3
Radiofrequency Technology
Thermal treatment using optical energy for various types of dermatologic problems has become very popular over the past 20 years. Light-based therapies with lasers and intense pulsed light (IPL) technologies have been increasingly used in aesthetic medicine for epilation, removal of vascular and pigmented lesions, reduction of fine wrinkles, and treatment of acne.1 The fundamental principle behind the use of light-based therapies is based on the theory of selective photothermolysis, which encompasses the following three tenets2,3:
- Optical energy penetrates deep enough to reach the treated target.
- Optical energy is mostly absorbed by the target, although surrounding skin may be heated significantly.
- Optical energy is strong enough to create thermal damage of the treated target.
In selective photothermolysis, a pulse of light set at the proper wavelength and proper duration is delivered to targeted sites. Although effective for a broad range of dermatologic indications, limitations have also been realized with light-based therapies.4 One of the main limitations is that optical energy must penetrate the epidermis to reach the depth of the targeted site. Optical energy is absorbed by melanin chromophores in the epidermis and hair shafts, and hemoglobin in blood. In photoepilation, light-colored hair is particularly difficult to remove because it has low levels of melanin and therefore may not absorb enough energy to achieve thermal destruction of the hair follicle. Conversely, high pigmentation of the epidermis also poses a problem because it may absorb too much energy, potentially causing adverse effects such as burns and hyperpigmentation. Wrinkles respond poorly to treatment with optical energy because collagen fibers do not contain chromophores. These limitations have stimulated investigators to look for new forms of energy that satisfy the principle of selective thermolysis but are devoid of the main disadvantage of optical energy for dermatologic applications; that is, a strong interdependence between treatment efficacy/safety and chromophore levels in the epidermis.
This chapter evaluates the use of an alternative source of energy—electrical current—for the selective treatment of different biological targets. Different from selective photothermolysis, the treatment using electrical current is termed selective electrothermolysis.
Electrical Current in Medicine
Electrical current has been used in medicine for more than a century. Low frequency or direct current (DC) causes spasms of the muscles and is used at low intensity for biostimulation, such as cardioversion of atrial fibrillation.5 High frequency current in the range of 0.3 to 10 megahertz (MHz), or radiofrequency (RF) current, produces a pure thermal effect on biological tissue that is dependent on the electrical properties of the tissue. The high efficiency of RF current for tissue heating has made it useful for electrosurgery and an attractive source of energy for various dermatologic applications.6,7 The mechanism of tissue heating is based on generating Joules of heat by electrical current. Generated heat is described by Joule’s law:
H = j2/σ
where j is the density of electrical current and a-is electrical conductivity.8 The value opposite to conductivity is named resistance or impedance (ρ). Distribution of electrical current can be calculated using the method described in the color section that follows.
Electrical conductivity depends on the frequency of the electrical current and the type and temperature of the tissue. The distribution of electrical current depends on the geometry of the electrodes. Two typical configurations are used in medicine: unipolar and bipolar. The major difference between the two systems is how the RF current is controlled and directed at the target. However, there is no difference in ultimate tissue effect at the same RF fluence.
Method for Calculating Distribution of Electrical Current a
Distribution of electrical current is described by a continuity equation
div j = 0 (A1)
where j is density of the electrical current. The equation states that electrical current starts in one of the electrodes and has to reach the other electrode closing the electrical loop through the electrical circuit.
According to the Ohm equation the current density is higher when conductivity and electrical field are higher:
j = σ E (A2)
where σ is electrical conductivity of tissue and E is electrical field strength, which is described by following equation:
E = −grad φ (A3)
where φ is a potential of the electrical field.
Combining Equations (A1) through (A3) the following equation for potential of the electrical field can be obtained:
div (σgrad φ) = 0. (A4)
Electrical conductivity depends on frequency of the electrical current and the type of tissue and its temperature. For analyzing electrical current distribution Equation A4 is solved using numerical computerization with boundary conditions depending on geometry of electrodes.
Unipolar System
A unipolar or monopolar system delivers energy through one electrode with a relatively small contact point applied to the treatment area while another large-size ground electrode is applied to the body at a distance far from the active electrode. A cylindrical electrode is applied to the skin surface, and the schematic flow of electrical current is depicted in Fig. 3-1. Electrical energy is concentrated near the tip of the electrode and decreases rapidly with distance. Penetration depth of RF current can be estimated as half the electrode size. Therefore, a 10 mm unipolar electrode has a penetration depth of ∼5 mm, which is deep enough to reach muscles.
Figure 3-1 Schematic representation of the flow of electrical current through the epidermis using a cylindrical unipolar electrode.
The advantage of a unipolar system is its ability to create a high-power density on the surface of the electrode. This attribute makes it popular in electrosurgery where a small-size electrode is used. The disadvantage of a unipolar system is its unpredictable behavior as the current passes through the body to the grounding electrode. In aesthetic medicine, examples of a unipolar system include the Visage (ArthroCare Corp., Sunnyvale, California) and ThermaCool (Thermage Inc., Hayward, California).
Bipolar System
A bipolar system passes an electrical current between two electrodes at a fixed distance. Both electrodes are applied to the treated area, and electrical current propagation is limited by the area between electrodes. The behavior of electrical current in a bipolar system is depicted in Fig. 3-2. Distribution of electrical current as a function of depth (calculated using the method described in the Equations section later in this chapter) for different distances between electrodes is presented in Fig. 3-3. Using the same definition of penetration depth as used for the electromagnetic radiation and particularly the depth where density of value is decreased by factor e = 2.72. The penetration depth of electrical current can be estimated as half the distance between electrodes.
Figure 3-2 Schematic representation of the flow of electrical current through the epidermis using a bipolar system.
The main advantage of a bipolar system is the controlled distribution of RF current inside the tissue, which is limited by volume between the two electrodes. Therefore, the bipolar system is less suitable for electro-surgery but appropriate for hemostasis and controlled vessel contraction using an intravascular catheter. In aesthetic medicine, examples of bipolar systems include the Aurora™ and Polaris™ systems (Syneron Medical Ltd.; Yokneam, Israel).
Electrical Conductivity of Different Tissue Types
Table 3-1 shows the electrical conductivity of different tissues at normal temperature and a frequency of electrical current at 1 MHz. Blood and parts of the body with high blood content have the highest electrical conductivity. Bone has very low electrical conductivity, and therefore, electrical current does not penetrate the bone but rather flows around it. Dry skin is also resistant to electrical current and must be hydrated to permit passage of electrical current into the tissue. Conductivity of tissue increases proportionally with frequency. In Fig. 3-4, the RF current from the electrodes penetrates the epidermis and concentrates in areas with high electrical conductivity. The pattern of distribution of electrical current demonstrates the possibility for selective treatment of blood vessels using conductive RF.
Figure 3-3 Normalized distribution of electrical current density as a function of the depth for different distances between electrodes in a bipolar system.
Tissue | Conductivity (S m m–1) |
Blood | 0.7 |
Bone | 0.02 |
Fat | 0.03 |
Dry skin | 0.03 |
Wet skin | 0.25 |
Temperature Dependence of Electrical Conductivity
Tissue conductivity is significantly correlated with tissue temperature. The thermal coefficient (α) of skin conductivity is ∼2% C−1; that is, every 1°C increase in temperature lowers skin impedance by 2%.9 Therefore, the distribution of electrical current can be controlled by precooling or preheating different parts of tissue. Fig. 3-5 describes the distribution of electrical current as a function of the depth of tissue with uniform temperature distribution for skin surface that has been precooled by 25°C. Surface cooling drives electrical current inside the tissue, increasing penetration depth. Likewise, preheating the target tissue increases conductivity and consequently selective heating by the RF current. Therefore, if the temperature of the target tissue is higher than that of the surrounding tissue, then the RF current will selectively focus to increase heating of the target tissue.
Clinical Applications
In aesthetic medicine, the use of RF as an energy source for selective electrothermolysis is a relatively new concept. Systems that incorporate the RF current have been in development for several years but were introduced only recently. Studies using these new technologies have demonstrated utility in hair removal, skin rejuvenation, and wrinkle reduction.
Hair Removal
The effective removal of unwanted hair using optical energy has been essentially limited to black and dark and medium tones of brown hair.10 Treatment of light-colored hair has been particularly difficult because of the limited concentration of melanin chromophores in hair follicles. In addition, safety is a concern in dark skin types, who have an increased risk of blistering and dyschromia, even with longer-pulse lasers.11 It would make sense that the combination of low levels of optical energy and electrical conducted RF current, the latter of which is not dependent on melanin for thermal absorption, may be able to effectively and safely remove hair, including light-colored hair, for all skin types.
Figure 3-4
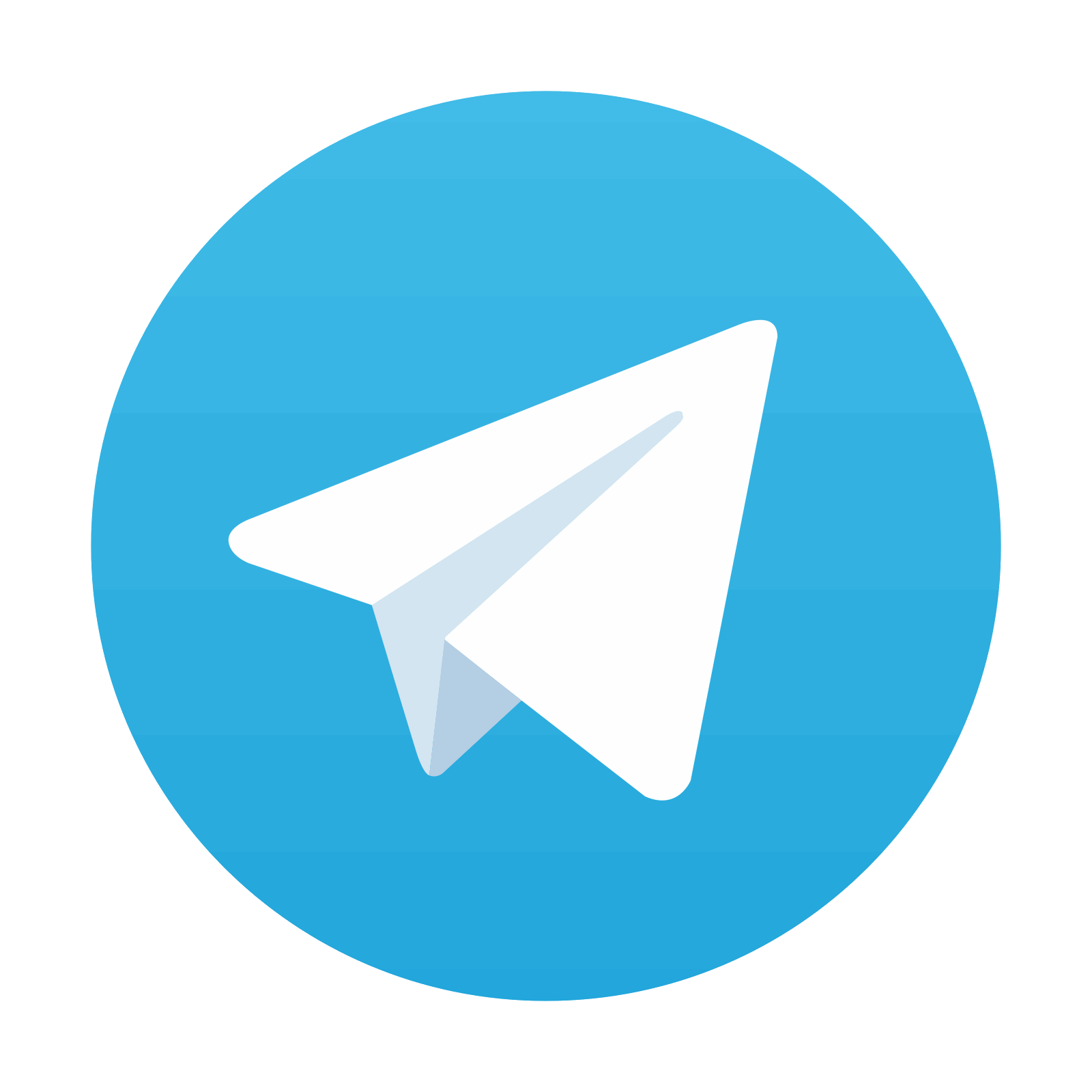
Stay updated, free articles. Join our Telegram channel

Full access? Get Clinical Tree
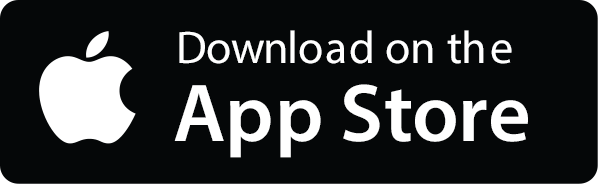
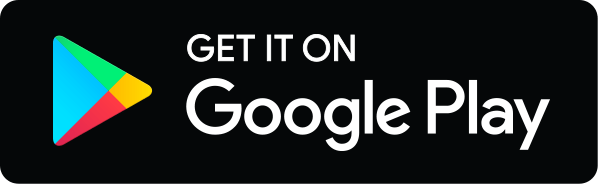