist in a sea of inflammatory cells, with no suggestion that they are the focus of the activity. There are one or two inflammatory cells beginning to push past the strong periodic acid–Schiff positive (PAS+) protection at 4–5 o’clock on the left and at 6:30 o’clock on the right, substantiating the concept of their secondary involvement. Second, the membrane provides a semipermeable barrier that provides physical separation between the body’s “inside” and its “outside.” Remember that the basement membrane under the epidermis separates the epidermis, which is considered “non-self,” from the underlying dermis, which is considered “self.” Material that is enclosed within the glassy membrane is a downward extension of the “non-self” epidermis (Figure 2.7). If you can think of the relationship between these two compartments as “self-inside” and “non-self-outside,” it gives you some idea why there is such an inflammatory reaction when the duct bursts, releasing “non-self” materials under the skin into the “self” dermis. If you ever had an ingrown hair, you can now understand why the body reacts to this foreign material. This material, your own hair, is considered by the part of your body beneath the epidermis to be something that was not supposed to ever be in the dermis. It was supposed to be out on the surface of the skin, well beyond the basement membrane and its extensions. This is important in understanding why the immune systems (both innate and adaptive) do what they do. More on that later. Figure 2.7 The basement membrane (shown as pink under the epidermis) wraps around the whole folliculopilosebaceous unit, like a vinyl glove. Anything above the pink layer, and anything within the vinyl glove equivalent, is considered “non-self” or “foreign” by the inflammatory guardians of the integrity of the inside “self” area. The three parts of the FSPU are all designed to grow. Each produces its special product, ductal lining cells, hair, or sebum. The growth and replacement of new ductal lining cells, as well as the production of hair and sebum, are turned on by hormones. Androgens (the male hormones) turn on beard hairs (in both sexes). They also turn on oil glands. And they turn on the ductal lining cells, the keratinocytes that line the central canal of the follicular part of the FPSU. Adding more stimulus (androgens and other hormones) to the system, and then adding more time for the reaction to occur, leads to an increase in production of these ductal lining cells. That increase in production results in effects well beyond what was supposed to happen in nature. That is why excess androgen effects produce moustaches and facial hair in women with certain hormonal disorders. And that is why excess androgen effects cause the acnes. Normal levels of androgen working on the follicular part of the FPSU yield a well-regulated production, growth, and elimination of the keratinocytes lining the duct. A finely tuned process called terminal differentiation [17] regulates the speed at which new cells, once produced, fulfill their destiny as detached keratinocytes and are lost up the follicular duct. We know that some human populations that are not exposed to the acnegens in our Western diet do not have acne. That is the baseline I choose to use in providing a rational explanation of acne’s development. When androgens and other hormonal acnegens are at normal levels, uninfluenced by unnatural hormone sources, no acne is produced. When the level of acnegenic activity exceeds the threshold of our individual tolerance, increased amounts of hair or sebum or ductal lining keratinocytes are produced. Increased hair may be a social problem, but hair doesn’t generally get stuck in the pores or become ingrown as a result of increased androgens. The reason is simple—pressure from below, from growth in the hair bulb, pushes the hair shaft to the surface. Likewise, increased sebum production may have sent an earlier generation of women to mirrors to powder their noses, but sebum does not get stuck in the pores. Sebum is a slippery oily liquid at body temperature, and it simply percolates up the follicular canal of the FPSU and onto the surface of the skin. Even when the follicular canal is so choked with keratinous material that a very “tightly inspissated” comedo results, sebum finds its way to the surface [18]. Never in years of “acne surgery” have I ever come across a pool of free sebum “trapped under the skin.” “Trapped sebum” as a cause of acne is a misconception that has inappropriately blamed sebum as a cause of acne for well over 50 years. Sebum is a bystander in the process of acnegenesis. An innocent bystander as far as comedo formation is concerned, sebum is not innocent of all blame. It feeds the commensal organisms and likely acts like hydraulic fluid in transmitting intraductal pressure—more on that later. To be clear, there is a disease in which sebum is trapped under the skin. It is rare, usually runs in families, and has the wonderful name of steatocystoma multiplex. But it is not acne, it is not caused by hormones, and it does not respond to the medications that are effective in treating the acnes, not any one of them, not even isotretinoin. So it is not hair from the pilar area that is plugging the follicular canal—indeed, the hair normally prevents such plugging. And it is not oil from the sebaceous gland plugging up the canal—it is a liquid and doesn’t plug anything. That leaves only one other source of trouble. No matter which acne we are discussing, the source of the problem is the little lining cells that are supposed to simply flake off into the canal and disappear up to the surface. When the ductal keratinocytes don’t flake off, they plug the duct, and they cause acne, whether acne vulgaris, acne rosacea, or acne inversa. So why has this simple and easily observed physical feature not been more widely understood? And why has it not been recognized as the real cause of acne? I think we can blame the “scientific method” in this case. Very early in their investigative lives, scientists learn that they must measure what they wish to study. Thus, for almost 50 years, acne investigators have been measuring what they could measure. They could measure sebum production on the surface, or they could count lesions. There are hundreds of papers written about the skin surface lipids and the amount of oil produced by the FPSU. And there are hundreds of acne papers in which comedones have been counted. Nobody has measured the weight or volume of the keratinous material caught in the lumen of the FPSU. There were some visually impressive studies done with cyanoacrylate adhesives that demonstrated the plugs to a certain degree, but no assay was ever developed to quantify the weight or volume of the comedo or the microcomedo. Anyone who has ever performed acne surgery or comedo extraction knows the challenge of extracting material of a certain diameter through an orifice with a much smaller diameter. With no ability to accurately and reproducibly measure the keratinous content of the duct, there was no technique to measure the effect of various therapies on the quantity of material trapped in the follicular canal. To do so would have required numerous small biopsies of the pilosebaceous units before, during, and after therapies. To measure sebum output, a simpler chore, cigarette paper is applied to the study site, held in place for a defined time period, and then removed and weighed to measure absolute weight and so calculate the rate of sebum production. It is sufficiently difficult to recruit study subjects just to wrap cigarette paper onto their greasy foreheads; doing serial biopsies would be a monumental undertaking. It is no wonder that this area has remained uninvestigated, and so it is no wonder that we have remained in the dark. That means that our only option until now has been to observe, postulate, consider, suggest, review, and reconsider the possible mechanisms involved in the development of these tiny early lesions. But perhaps now, with the availability of confocal microscopy, automated serial cross-sectional photography, accurate marker-driven pore location, and almost instantaneous computerized calculation, a system could be designed to quantify comedonal volume. Four matched cohorts of patients previously cleared with isotretinoin could be provided with a zero-dairy, low-glycemic-load diet as a control and three experimental diets: one would be a full-dairy, low-glycemic-load diet; a second would be a zero-dairy, high-glycemic-load diet; and the third, a full-dairy, low-glycemic-load diet. I offer that suggested protocol to better financed and more technologically capable colleagues than I. But I offer one caveat—an attempt to recruit volunteers into such a study, one that involved introducing dairy to a blinded population of patients cleared with Roaccutane attracted only two recruits in 14 months. So, while we wait for the technical capacity and backing to quantify what we already know, let’s look a little deeper into the pore. Remember the tight PAS + wrapping around the whole FPSU? It fits like a thin, tight, and tough vinyl glove on your hand and fingers. It holds the whole unit firmly together. As the FPSU produces its various products (hair and sebum and ductal keratinocytes), it expands a bit, resistance is created, and just enough pressure is generated to force the contents of the canal, both sebum and keratinocytes, along the path of least resistance toward the surface. If it were not for the glassy membrane and the fibrous root sheath, the whole FPSU would simply expand like the comedones in Favre–Racouchot syndrome. Remember that hair doesn’t need any constrictive pressure to head to the surface; it is growing out on its own, pushed from below by the power of cellular reproduction in the hair papilla and the walls of the pilar unit. The same power of cellular reproduction operates within the follicular unit, but the push is centripetal (toward the center). When the center of the tubular structure is filled up and more cells are added, the intraductal pressure must rise, the intraductal keratinocytes become compressed and folded, the duct expands to the circumferential limit imposed by the glassy membrane and root sheath, and there is “pushback” against the constraints of the follicular walls [19]. The pressures are so great that the keratinocytes can be folded back on themselves, as illustrated (Figure 2.8) years ago using electron microscopy [20]. This is the source of the pressure that eventually weakens, splits, and ruptures those walls. Figure 2.8 The pressure produced within the duct is such that the keratinocytes, unable to separate from each other, are thrown into folds within the strained confines of the follicular duct. That brings us to the very basic question—what leads to the “tipping point” where the ductal keratinocytes stop flaking off and start to form the tiny structure that Plewig called the “follicular filament” [18]? This filament (Figure 1.1a) is the earliest sign of the microscopic keratinocytic plug, just before the formation of the microcomedo. It grows to form the keratinous plug that accumulates within the follicular canal. What starts the life of the plug that slowly grows and expands within the canal until it becomes a force to be reckoned with, a force that can burst that vinyl glove, releasing all that “outside the body” non-self-material into the “inside the body” environment where it causes such trouble? Before we get into the mechanism behind all that, we need to take a further look at the anatomy of the follicular portion of the FPSU as it occurs in acne. So let’s step back a short distance for another look at what we know so far. We have a closed system that has a limit to how much it can expand. We have contents that normally are produced at a steady rate and that can exit the system at the same steady rate, so that there is no real strain on the container’s walls. So what can go wrong to challenge the container’s walls and lead to their rupture? The first problem worth considering is the increase in output of “product.” We know the hair is not part of the problem. Terminal hair is basically a “constant,” passing through the canal, always the same diameter, never getting stuck. Even the tiny vellus hairs, supple and soft as they are, normally reach the surface with little or no difficulty. And we know that sebum simply flows out of the follicular canal onto the skin, producing the oily skin we all know is part of acne vulgaris and acne rosacea. Oily skin is not such a constant feature in AI/HS, but there is a reason for that—more later. So that leaves us with the plug in the follicular canal, the distinguishing feature of all the acnes, even when it is invisible. That leads logically to the next subject, physiology, which gives us an idea of the mechanisms driving the increased amount of material in the follicular canal. The FPSU makes hair and sebum and a lining for the duct to the surface. What could be simpler? That really is all you need to know, and you could stop right here if it were not for the external factors that convert a well-balanced system to a pathological process. In the acnes, the normal process of cell renewal in the follicle is overstimulated to the point that it becomes abnormal and comedonal acne is produced. This initial comedonal stage of acne is non-inflammatory acne. In many pores and many patients, it may never get beyond that stage. But when the process expands to involve the immune systems, the characteristics of inflammatory acne appear. If the cause of the overstimulation is reduced to a normal level, or is eliminated, the pathologic process settles down and the physiologic processes return to normal. If not done in time, the inflammatory epiphenomena take on lives of their own, unless potent therapy and effective secondary prevention are brought to bear. So what drives all this activity, and how does it all work together? Of the three functional units of the FPSU, the pilar unit has the least to do with acne. The area of the body most thickly covered with hair, the scalp, develops true comedones only exceptionally rarely. Each hair is produced on a cyclic schedule, growing under the combined influence of (female) estrogens and (male) androgens. The timing is still a bit of a mystery. The hair starts in the papilla (hair bulb), the deepest part of the pilar unit. It picks up whatever color its owner’s DNA is coded for, from special cells in the papilla and bulb, and then it heads north. Forced upwards from below by new hair cells being continually produced, it is pushed from the pilar unit through the follicular unit on the way to the surface. It starts out in the pilar unit wrapped in two layers called the inner and outer root sheathes, but it separates from them at the top of the pilar unit. At that point, the now-independent hair shaft acquires a thin and ever-renewing coat of protective and lubricating sebum as sebocytes dissolve around it before the hair heads to the outside world. The sebum that layers onto the hair is produced by the sebaceous glands (Figure 2.9) that are attached to the lower end of the follicular unit at the sebofollicular junction. These glands are narrow-necked structures that look like sacks full of dozens of boring, mostly round, but tightly packed thin-walled cells that are almost all fat-laden cytoplasm with only a tiny nucleus. These sebocytes start life as basal cells inside a protective sack of the same basement membrane material that is wrapped around the other two parts of the FPSU. Figure 2.9 This sebaceous gland is cut in cross-section in an area away from the sebofollicular junction and shows a healthy periodic acid–Schiff positive (PAS+) support structure and a minimal population of tissue-resident immunocytes. The basal cells are stimulated into action mainly by androgens, the male hormones, but other hormones and growth factors are all part of the push to grow. When conditions are right (and nobody really knows for sure what the trigger is), a basal cell will divide into two daughter cells. One will stay behind as a basal cell, and the other (now a sebocyte) will be pushed into the body of the gland. There, the sebocytes grow, fill with various lipids (fats, waxes, and oils), and are gradually pushed up to the neck of the gland, near the hair and the follicle. They head toward the ductus seboglandularis (sebaceous gland duct). The sebaceous cells are actually pushed toward the exit like people leaving a theatre, jostling for the next free space, but each maintains its walls intact until it actually reaches the “door.” As soon as it gets through the sebaceous duct, up against the hair, the sebocyte’s wall dissolves, and the oil is released onto the hair in the follicular unit. Even on a microscopic level, there is no “pool” of sebum in the sebaceous duct or anywhere under the skin. The sebocyte remains intact until it actually reaches the hair it is meant to lubricate (Figure 2.10). Figure 2.10 The sebaceous unit plugging into the isthmus of the pilofollicular structure. Note there is no free sebum pool—the sebocytes dissolve only upon contact with the hair. Note the inflammation concentrated where minimal periodic acid–Schiff positive (PAS+) material is present at 6–6:30 and 7–9 o’clock and one or two inflammatory cells at 3 o’clock. Much of the best research in acne has been directed at figuring out what hormone and enzyme systems provide the push to the basal cells to make them produce either many or few daughter cells (sebocytes), and so regulate the oiliness on the skin surface. This complex subject is coming up in the next couple of sections. The same hormones that push the hair up from the pilar unit and push the sebocytes up to the junction of the follicular unit, where they can burst and lubricate the hair shaft, also have serious work to do in the follicular unit. In fact, they are the cause, and this is the location, of the first sign of trouble in all the acnes. As the hormones turn on the basal cells that lie just inside the glassy membrane of the follicular unit, daughter cells are again produced, but this time they turn into keratinocytes instead of sebocytes. And instead of heading randomly to the exit, they normally follow a very strict and formal path toward the center of the follicular duct. When all is going according to plan, each keratinocyte gradually matures from a cuboidal cell to a gradually flattened cell and eventually becomes pancake-shaped as the center of the duct is approached. Internal chemical processes gradually use up the energy provided by the fats and complex sugars. This energy fuels the formation of special proteins that make keratin for the keratinocyte and also forms the protein and complex lipid and sugar junctions between the cells, all needed to complete the maturation and terminal differentiation of the keratinocytes. Residual energy sources then produce proteases that dissolve the junctions between the fully differentiated keratinocytes until they are fully separated into squames. These are the residual flat flakes of keratin that normally separate from each other, flake off into the central duct, and mix with the sebum. Suitably lubricated, they slide up to the surface in the space between the follicular wall and the hair shaft, if there is one. That is how it is supposed to work, and that is how it does work until too much stimulus is added to the system. The chemical processes that run in the background, making the physiology work, run through highly complex biochemical reactions that produce equally complex and varied products. Each of the basal keratinocytes and basal hair root cells and basal sebocytes uses its own specialized set of enzymatic reactions, chosen from the huge set of possible reactions the cellular genome can produce on demand. Just think for a moment—back to the embryology section (Section 2.4). Those few fetal cells that made the buds that formed on the underside of the fetal skin can go on to produce a full beard, or a greasy scalp, or a face and back full of inflammatory acne, or a perineum full of draining acne inversa lesions, or Farrah Fawcett’s glorious mane of hair. DNA and hormones are a potent combination. The FPSU produces cells that line the follicle, the hair, the pigments that color the hair, the multiple components of sebum, the cell walls of sebocytes, and the enzymes that run the show. These products are made up of fats, proteins, and carbohydrates, but the expression and organization of each vary tremendously from tissue to tissue. Let’s consider fats first. Fats can be used for energy, or as a structural material, or they may simply be exported, as sebum. For example, intracellular fat in keratinocytes is used up over their life span to power their metabolic processes. If the terminal differentiation process that leads to keratinocyte maturity is incomplete, lipid is left over unused and shows up under the electron microscope as pooled lipid droplets in layers of keratinocytes (Figure 2.11), demonstrating arrested maturity [20]. Other fats are combined with proteins to form complex combinations called lipoproteins. These larger molecules are used in cell wall construction and for the creation of the tiny intracellular organelles that are the factories and energy management systems of the cells. The fats that are created for export in the sebocytes are a fairly limited group. They are triglycerides, cholesterol, fatty acids, and squalene. Each has a role to play in acne, but their prime role is lubrication during birth. Their second role is protection of the human skin to prevent its drying out, simply by sealing water into the skin and thereby preventing evaporation from the skin surface. Figure 2.11 The failure of the keratinocytes to achieve terminal differentiation, likely due to anoxia and mediated by hypoxia-inducible factor 1 (HIF-1), leaves behind the lipids that would have been used as structural lipids or as a source of energy to fuel the differentiation. Proteins come in three sizes, small (peptides with up to 10 amino acids), medium (polypeptides with up to 50 amino acids), and large (proteins with over 50 amino acids). The peptides are usually building blocks for larger proteins. The polypeptides have numerous uses in acne, including a large number of hormones and growth factors. A fairly short example, FoxO1, is the polypeptide nuclear transcription factor that is the prime regulator of the androgen receptor that controls acne [21]. Proteins can be long and strong so they find their main use as structural components like the collagen fibers that wrap around the FPSU to give it strength. They also can be incredibly complex, bent into all sorts of shapes so they can form the flexible, electrically charged, amorphous mass that enzymes are made from. That leaves the carbohydrates, which generally serve as the prime energy source for the FPSU but also form parts of various structures. One such structural material that is important in all the acnes is glycoprotein (glucose is the carbohydrate sugar that is combined with the protein). This is part of the material that makes up the glassy membrane; indeed, the sugar part is what makes the PAS + material stain pink under the microscope [22]. This fibrous web supports the whole FPSU structure, giving it the strength and shape needed to support and anchor hair. It also provides the support that puts the squeeze on sebaceous glands and sebum to keep the oil flowing, and provides support to the follicular tube that takes both hair and sebum to the skin surface. As elsewhere in nature, “carbs” may be either essential for health in normal amounts, or a cause of disease or disorder in either deficiency or excess. The FPSU, left to itself, would not produce acne. That is the situation in families with congenital absence of 5α-reductase, and also in the Aché and Trobriand tribes whose diets are free of dairy and high-glycemic-load foods. So, where do the hormones come from that cause all this trouble? And why, if these are normal hormones, should we not expect acne as a normal event in everyone during puberty and post-adolescence? And why does acne normally fade when maturity arrives? Acne is turned on by a cascade of chemical changes that are driven by a host of hormones. Hormones are basically chemical messengers. They are attracted to receptors on the surfaces of enzymes and are modified in various ways while attached to the enzyme receptor, and then these modified (and often more potent) hormones may travel to other enzyme receptors for additional modification. Eventually they travel to the androgen receptor, where they turn on the processes that lead to acne. The production of dihydrotestosterone (DHT), the potent androgen that drives acne, starts with simple cholesterol, the essential building block of all our steroid-based hormones. Much maligned, cholesterol is the building block for many essential body processes. Our nervous system would not function without it. Its contribution to the reproductive hormones’ structure allows us to grow and mature into reproductive human beings. Without cholesterol, we would have evolved in a different direction, if at all. Through a series of stepwise changes supervised and facilitated by a limited number of enzymes, the cholesterol molecule is neatly converted to pregnenolone (top left) and then to DHT, the most potent androgen and so the most potent acnegen. In the diagram of this cascade (Figure 2.12), the two steps from left to right are the responsibility of 3β-hydroxysteroid dehydrogenase (plus an isomerase) and 5α-reductase; the three steps from top to bottom are mediated by 17α-hydroxylase, 17α-lyase, and 17β-hydroxysteroid dehydrogenase. These are responsible, during the production of cow milk, for the presence of several 5α-reduced molecules that act as precursors of DHT (Section 4.2.4). They are not shown here. Figure 2.12 The intracrine system utilizes five onsite enzymes to produce the range of androgens needed in and around the folliculopilosebaceous unit and elsewhere in the body. Some may be made directly from cholesterol; others may utilize the androgen precursors in dairy products. The hormones that drive acne are complex, and they come from different sources. Those from inside the body (from the ovaries, testes, and adrenal glands) are called endogenous (Section 4.1). Those from outside of the body are called exogenous (Section 4.2). Others are produced within the FPSU itself by the intracrine system (see Section 2.8.1). The exogenous hormones we generally hear about are the “steroid” hormones given to athletes. These are both human and animal hormones, and are used in both human and animal athletes. These are the anabolic steroids used to build muscle, strength, endurance, and speed. There are many of them, some natural and some semisynthetic. They may be used singly, or several may be “stacked” together to achieve the results the user desires. The normal human body does much the same with its hormones, but operates with a different and totally natural “stack” to create and grow everything from babies to beards. But there is another whole world of exogenous hormones out there. Some are present in nature, like those in cow milk, which at least are natural in cows. Some are artificial or manmade (like birth control pills). Some of the chemicals we are exposed to are not really hormones but are environmental pollutants that can mimic the effect of hormones in our bodies. They have the potential to act as hormonal disruptors, another long story. All these chemicals have one thing in common—they are all “keys” to the lock in the androgen (and other) receptors. It is important to realize that the keys to the lock are quite varied and have quite varied effects. Imagine a key that fits the keyhole loosely and falls out easily. On the other end, imagine a key that locks into the keyhole permanently and cannot be removed. How about a key that fits lots of different keyholes and can open the door in most of them? Like a master key that can work in many different apartment doors, it has a much broader range of effects. Then consider that some of the loose keys can be pulled away by carrier proteins like sex hormone–binding globulin (SHBG), leaving the keyhole open for another competing hormone that has a greater affinity (a stronger pull) to the keyhole. That keyhole, the one that holds the hormone tighter, is said to have a greater avidity. The tighter fitting hormones will stay in the keyhole longer, sometimes forever. Then go one step further and consider that even if the key fits in the keyhole, it sometimes will not turn in the lock. This is the way car keys used to be—you could get in the door with the door key, but even if it fit in the ignition keyhole it wouldn’t turn on the ignition. Chemicals with this sort of “chemical look-alike” pattern are used as hormone blockers. This presents us with a tremendous variation in the effects that various hormones (the keys) have on various tissues. Not only does each hormone sometimes fit in more than one kind of receptor, but it may have effects on that receptor that will vary from antagonism (reversing or blocking the normal effect of the hormone) to neutral (essentially doing nothing, like a key that fits but cannot be turned) to agonism (an agonist is something that positively makes things happen) so the hormone does what it was designed to do. Each action will vary, so, for example, you will see the term weak agonist, which means it works, but is not a strong influence. DHT is a 5–10 times stronger agonist in the androgen receptor controlling an acnegenic enzymatic process than is testosterone (T), a weaker agonist. This process and the whole subject of hormones and receptors would be simple if T and DHT were the only hormones that worked in the androgen receptor, but overlapping functions confuse things. Some receptors will accept a variety of hormones (and other matching chemicals), and some hormones can activate a variety of receptors. Receptors will also bind things that they were never intended to bind. That includes toxic substances such as dioxins (see Section 5.1) but also some of the drugs we use. To complicate matters further, steroid hormones have the ability to do all sorts of different things, at quite different locations, all related to their attraction to the receptors, the “fit” with the receptors, the “avidity” of the receptors (how hard they hold onto the hormone), the number of competing hormones looking for receptor sites, and other variables. The last variable includes the process regulated by the individual receptor in the individual cell. For example, dermatologists regularly use spironolactone, a look-alike chemical that blocks androgen receptors and acts as a “competitive inhibitor.” It is great for slowing down the plugging of pores and so improving acne in women, but it can also stop or slow hair loss on women’s scalps, decrease the amount of unwanted hair on women’s faces and bodies, cause breakthrough menstrual bleeding, minimize acne rosacea, reduce leg swelling and lower blood pressure.
The folliculopilosebaceous unit—the normal FPSU
2.1 Anatomy
2.2 Genetics
2.2.1 Acne vulgaris
2.2.2 Acne rosacea
2.2.3 Acne inversa/hidradenitis suppurativa (AI/HS)
2.2.4 The scottish twins
2.3 Epigenetics
2.3.1 The farmer’s boys
2.4 Embryology
2.5 Histology
2.5.1 Onwards and downwards
2.5.2 What is going on inside the FPSU?
2.6 Physiology
2.6.1 Hair first
2.6.2 Oil second
2.6.3 Last but definitely not least: the follicle
2.6.4 Looking deeperspan>
2.7 Biochemistry
2.8 Hormones, enzymes, receptors, and the intracrine system
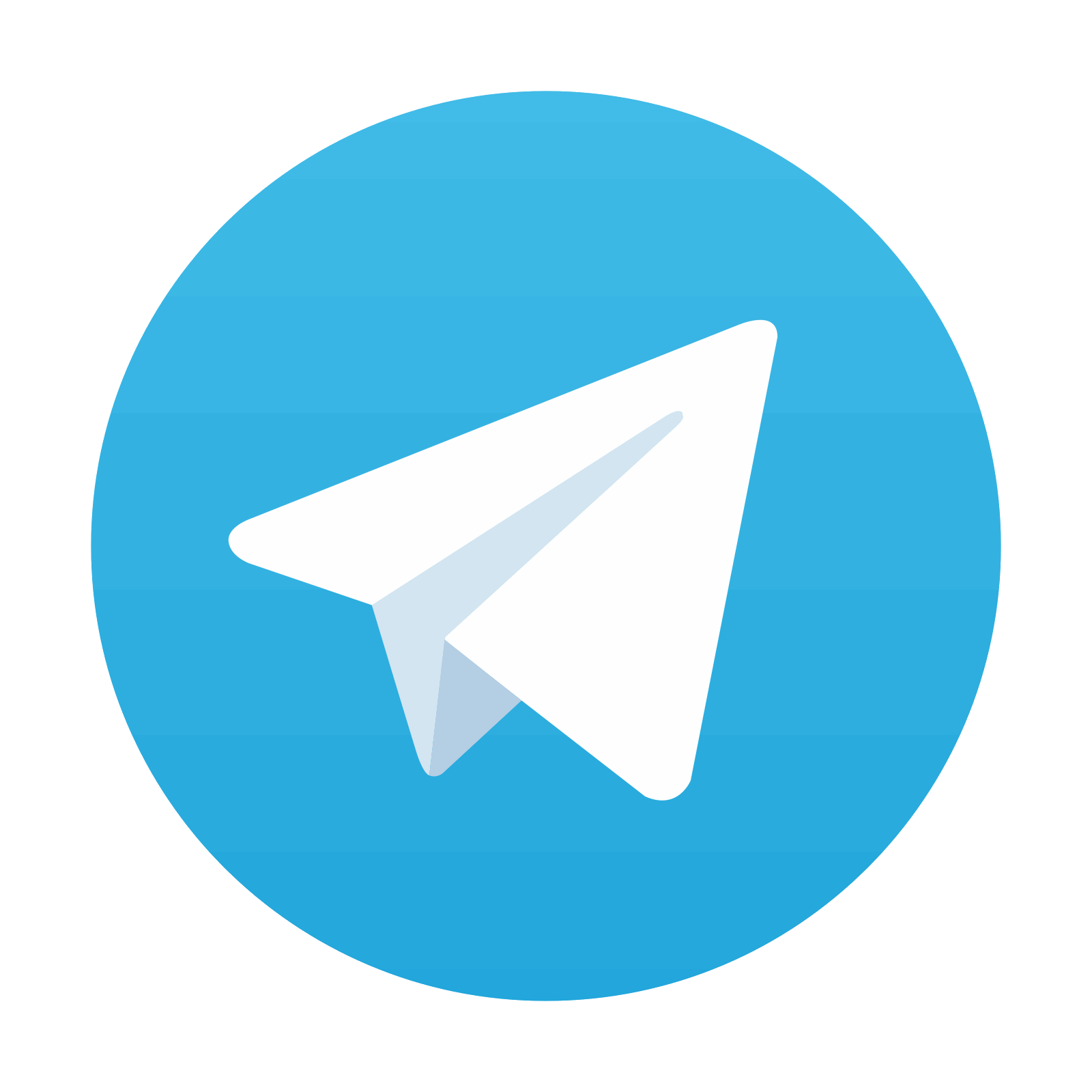