Fig. 31.1
Spectral signature and irradiance in mW/cm2 of three test lamps with mixed outputs used in PPT study 1 (a) VL lamp, (b) UVA tubes, and (c) IR bulb
Results were as follows: VL had the most number of (+) reactions that were reviewed and reported separately in 2008 [21]. All (+) reactions were of the phototoxic type and relevant, to make the diagnosis of VL-Phototoxic Contact Dermatitis, with persistent pigment at all (+) PPT sites. In the melasma group, 55 % (N = 11/20) had 29 (+) PPT reactions to 11 fragrances, 11 North American, and seven plant photoallergens (PAs); no reactions were noted in the non-melasma group. This association was highly significant (P = 0.00, two-tailed Fischer’s exact test) such that compared to a (−) PPT, a (+) reaction predicted 12.67 times increased likelihood of having melasma (P = 0.05; 1.402–114).
UVA irradiation had 10 (+) phototoxic type reactions with persistent pigment at (+) PPT sites. IR had 12 (+) phototoxic type reactions but showed no persistent pigmentation.
31.2.1.2 PPT Study 2 (2010) [22]
This consisted of a case series of 20 Filipinos with melasma and irregular melasma-like pigmentation, without dermatitis on their extensor arms and V-area of the neck. The same photosensitizers as in Study 1 were used, with a Birtcher lamp for PPT (Fig. 31.2).


Fig. 31.2
Birtcher lamp: a 20 W mercury arc lamp with a barium silicate–nickel oxide filter
PPT using low-energy Birtcher or Wood’s lamp has shown photoallergy [23] to musk ambrette [24] and to other men’s perfumes [25].
In the case series (Fig. 31.2), many (+) decrescendo reactions to perfume (6/20, 30 %), plant (16/20, 80 %), Scandinavian series (11/20, 55 %), and SSLI sunscreen series (8/20, 40 %) were seen, all in concordance with persistent pigmentation at (+) test sites.
Diagnosis was UVA 1 – phototoxic contact dermatitis with pigment persistence at (+) PPT reaction sites.
For the first time, these PPTs showed (+) responses to VL and IR, not just to UV, the accepted action spectrum of most PS. These appeared to be phototoxic reactions (macular, non-spreading, and not pruritic). The persistent post-exposure hyperpigmentation clinically mimics post-inflammatory hyperpigmentation seen after most photosensitivity reactions in brown skin. Of relevance is that presently, it is now accepted that compact fluorescent lamps emit a mixed spectra of UV, VL, and IR [26, 27]. Despite the low radiation dosage emitted by these indoor lights, landmark papers have established the principle of “cumulative damage” referring to the repeated sub-erythemal radiation exposures that eventually result in erythema and lower the MED [28–30]. The next step was to find out if like UVA, the standard PPT test energy pure VL can also elicit PACD.
31.2.1.3 PPT Study 3 (2013–2015) [7]
This study used an LED lamp emitting pure VL (Fig. 31.3) for testing four patients with recalcitrant melasma and photosensitive hyperpigmentation even when using broad-spectrum sunscreens. Pure VL-PPT in four patients resulted in only a few (+) reactions of the crescendo type, with spreading borders, and pruritus lasting up to 2 weeks. These were interpreted as photoallergic reactions. The resulting pigmentation persisted for weeks to many months.


Fig. 31.3
LED pure VL spectrum 400–760 nm, no UV or IR, used in Study 3 VL-PPT
Figure 31.4a–f shows typical VL-PPT (+) reactions to p-Phenylenediamine in one of the patients. He had both allergic contact dermatitis and VL photoallergic contact dermatitis. Photoaggravated CD was ruled out because the (+) reactions lasted for at least 2 weeks and more.


Fig. 31.4
LED-VL-PPT to p-Phenylenediamine
A PPT done in 2007 used the UVA tubes (shown in Fig. 31.1b) and the PSs in PPT Studies 1 and 2. Unirradiated sites (Fig. 31.4a–c) had many (+) reactions: 10 of 13 plants, 8 of 21 Scandinavians, and 6 of 24 fragrance PSs. These results were interpreted as angry back or excited skin at unirradiated sites. UVA-Irradiated PSs were all (−) except for one fragrance PS (Fig. 31.4d–f). The minimal reaction at the irradiated sites was signed out as photoinhibition.
Persistence of a photo-distributed eruption led us to perform a follow-up PPT (2013) using a pure VL LED lamp and 64 PS: 55 from the American Contact Dermatitis Society (ACDS) 80-allergen series and nine sunscreen ingredients from SSLI. The same 64 PS served as unirradiated controls. While PPT to mixed energy lamps elicited PTCD, PPT using pure VL elicited PACD followed by clearance of the pigmentations on avoiding the PS, the eliciting lights, and with the use of daily sunscreens with protection factors to VL and IR (Fig. 31.5).


Fig. 31.5
Filipino male, 57 y/o, with recurrent melasma since the 1990s. VL-PPT done in 2013. 2013 Before VL-PPT (a) right side of the face, (b) left side of the face 2015 Same patient, after the 2013 VL-PPT and use of appropriate measures to control melasma (c, g) right side of the face, (d, e) left side of the face, (f) full front face
31.2.2 Pigmentation Induced by Radiation: In Normal Skin
Reaction to UVB starts with erythema followed by a protective tan from an increase in the number of melanocytes, new melanin production, and redistribution to keratinocytes. UVA-induced pigmentation is caused by neomelanogenesis and is brought about by immediate photooxidation of existing melanin/precursors/metabolites. The molecular and biologic changes of these UV-induced pigmentations are well studied, and current broad-spectrum sunscreens are protective [31, 32].
31.2.3 Beyond UV, VL, and IR Studies [33]
In electron spin resonance, activation of endogenous photosensitizers was shown in ex vivo skin explants exposed to filtered natural midday sunlight [34]. The reactive oxygen species (ROS) generated is calculated at 4 % from UVB, 46 % from UVA, and 50 % from VL. In an in vitro study, exposure to VL resulted in increased ROS production, increased expression of MMP-1 and TNF-α mRNA in epidermal keratinocytes [35]. Aside from photooxidation, ex vivo hormonal/molecular and clinical studies showed that even in Caucasians, but more so in brown skin, VL also activates melanogenesis after multiple (preconditioning) VL exposures and even at lower doses of VL [36, 37]. These findings align well with the VL-induced skin pigmentation demonstrated in skin tests and in histopathology of Caucasian versus brown skin exposed to 400–800 nm artificial light at 80–480 mW/cm 2 [38–40].
Consistent with these findings is the persistent pigment at (+) PPT reaction sites of PS exposed to pure VL or to mixed spectrum indoor light sources. In the author’s studies on normal subjects to determine the MED to VL for sunscreen protection factor, pigment likewise persisted for several weeks before fading away gradually [7].
Although indoor radiation fluences are low and minimized further by movements away from the light source, cumulative damage to chronic low-dose sub-erythemal radiation has long been shown in normal and in photosensitive skin [41, 42].
31.3 Vitiligo in Brown Skin: Photoprotection as Part of Therapy
Although normal skin of Fitzpatrick phototypes I to II and the vitiligo macule look similar to the naked eye, they differ at the molecular level. Normal phototypes I and II skin has melanin-producing melanosomes within melanocytes possessing dendritic connections to about 40 keratinocytes. Vitiliginous skin has few, if any, functioning melanocytes, melanosomes, or melanin; thus, cannot respond to sun exposure by increased production of sun-protective melanin [45].
Global studies have shown that chronic sun exposure and a history of sunburns contribute to the increasing incidence of melanomas and non-melanoma skin cancers (NMSC) in people with types I and II [46] skin and a similar, though smaller, increase in brown skin types III–V [47]. Clinicians are concerned about contradictory reports on the incidence of melanoma and NMSC in vitiligo patients prompting a recent paper on the subject [48]. Of the 1024 retrieved articles on melanoma and NMSC in vitiligo in this review, five were selected to assess best evidence.
A case-control questionnaire survey by Teulings, et al., (the Netherlands, mostly skin types II and III) on 1307 patients with vitiligo and their normal partners, found a threefold decreased probability of melanoma (odds ratio OR 0.32, 95 % CI 0.12–0.88) and NMSC (OR 0.28, 95 % CI 0.16–0.50) after adjusting for risk factors (>100 nevi and sunburn in childhood). Seven melanomas occurred in those with normal pigmented skin and two basal cell carcinomas (BCC) within vitiligo lesions. The questionnaires were answered by patients at their homes without the assistance of a trained physician; hence, accuracy of patient recall may be questionable [49].
The next four were cohort studies. In Paradisi et al.’s study, from a large reference center in Rome (no skin types given), 11 melanomas occurred in 10,040 vitiligo patients and 118 in the control group of 25,956 non-dermatological patients seen for vascular surgery (RR 0.24 95 % CI 0.13–0.45). There were 37 NMSC in vitiligo patients, 509 in the control (RR 0.19 95 % CI 0.14–0.17). This study had a large number of subjects and good internal validity, but there was an age distribution difference in the two groups [50].
Another relatively large prevalence study of Lindelof et al. from two Swedish hospitals (no skin type given) consisting of 1052 vitiligo patients found only one with melanoma and no SCC. The review authors calculated an absolute risk of developing melanoma at 0.001 (95 % CI 0.0002–0.0054). This study did not elaborate on factors that could have influenced the outcome [51].
Schallreuter et al. reported on 136 Caucasians with vitiligo who were screened for NMSC. No melanomas, BCC, or SCC were seen. Despite a significant number of early childhood sunburns, there was no histologic evidence for sun-related damage and no increased risk for common photodermatoses [52].
Hexsel et al. screened 477 vitiligo patients for the occurrence of NMSC which resulted in a calculated absolute risk of 0.013 (95 % CI 0.0058–0.0272). There was one basal cell carcinoma (BCC) and one squamous cell carcinoma (SCC) on vitiliginous skin, three BCCs and one SCC on normally pigmented skin [53].
While the above studies showed a decreased incidence of vitiligo skin cancers, in the largest cohort of 10,040 patients, Paradisi found that those who underwent phototherapy when compared with those who did not had a higher and statistically significant risk for skin cancers. The phototherapy group risk for melanoma was 7.54 (95 % CI 1.99–28.56); for NMSC, it remained high at 4.7(95 % CI 2.0–10.8). Anecdotal cases of SCC on exposed and non-exposed skin of vitiligo in brown skin, with or without phototherapy, also continue to be reported [54–59].
The authors of the Hammoud review [48] warn that since none of the studies described the extent of vitiligo nor included patients who died from melanoma or NMSC, risk underestimation is a possibility.
Racially, Hexsel’s cohort of vitiligo patients were 42 % Caucasian, 41 % Brown (29 % African-American, 6 % Asian-Pacific Islander, 2 % Hispanic, 2 % Middle Eastern, 2 % Native American) and 17 % with unavailable racial designations. The small sample of 477 vitiligo patients was deemed not large enough to detect skin cancer in brown skin as only Caucasians had the six NMSC (four in vitiligo, two in normal pigmented skin). This was the only study on incidence rates comparing the vitiligo cohort with two general US populations. Their results suggest that there may be an increased or equal risk of NMSC in vitiligo patients, a finding that may run counter to the long-standing belief of decreased skin cancer risk in Caucasians with vitiligo. The authors caution that health care providers should not underestimate the risk of this common cancer in vitiligo patients [52]. Possible reasons for the negative association between melanoma and NMSC in individuals with vitiligo may be (1) patients with vitiligo probably have been told to protect their skin against the sun and (2) an over expression of the p53 tumor suppressor gene which can kill cells via a dual transcription-dependent and -independent function in the nucleus and at the mitochondria has been proposed to explain this low BCC and SCC risk.
In normal unstressed cells, p53 is a very unstable protein with a half-life from 5 to 30 min, present at very low cellular levels due to continuous degradation mediated by murine double minute 2 gene (MDM2) [60]. A study on phototypes III and IV brown-skinned Egyptians with vitiligo (all with outdoor occupations) demonstrated significantly strong expression of p53 and MDM2 proteins versus controls in normally pigmented and depigmented lesions at the epidermis, skin adnexa, and blood vessels and higher in those with generalized than with localized vitiligo. The rapid induction of high p53 protein levels may prevent inappropriate propagation of cells carrying potentially mutagenic damaged DNA such as after sun exposure [61].
31.4 Sunscreens: Testing, Labeling, Efficacy, Safety
The US FDA 2011 Final Rule [64] is mostly aligned with the European cosmetic, toiletry and perfumery industry’s COLIPA. These, the ISO, and regulatory bodies in the UK, Australia, New Zealand, Japan, and the ASEAN’s harmonized rule differ in accepted ingredients, concentrations, and classification as over-the-counter (OTC), therapeutic drugs, or cosmetic. However, all agree that the ideal profile is uniform protection across the entire UVB/UVA range (290–400 nm) similar to that provided by sun avoidance [65].
Sun protection factor (SPF) protects in the UVB 290–320 nm range and is obtained through an in vivo methodology on at least ten subjects using a xenon arc solar simulator with specific guidelines on test light specifications, usage, and testing methodology. Labeling should include uses, warnings, directions, non- or water resistance, and the listing first of active and then inactive ingredients in alphabetical order.
UVA protection factor (UVA-PF) testing utilizes the more ethical in vitro method that is well on its way to being harmonized among the various regulatory bodies. The US FDA mandates determination of the critical wavelength (CW) at which 90 % of the UVB and UVA is absorbed, by a pre-irradiated test sunscreen film applied at 0.75 mg/cm2 on a polymethyl methacrylate (PMMA) plate. CW greater than 370 nm is the simple pass/fail criterion for UVA-PF, which if 1/3 the SPF of 50+, the sunscreen can be claimed to be broad spectrum.
For the author, it is but proper that all sunscreen products must undergo aforementioned tests to prove the veracity of their claims of “broad spectrum” and protection factor number; efficacy cannot be based on ingredients alone. These details on how sunscreens are evaluated for efficacy and safety are mentioned here because in some countries, there are products that claim “broad-spectrum sunscreen” and protection factor numbers based just on ingredients in the formulation and unsupported by the above testings. An informal survey by the residents of the Skin and Cancer Foundation, Inc. (Philippines) showed several such “sunscreens” sold as cheaper products to the public and even to dermatologists who dispense them with labels as claimed by the sellers who often are compounding pharmacists or chemists without a photo laboratory to support their label claims.
VL and IR protection factors: There are as yet no standard guidelines on determination of protection factor (PF) determinations for VL and IR but some companies are already responding to this need [66, 67]. At our center, these VL and IR-PPT studies were initiated and the need to photoprotect against VL and IR was substantiated. We have adapted the methodology of UVA for in vivo PF testing but used the LED-VL for VL and un-/cooled IR for IR. These studies achieved up to 6 VL-PF and 6 IR-PF.
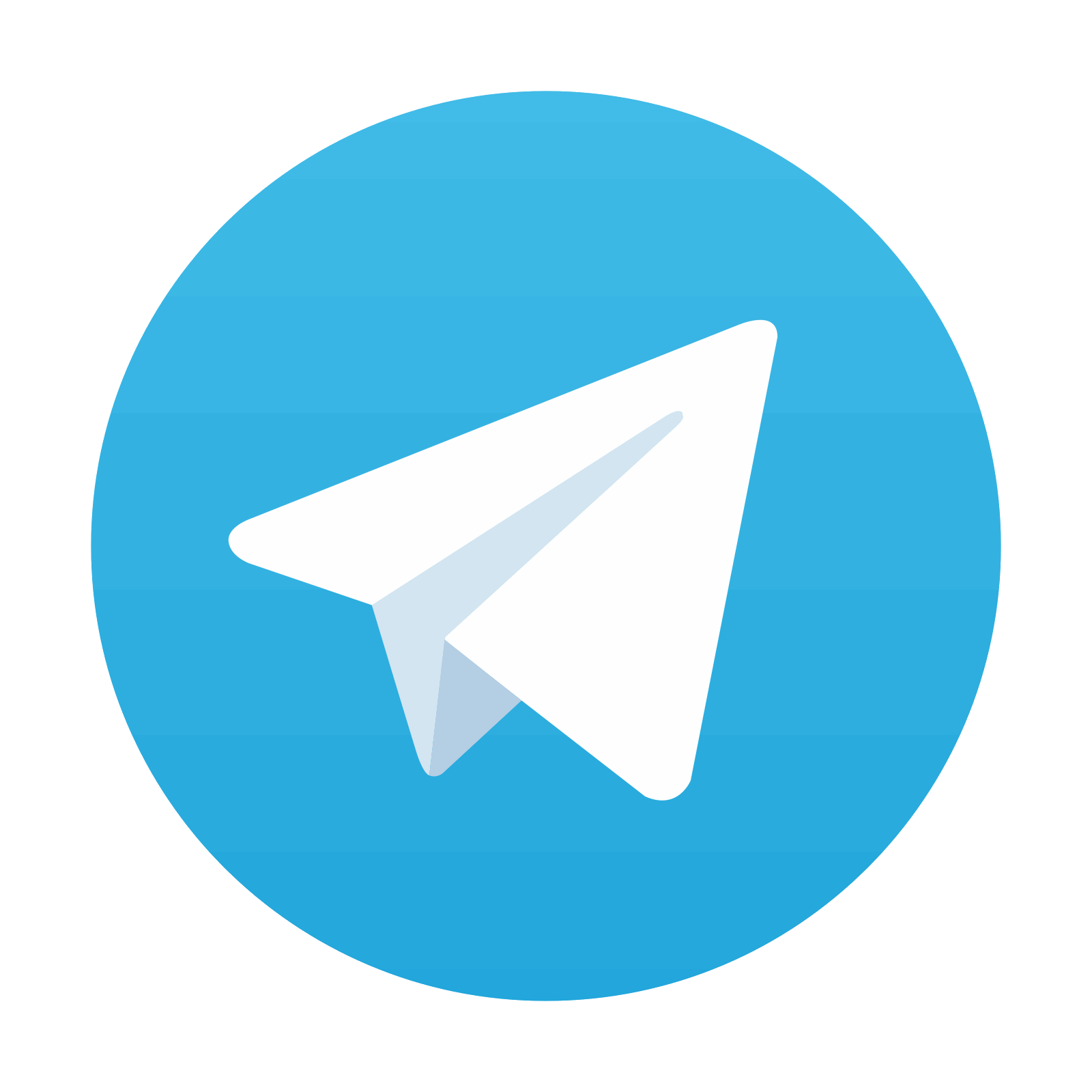
Stay updated, free articles. Join our Telegram channel

Full access? Get Clinical Tree
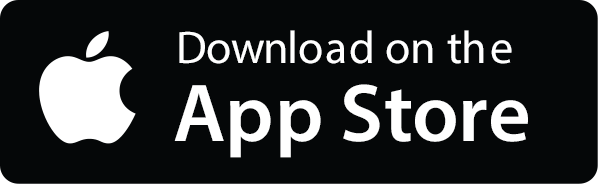
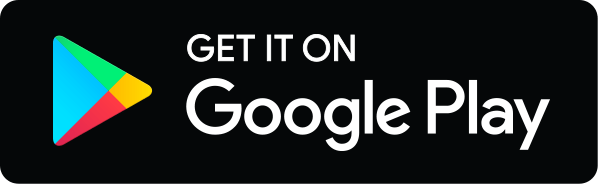