Skin Flap Physiology
Physiologic Characteristics of Skin
The blood supply to the skin serves two important functions. It provides nutritional support and a thermoregulatory mechanism for the body. Primarily because of its thermoregulatory function, the rate of blood flow through the skin is one of the most variable in the body. Under ordinary skin temperatures, the amount of blood flowing through the skin (0.25 L/m2 of body surface area) is approximately 10 times the flow required for nutritional support.1 Blood flow can increase up to seven times this value with maximal vasodilation. When the body is exposed to extreme cold, blood flow can be reduced to levels that are marginal for cutaneous nutrition.
The nutrient capillary network in the reticular dermis and the arteriovenous shunts in the more superficial papillary dermis2 perform the two functions of the cutaneous circulation. The amount of blood flow to the skin depends ultimately on arteriolar pressure and flow. Under conditions of adequate systemic vascular pressure, however, arterioles act as preshunt and precapillary sphincters that regulate the flow through each vascular network.3
The sphincters in the two vascular systems respond to different stimuli (Fig. 2-1). The precapillary sphincter, which controls the amount of nutritive4 blood flow to the skin, responds to local hypoxemia and increased metabolic byproducts by dilation.5 Under these conditions, the blood flow is increased (reactive hyperemia being an example). The preshunt sphincters are involved in regulating the changes in blood flow that affect thermoregulation and systemic blood pressure.6 Release of norepinephrine by the postganglionic sympathetic fibers results in contraction of the preshunt sphincters. This diverts blood away from the skin surface, where heat loss can occur. With increased body temperature, the sympathetic vasoconstrictor impulses decrease, allowing increased blood flow to the skin.4
FIGURE 2-1 Precapillary () and preshunt (
) sphincters in skin. The precapillary sphincter regulates nutritive blood flow to skin and responds to locally produced stimuli. The preshunt sphincter is involved in thermoregulation and is affected by sympathetic stimuli from the central nervous system.
Neurovascular Supply to Local Skin Flaps
Blood vessels travel by one of two main routes to terminate in the cutaneous circulation. Musculocutaneous arteries pass through the overlying muscle to which they provide nutrition; septocutaneous arteries (also referred to as direct cutaneous arteries) travel through fascial septa, which divide the muscle segments (Fig. 2-2).
FIGURE 2-2 Depiction of varying pathways to skin that defines musculocutaneous (MC) and septocutaneous (SC) arteries.
The neural supply to the skin originates from both sensory nerves and sympathetic nerves. The sensory nerves are distributed in segmental fashion, forming dermatomes, and participate in the skin’s protective function. The postganglionic terminals of cutaneous sympathetic nerves contain the neurotransmitter norepinephrine and are found in the area of cutaneous arterioles.4
Vascular Design of Common Local Skin Flaps in the Head and Neck
The vascular supply to a flap is critical to its survival and is the basis of one classification system of flaps (Fig. 2-3). Local flaps in the head and neck are primarily random or arterial cutaneous.
FIGURE 2-3 Classification of skin flaps based on vascular supply. A, Random. B, Arterial cutaneous. C, Fasciocutaneous. D, Musculocutaneous.
Random Cutaneous Flaps
Length-to-width ratios of random cutaneous flaps have been recommended for various areas of the body. These differences reflect a regional variation of the neurovascular supply to the skin. Such a description can serve as a guide in designing random cutaneous flaps but should not imply that a wider flap would extend survival length.7
Arterial Cutaneous Flaps
Arterial cutaneous flaps (also called axial pattern flaps and perforator flaps) typically have an improved survival length relative to random cutaneous flaps.8 This advantage results from the incorporation of a septocutaneous artery within the longitudinal axis. An island flap is an arterial flap with a pedicle consisting of nutrient vessels without the overlying skin. Island flaps can be useful to increase flexibility and to reduce pedicle bulk in certain reconstructive procedures.
The surviving length of arterial flaps is related to length of the included septocutaneous artery. Survival beyond the arterial portion of the flap is based on the subdermal plexus and is essentially a random cutaneous extension of the flap. Flap necrosis secondary to ischemia can be said to occur only in the random portion of the flap (destruction of the arterial pedicle making the entire flap random). Preoperative imaging with computed tomographic angiography to evaluate perforator distribution9 as well as the subcutaneous arterial pattern10 can further increase the usefulness of these flaps.
Physiologic Changes after Skin Flap Elevation
Impairment of Vascular Supply
Partial interruption of the vascular supply to the skin is the most obvious and critical change that occurs with elevation of a cutaneous flap. This interruption results in a local decrease in perfusion pressure to the skin. In arterial or myocutaneous flaps, the blood supply to the skin overlying the vascular pedicle is usually adequate. In random flaps or random extensions of flaps, the decrease in perfusion pressure becomes more pronounced with increasing distance from the base of the flap.11 When perfusion is reduced in one area of a random flap, the adjacent vascular territories supplied by a separate perforating vessel can provide a low-pressure blood supply through the subdermal plexus (Fig. 2-4). Because the nutritional requirements of skin are relatively low, a number of vascular territories can be compromised before necrosis will result.
FIGURE 2-4 Vascular territories in skin flap. Multiple perforating vessels exist and are interconnected at the periphery of their vascular territory. When vessels are cut, blood supply can be replaced from nearby perforating vessels and tissue necrosis does not occur.
The survival length of the random portion of the flap depends on the physical properties of the supplying vessels (intravascular resistance) and the perfusion pressure.11 When the perfusion pressure drops below the critical closing pressure of the arterioles in the subdermal plexus, nutritional blood flow ceases and flap necrosis occurs. In the past, random cutaneous flaps were often designed relative to a desired length-to-width ratio, a wider base being needed to successfully transfer a longer flap. The wider random flap includes only additional vessels with the same perfusion pressure. The relationship between perfusion pressure and critical closing pressure is not altered, and no change in survival length occurs7 (Fig. 2-5).
FIGURE 2-5 Fallacy of length-to-width ratio. Slope of decreasing perfusion pressure versus flap length does not change with incorporation of additional vessels (flap A versus flap B) with same perfusion pressure. Flap necrosis occurs when perfusion pressure falls below critical closing pressure of capillary bed.
Myers has emphasized that “fresh flaps are always both viable and ischemic.”12 Depending on the degree of ischemia and the amount of time before recovery of nutrient blood flow, the flap will either die or recover. In the pig model, arterial and random flaps can tolerate an average of 13 hours of total avascularity and remain viable.13 In flaps with reduced perfusion, this time is probably much longer.
In surviving flaps, blood flow gradually increases. If the flap is in a favorable recipient site, a fibrin layer forms within the first 2 days. Neovascularization of the flap begins 3 to 7 days after flap transposition. Early neovascularization has been detected at 4 days in the pig and rabbit models14 and at 3 days in the rat model.15 Revascularization adequate for division of the flap pedicle has been demonstrated by 7 days in animal models and humans.14,16
Beginning with an angiogenic stimulus, the angiogenic process involves a number of discrete yet overlying steps (Fig. 2-6). Initially, the vessels become dilated and permeable with retraction of the endothelial cells and a decrease in endothelial junctions. The basement membrane is then dissolved by proteases, and the endothelial cells migrate from the vascular wall toward the angiogenic stimulus. Behind the leading front of migrating endothelial cells, endothelial cell replication begins, forming a capillary sprout that elongates toward the angiogenic source. The nearby capillary sprouts then anastomose to each other, forming capillary loops. As capillary loops and sprouts continue, the loops become patent, forming newly formed blood vessels. These blood vessels differentiate and lay down basement membrane consisting of type IV collagen, laminin, and proteoglycans. Pericytes and fibroblasts then migrate to the capillary loop sites.17
FIGURE 2-6 Steps in angiogenesis. A, Initial stimulus with retraction of endothelial cells and thinning of basement membrane. B, Migration of endothelial cells and formation of capillary sprout. C, Formation of capillary loops, which become patent to form new blood vessels.
With the continued presence or absence of the angiogenic stimulus, substantial remodeling, regression, and rearrangement of the new capillaries occur.17 Some capillaries join preexisting flap vessels (inosculation), but the majority of revascularization appears to involve direct ingrowth of recipient vessels into the flap18 (Fig. 2-7). New capillaries can grow toward an angiogenic source at a mean rate of 0.2 mm/day. When the angiogenic stimulus is discontinued, the capillary vessels regress and eventually disappear during a period of weeks. Angiogenic growth factors can stimulate capillary growth over distances of 2 to 5 mm.19
To prevent an uncontrollable cascade of neovascularization, mechanisms to inhibit angiogenesis are believed to exist. Evidence suggests that pericytes can suppress endothelial growth by direct contact.20 Thus, the physiologic response of angiogenesis may be analogous to the blood coagulation pathway that must be maintained at a constant steady state of control. In soft tissue wound repair, macrophages, lymphocytes, mast cells, and platelets are involved in releasing various factors that modulate angiogenesis.
The venous outflow from the skin is also impaired with flap elevation. Venous flow can occur through the subdermal plexus or by venous channels that accompany the feeding artery in the pedicle. Complete venous occlusion in the early period after elevation may be more damaging to flap survival than inadequate arterial supply.21 Fortunately, the subdermal plexus alone is often adequate to provide sufficient venous outflow. Care must be taken, however, to preserve venous outflow in flaps pedicled solely on the feeding vessels.
Nerve Section
Both cutaneous and sympathetic nerves are severed in the process of flap elevation. Denervation of a skin flap postpones neovascularization of the skin flap. Whereas loss of sensation may limit the usefulness of the flap after transfer, adrenergic denervation has implications for flap survival. When a sympathetic nerve is divided, catecholamines are released from the nerve terminal and the mechanism for catecholamine reuptake is eliminated.22 A local “hyperadrenergic state” develops that produces vasoconstriction mediated by alpha-adrenergic receptors in the cutaneous vasculature. In addition, severe sympathetic denervation contributes to the production of oxygen free radicals, which may exert their inhibitory effects on neovascularization.23
The vasoconstricting effect of sympathectomy further reduces the total flap blood flow24 that has already been diminished by division of supplying vessels. This negatively affects the ratio of perfusion pressure to the critical closing pressure of the arterioles in the subdermal plexus. A greater proportion of the distal flap is excluded from the blood supply and necrosis becomes more likely. The stored transmitter is depleted within 24 to 48 hours,25 and blood flow increases as the concentration of norepinephrine declines. In critical areas of the flap, however, the time to recovery of nutrient blood flow may be delayed sufficiently to produce additional necrosis.
Inflammation and Prostaglandins
The surgical trauma associated with an acutely raised flap results in an inflammatory response. Histamine, serotonin, and kinins are released into the extracellular compartment after flap elevation, increasing the permeability of the microcirculation. The result is an increase in the concentration of proteins and cells within the extracellular space. The presence of nonbacterial inflammation beginning a few days before flap elevation has been shown to improve flap survival.26,27 This is presumably the result of an increase in local blood flow. The inflammation created during flap elevation, however, may have deleterious effects due to the resultant edema formation.
Prostaglandins are derived from essential fatty acids that are incorporated in membrane phospholipids (Fig. 2-8). Activation of phospholipases results in the production of prostaglandin H2 (PGH2) by cyclooxygenase. Prostaglandin E1 (PGE1) and prostaglandin E2 (PGE2) can be synthesized from PGH2 by isomerases in the vascular endothelium. Both PGE1 and PGE2 produce vasodilation. Prostaglandin D2 (PGD2) is also formed by an isomerase reaction and is the principal cyclooxygenase product of the mast cell. Its effects on the cutaneous microvasculature are similar to those of PGE1. Prostacyclin (PGI2) is a vasodilating agent and inhibitor of platelet aggregation that is derived from PGH2 through the action of prostacyclin synthase. In the skin, PGI2 is primarily produced in the endothelial cells of blood vessels.1,28
FIGURE 2-8 Synthesis of prostaglandins and thromboxanes and their general effects in cutaneous circulation.
Thromboxane synthetase converts PGH2 into thromboxane A2 (TxA2) and is primarily located in the platelets. Its effects include vessel constriction and promotion of platelet aggregation. Prostaglandin F2α (PGF2α) is derived from PGH2 by a reductase reaction. A marked increase in resistance is seen in cutaneous arteries, arterioles, and venules in the presence of PGF2α.29
The synthesis of prostaglandins and thromboxane can be altered by pharmacologic manipulation. The action of phospholipase A2 can be inhibited by drugs that reduce the availability of calcium. Glucocorticoids also affect phospholipase A2 activity by inducing the synthesis of a protein that inhibits the enzyme.30 Aspirin and other nonsteroidal anti-inflammatory medications interfere with the cyclooxygenase enzyme, inhibiting the synthesis of PGH2.
Prostacyclin levels were found to peak 7 days after elevation of a porcine flank flap.1 Elevation of a bipedicled rat dorsal flap resulted in elevated levels of PGE2, PGF2α, and TxB2, with a return to nearly normal levels by day 7. Conversion to a single pedicle flap (“delay”) resulted in a blunted production of thromboxane and an elevated PGE2 level. Elevation of an acute flap in the same model showed an elevation of PGE2, PGF2α, and TxB2 that was greater and more prolonged than with surgical delay.31 Prostaglandins clearly play a role in the inflammatory response after flap surgery. Whether these changes in prostaglandin levels represent a cause or a side effect of the observed phenomenon remains to be demonstrated.
Reperfusion (Free Radicals)
Return of blood flow to a flap that is ischemic as a result of excessive release of norepinephrine occurs in approximately 12 hours. With norepinephrine depletion and continued inflammatory response, blood flow can reach a maximum at 24 hours.24 When oxygen becomes available with reperfusion, an additional menace to flap survival is produced, the free radical. This byproduct of reperfusion can cause damage at both the cellular and subcellular levels,32 contributing to postischemic tissue necrosis.
Free radicals are extremely reactive compounds because of an unpaired electron in their outer orbitals. Oxygen free radicals are formed by the sequential univalent reduction of molecular oxygen. The superoxide anion radical (O2−) is formed by the addition of a single electron to molecular oxygen. Superoxide is a byproduct of adenosine triphosphate production in the mitochondria and other oxidation reduction reactions.32 Polymorphonuclear cells are a second source of superoxide radicals that are released in response to bacterial inflammation.
A major source of free radicals in ischemic tissue is the enzyme xanthine oxidase33 (Fig. 2-9). With ischemia, high-energy phosphate compounds are converted to hypoxanthine that accumulates in the tissues. When oxygen becomes available with reperfusion, xanthine oxidase catalyzes the conversion of hypoxanthine into uric acid, producing superoxide in the process. This reaction is an important mechanism in ischemic tissue injury in skin flaps.34
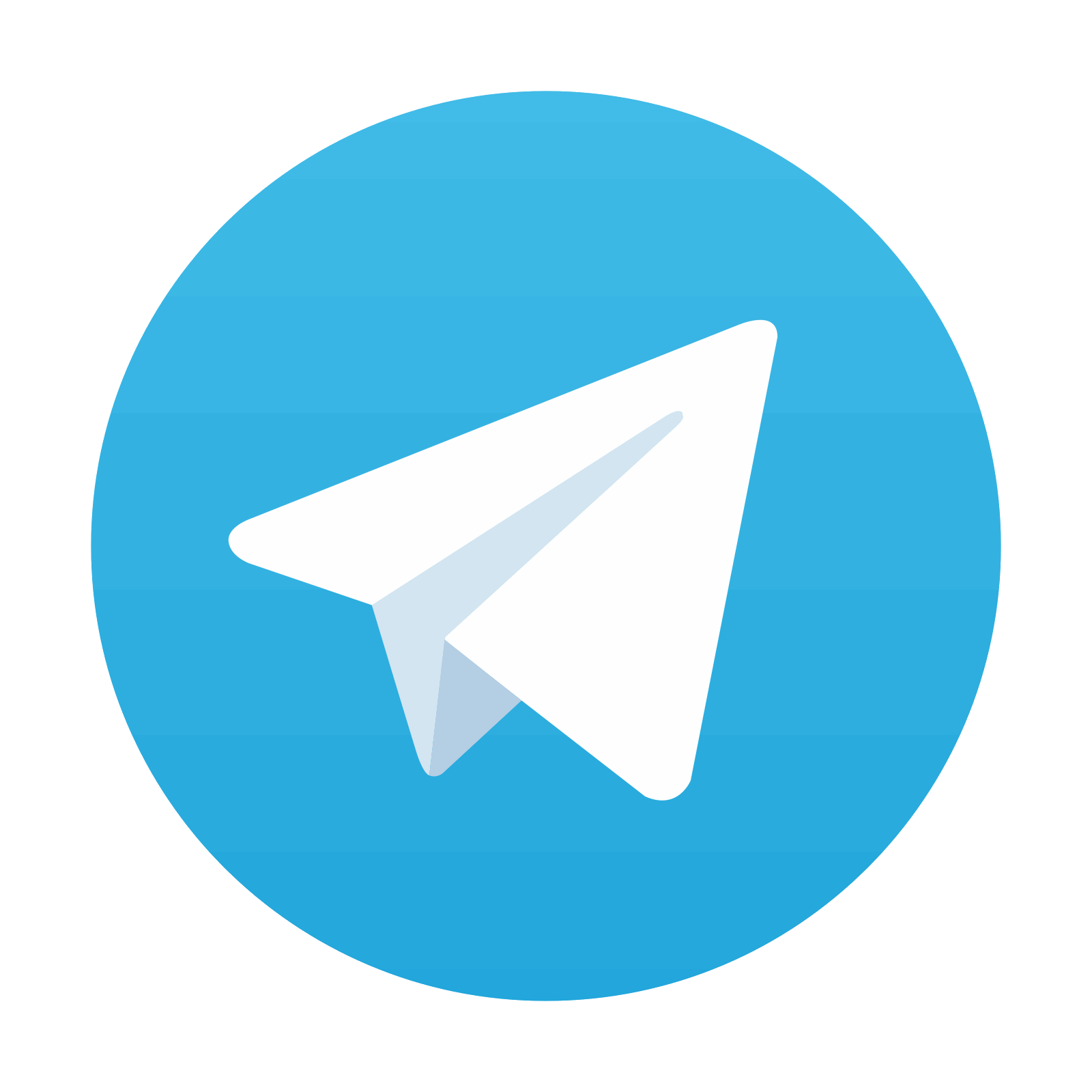
Stay updated, free articles. Join our Telegram channel

Full access? Get Clinical Tree
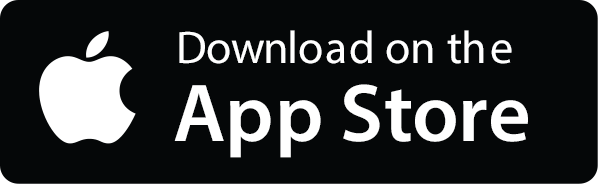
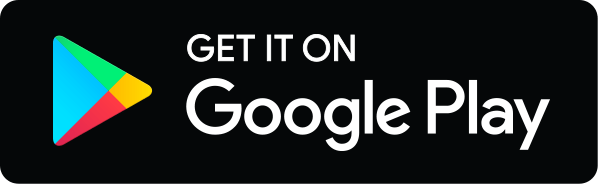