Psoriasis is a model disease for the development of pharmacogenomic markers of treatment response, with ready access to diseased tissue and objective validated outcome measures. With the application of state-of-the-art technologies and investment in careful experimental design, the goal of stratified medicine in psoriasis may be possible. Current pharmacogenomic studies in psoriasis show excellence in many areas, including the investigation of a broad range of psoriasis therapies. To facilitate the advent of stratified medicine in psoriasis, uniformity of study design is required, with high quality, consistent phenotyping strategies for participants; definitions of outcome; and the publication of reproducible methodologies.
Key points
- •
Pharmacogenomics is the study of the relationship between drug response and a genomic test, broadly to include changes in the genome, epigenome, transcriptome, proteome, or metabolome.
- •
Stratified medicine refers to the potential to make clinical decisions based on a pharmacogenomic test by adjusting dosage or choosing a different drug.
- •
Psoriasis is a model disease for the development of pharmacogenomic markers of treatment response, with ready access to diseased tissue and objective validated outcome measures.
- •
With the application of state-of-the-art technologies and investment in careful experimental design, the goal of stratified medicine in psoriasis may be possible.
Introduction
Pharmacogenomics
The way in which an individual responds to a drug is affected by many factors, including those related to the drug, the disease, and the individual. Pharmacogenetics , a term suggested by Vogel in 1959, is the study of relationships between genetic polymorphisms and drug response.
A gene can be defined as exhibiting a polymorphism if the variant allele exists in the normal population at a frequency of at least 1% (Meyer 2000). The most frequent type of polymorphism is a single nucleotide polymorphism (SNP); however, they can take on several forms, including nucleotide repeat sequences. Polymorphisms may result in altered efficacy or toxicity of a given drug through mechanisms such as alteration of its absorption, distribution, metabolism, and elimination.
The presence of a genetic polymorphism is not the only way in which drug response may vary; the potential for posttranslational changes, including gene–environmental interactions, also is critical. Although the terms pharmacogenetics and pharmacogenomics are often used interchangeably, pharmacogenomics encompasses changes in not just the DNA code but also the transcriptome, metabolome, and epigenome and their interrelationships to identify their influence on response to treatment.
Personalized Therapies
When a pharmacogenomic investigation finds a marker or markers predictive of response to treatment, there is potential for making clinical decisions based on a pharmacogenomic test by adjusting the dosage or choosing a different drug. Drug treatment may be referred to as targeted, as each patient may have treatment selected based on their own rather than population characteristics. This process is referred to as personalized or stratified medicine. Advantages of the application of pharmacogenomics are far reaching, allowing minimization of treatment cost and the improvement of treatment safety through the reduction in adverse events. Furthermore, individualization of therapy may allow drug repositioning, as an existing therapy may be newly applied to a selected cohort of another disease. To achieve the goals of personalized medicine, testing in the field must be accurate in predicting drug response and be cost effective. The potential properties of an ideal pharmacogenomic test are shown in Fig. 1 .
The Successes of Personalized Medicine in Other Fields
Translation of pharmacogenomic studies has resulted in major successes in personalized medicine, exemplified by progress in oncology.
A key example is that of the epidermal growth factor receptor (EGFR) pathway with activation being necessary before the development of non–small cell lung cancer. As a result, EGFR has become a key focus for the development of targeted therapy with certain chemotherapeutic agents only being suitable in individuals expressing specific mutation in the EGFR gene. Diseased tissue, in the form of cancer biopsy specimens, allows ready testing of individual genetic/genomic markers but is often challenging to obtain because of anatomy or access. In the case of EGFR inhibitor therapies, a focus on the development of genomic markers extracted from blood rather than tissue may soon be possible.
Inflammatory Conditions
Numerous studies have sought genetic determinants to therapies in the treatment of inflammatory conditions such as rheumatoid arthritis, asthma, and inflammatory bowel disease.
In the treatment of rheumatoid arthritis, the common tumor necrosis factor ( TNF ) −308G>A promoter-region polymorphism was found to be modestly predictive of poor responses to TNFI therapy, irrespective of the agent used. Variation in the CD84 gene, which encodes SLAM family member 5 (also known as leukocyte-differentiation antigen CD84), has been shown to be predictive of responsiveness to the soluble TNF receptor 2 fusion protein, etanercept ( P = 8 × 10 −8 ), and the PDE3A–SLCO1C1 locus, which harbor the genes encoding cGMP-inhibited 3′,5′-cyclic phosphodiesterase A and solute carrier organic anion transporter family member 1C1, has been associated with response to the TNF inhibitors etanercept, infliximab, and adalimumab.
In the study of asthma, genes have been identified as important in the response to both inhaled corticosteroids and inhaled short-acting β-agonists. Transcriptome profiling is now being used to offer a novel and comprehensive assessment of glucocorticoid response. For those with severe disease, the drug pipeline of biologic therapies holds great promise, with TNF inhibitors forming only part of the number of agents, with others targets including interleukin (IL)-4, the IL-4 receptor, and IL-13. The hope is that these therapies may be personalized, and interaction has been shown between anti–IL-4 receptor-α therapy and IL4RA gene variation.
However, none of the described associations have yet proven sufficiently predictive to inform clinical decisions regarding selection of the most appropriate therapy for individual patients.
Pharmacogenomics and Dermatology
Melanoma treatment
Improved survival in melanoma has been reported in those treated with the BRAF kinase inhibitor, vemurafenib, with a V600E mutation in the BRAF gene. In a phase III clinical trial, treatment with vemurafenib was compared with the melanoma chemotherapy agent, dacarbazine. At the time of study, dacarbazine was the only agent approved by the US Food and Drug Administration for the treatment of metastatic melanoma. Mutations in the proto-oncogene BRAF gene led to downstream activation of MAPK pathway and occur in 40% to 60% of melanomas with 90% of these mutations resulting in the substitution of glutamic acid for valine at codon 600 ( BRAF V600E ). Although these targeted therapies have shown some clinical promise, their use has been limited by partial response and high relapse rates. The overall survival benefit is modest, with various mechanisms of drug resistance described, and future efforts may rely on the development of successful combination therapies aimed at simultaneously targeting tumor mechanisms and circumventing resistance strategies.
Azathioprine dosing
Azathioprine dosing based on thiopurine methyltransferase (TPMT) measurement provides an example of the application of pharmacogenetics in dermatology. Common polymorphisms in TPMT, a key enzyme in thiopurine metabolism, can lead to variable levels of myelosuppression in individuals treated with azathioprine. A randomized, placebo-controlled, double-blind trial of azathioprine use in moderate-to-severe atopic eczema determined that with use of a TPMT-based azathioprine dose regimen, drug efficacy could be maintained while avoiding drug toxicity.
Dapsone
Dapsone is a sulfonamide the licensed indications of which include use in dermatology for the treatment of dermatitis herpetiformis. Patients with glucose-6-phosphate dehydrogenase (G6PD) deficiency are susceptible to hemolysis when treated with oxidative drugs such as dapsone. G6PD exhibits high genetic diversity, and when dapsone treatment is planned, determination of G6PD status should be considered in at-risk populations.
With the assessment of TPMP and G6PD, enzyme activity is measured by the pretreatment test and not directly the patient genotype.
Pharmacogenomic Investigational Design for Psoriasis
To facilitate the advent of stratified medicine, pharmacogenomic studies must be designed with translation to the improvement of clinical care in mind. In the case of psoriasis, high-quality study design with use of adequately powered, well-phenotyped cohorts is necessary and may require application of one of the newer omics technologies. Because this field is rapidly evolving, these new technologies are briefly reviewed.
Technologies
Over recent years, the use of nucleic acid sequencing has increased exponentially as the ability to sequence has become accessible to research and clinical laboratories worldwide. Next-generation sequencing refers to any technology that uses a high-throughput parallel approach to sequencing large numbers of different sequences (a library) in a single reaction. RNA profiling exploits recent advances in next-generation sequencing to provide a comprehensive measurement of the genes being expressed in a sample. RNA sequencing (RNA-Seq) can complement traditional histopathologic, immunologic, cytogenetic, and proteomic means of pharmacogenomic analysis. The open platform offered by RNA-Seq also enables direct comparison with other open access research data. Although the association in genomic studies tends to be of one or more SNPs with either response to (or toxicity of) a therapy, in transcriptomic studies this may be in the form of a gene expression profile or signature of treatment response. Gene expression profiling from lesional versus nonlesional skin in a patient with psoriasis may play a key role in establishing biomarkers of treatment response in psoriasis.
Epigenetics is the study of mitotically heritable changes in gene expression that do not involve any alterations in the underlying DNA sequence. Compared with the largely static DNA sequence, the epigenetic status of the genome varies dynamically in different tissues. Identifying epigenetic abnormalities involved in the onset and progression of disease is of great relevance to the development of new therapeutic approaches because, unlike genetic mutations, epigenetic aberrations are potentially reversible with the use of pharmacologic agents. The 3 main epigenetic mechanisms are DNA methylation, histone modifications, and RNA-associated gene silencing.
Untargeted, global metabolite profiling, referred to as metabolomics (or metabonomics ), can offer insight into disease processes, drug toxicity, and response to therapies. Obtaining metabolic profiles from complex samples, such as biological fluids, requires powerful, high-resolution and information-rich analytical methods, for which spectroscopic technologies are generally used, (eg, mass spectrometry). Metabolite separation techniques, including liquid chromatography, can reduce complexity and provide additional information on metabolite properties to aid metabolite identification.
Pharmacogenomics of Psoriasis
Pharmacogenomic studies to date in psoriasis, as in many other areas of medicine, have been underpowered and have lacked robust, prospectively acquired data with clear delineation of disease response and adverse events, such that major advances in this important area have not been made. Studies have varied as to their application of available technologies, with some studies not designed as pharmacogenomic association studies but as investigations of genomic or transcriptomic changes with therapy that may lead to advances in understanding of psoriasis pathogenesis. These studies were, therefore, not designed primarily with power to detect association with treatment response. This finding has been exemplified by the lack of replication in studies to date.
Topical Therapies
Several early pharmacogenetic studies have evaluated a possible association between polymorphisms in the vitamin D receptor (VDR) with the clinical response to topical vitamin D analogue preparations. Studies to date have varied in design, including the choice of topical agent and how it was administered to the patient, cohort size, time point of assessment of response, and how response has been measured (eg, patient self-reported data vs physician assessment). Studies varied in the technology applied and whether an association between the polymorphism evaluated and response to treatment was reported. When an association has been found with a polymorphism in the VDR, the findings have not been replicated nor has the associated polymorphism been found to be functionally active. To date, no convincing biomarker of response has been found for any topical therapy used in the treatment of psoriasis.
Pharmacogenomics of Phototherapy
Pharmacogenomic studies in psoriasis patients treated with ultraviolet (UV) radiation are limited. One group investigated if interindividual differences in the cutaneous expression of a variety of drug-metabolizing and cytoprotective genes, including cytochrome P450s and drug transporters, occurred in those exposed to ultraviolet radiation. The group found marked interindividual differences in the constitutive expression of many drug-metabolizing enzymes. However, this study correlated the UV radiation treatment with change in gene expression rather than improvement of psoriasis. Thus, further research is required to determine the potential role of these genes as biomarkers of treatment response.
Genetic polymorphisms in the p53 gene and in the VDR gene have been evaluated in relationship to clearance of psoriasis with narrow-band UVB phototherapy in 2 studies. The Arg/Arg homozygote state of the p53 gene was reported as significantly increased in the response group, but from patient-reported data the Taq1 VDR polymorphism was found to be predictive of remission duration.
In a study of 32 patients receiving either narrow-band UVB or bath psoralen ultraviolet A (PUVA) phototherapy, IL-6 levels were measured before and after courses of treatment, and IL-6 levels correlated with percentage reduction in the objective validated psoriasis outcome measure, Psoriasis Area and Severity Index (PASI). Limitations of this approach include lack of description of participant phenotype, lack of control for additional therapies, and correlation of the investigated marker with a nonvalidated method of assessment of response.
Several studies have investigated the gene expression profile from lesional skin from patients with psoriasis treated with phototherapy, which, although not originally intended to find a single pharmacogenomic marker of treatment response, are of interest to inform future pharmacogenomic work. An example of this work would be the identification of significant reduction in inflammatory cytokines including IL-20 and IL-23 in responding and not nonresponding plaques of psoriasis during treatment with narrow-band UVB phototherapy. These findings can be taken forward for validation through adequately powered pharmacogenomic investigations.
Methotrexate
One of the largest and most comprehensive pharmacogenetic studies in psoriasis has been the evaluation of SNPs on treatment response to methotrexate. Adults with chronic plaque psoriasis treated with methotrexate were recruited retrospectively, and 84 SNPs from 5 genes involved in pharmacodynamics factors related to methotrexate were analyzed. Three SNPs in the gene ABCC1 (from the efflux transporter ATP-binding cassette subfamily C) and 2 of the SNPs (introns 2 and 9) in the gene ABCG2 (ATP-binding cassette, subfamily G, member 2) were associated with response to methotrexate. No significant association was detected between clinical outcomes in patients with psoriasis treated with methotrexate and SNPs in 4 genes coding for enzymes involved in methotrexate intracellular metabolism. An earlier study, which used some of the same patients studied by Warren and colleagues, found that the reduced folate carrier 80A allele was associated with methotrexate toxicity and the thymidylate synthase 3′-UTR 6 base-pair deletion was associated with poor clinical response to methotrexate. Although of some promise, these studies have not been repeated and no clinical translation has yet ensued. Furthermore, characterization of treatment response retrospectively is a significant weakness of all these studies.
Acitretin
Acitretin is a synthetic retinoid that is a well-established second-line agent in the treatment of severe psoriasis. Although acitretin has relatively low efficacy and is poorly tolerated because of numerous side effects, including mucocutaneous dryness (the extent of which frequently limits dosing), hyperlipidemia, and teratogenicity, it has a place as either monotherapy or in combination with phototherapy, in the treatment of a subset of patients with severe disease. There is limited pharmacogenomic work for this therapeutic agent, but polymorphisms of the apolipoprotein E gene ( APOE ) and the vascular endothelial growth factor gene ( VEGF ) have been evaluated as predictors of response to psoriasis patients treated with acitretin. Although the frequency of the APOE e4 allele (+3937C/+4075C) was higher in patients with chronic plaque and guttate psoriasis than in controls, there was no significant difference in the frequency of alleles in acitretin responders and nonresponders. There was a significant increase in the frequency of the -460 TT genotype of the VEGF gene in patients who were nonresponsive to acitretin therapy compared with those who cleared with treatment, but the numbers of patients in these studies was small.
Ciclosporin
Several studies have evaluated gene expression profiling during ciclosporin therapy. All reported significant changes in disease-related genes in patients responding to ciclosporin.
All studies were limited by small cohort size. In 2 cases, the primary agent under investigation was a multifunctional cytokine, recombinant human IL-11, with ciclosporin-treated patients acting as a comparison cohort.
Biologic therapies for psoriasis
Several pharmacogenomic studies have been carried out investigating potential predictors of biologic treatment response. Investigational approaches have varied in terms of agent(s) being studied the use of hypothesis free versus a candidate gene approach and therefore the technologies used. As in studies of biologic response in other inflammatory disorders, candidate genes for investigation include those known as markers of disease associations. Further candidates have been genes from relevant pathways of drug action including polymorphisms of receptor activity.
At least 44 SNPs in the tumor necrosis factor (TNF) gene have been identified, and 2 SNPs in the promoter region (G-to-A transitions at the -238 and -308 sites) seem to be functionally relevant, affecting TNF expression. Tejasvi and colleagues reported their study of 683 patients who received anti-TNF therapy or systemic agents for the treatment of psoriasis. SNPs in the promoter region of TNF were examined and compared with drug response (however, response was self-evaluated). An association was seen among adalimumab responders with the TNF-1031T/C polymorphism (overall risk, 4.43, P = .04).
The HLA-Cw*0602 allele of the human leukocyte antigen (HLA) class I HLA-Cw6 is the main genetic determinant of psoriasis, designated “psoriasis susceptibility” 1 (PSORS1). Pharmacogenetic studies of the HLA-Cw*0602 allele have not shown an association with response to anti-TNF therapy. In a retrospective study of 98 patients with chronic plaque psoriasis treated with etanercept (n = 78) or adalimumab (n = 50), the presence of the HLA-Cw*0602 allele did not predict response to etanercept, and there was a nonsignificant trend suggesting that the HLA-Cw*0602–positive patients were more likely to respond to adalimumab.
Gene expression profiling from lesional versus nonlesional skin in a patient with psoriasis may play a key role in establishing biomarkers of treatment response in psoriasis. Krueger and colleagues evaluated gene expression profile from 15 patients treated with etanercept (50 mg twice weekly) at baseline and after 12 weeks of therapy. The primary outcome measure was to evaluate how “closely the transcriptome returned to the non-lesional state in responders.” However, limitations of this approach include that response was determined histologically as described in the group’s previous report through epidermal thickness and keratin 16 immunohistochemistry from samples taken at 12 weeks of etanercept therapy (50 mg twice weekly.) The mean percentage clearance of the 16 responders from the original cohort of 20 patients was 74.5%. Improvements in objective validated outcome measures for psoriasis were not indicated.
The results indicated that 248 genes did not return to baseline expression after 3 months of treatment. Microarray work was conducted using a previously established psoriasis transcriptome and the current cohort assessed for resolution using the differently expressed genes from the group’s previous works. From these, genes with less than 75% improvement made up a residual disease genomic profile. In the group of upregulated genes, 76 probe sets (64 genes) did not improve beyond 75% with treatment and 206 probe sets (184 genes) from downregulated genes did not show 75% improvement. The microarray chips did not reliably detect several key cytokines, including interferon-γ, IL-17, and IL-22.
The team also hypothesize that altered expression of genes associated with structural cell types leaves a molecular scar in resolved plaques of psoriasis. Many of the genes that did not completely resolve with treatment involved structural cutaneous components, including markers of lymphatic endothelial cells. Biologic pathways were evaluated for gene changes. Only selected pathways are presented in the data, including the leptin pathway, in which the change was 56% improvement with treatment.
This group has further attempted to develop a genomic profile to predict histologic response to the anti-CD2 fusion protein, alefacept. In a cohort of 22 patients treated with a total of 12 weekly intravenous 7.5-mg doses of alefacept, response was determined histologically as described in their earlier work. Gene expression profiling was conducted on peripheral blood mononuclear cells from baseline samples, and 23 threshold genes were selected to form a classifier to predict response using the pretreatment blood measurements. The study used only a small cohort, and 2 of the nonresponding patients were misclassified as responders. Of interest, gene expression profiling was conducted in peripheral blood mononuclear cells at 24 hours of therapy. At this early time point, there was suppression of inflammatory genes, although data are not illustrated. The group previously published work on the agonistic mechanism of alefacept from data from the same cohort. In this earlier work, gene expression profiling was conducted at baseline, 1, 6, and 24 hours and weeks 2, 4, and 13 (before alefacept administration). A significant increase was seen in the proinflammatory genes STAT1 and MIG within the first day of treatment, but gene expression normalized by week 13 of treatment. However, several immune-related genes were downregulated with treatment, and the group hypothesized that their results supported “negative regulation of genomic pathways in T cells that could influence long-term activation or reactivation responses of T cells.” Gene expression profiling was separated by histologic response to therapy, and Foxp3 , a marker of regulatory T cells, was induced in responders preferentially to nonresponders 6 hours after treatment.
The relationship between the presence of the HLA-Cw*0602 genotype in patients with psoriasis has also been evaluated in relationship to response to the biologic agents, efalizumab and ustekinumab. A 2009 abstract publication reported that the presence of HLA-Cw6 predicted response to efalizumab therapy. No participant details, including phenotype details, details of drug administration including duration, or how response to treatment was assessed were provided.
An Italian cohort of 51 patients with moderate-to-severe chronic plaque psoriasis treated with at least one ustekinumab injection underwent HLA genotyping and evaluation of response to treatment at or after 3 months from initial injection. An association between the presence of HLA-Cw*0602 and ustekinumab response was reported, with higher rates of response in terms of PASI 75 at 4, 12, and 28 weeks of therapy (at week 28, 96.3% patients with a positive response reached PASI 75 vs 72.7% of patients with a negative response P <.008). There was no listing of inclusion or exclusion criteria, specifically, no mention of psoriasis phenotype for included participants or an inclusion of only participants with a certain qualifying psoriasis severity index. Although the presence of HLA-Cw*0602 is known to be more strongly associated with type I psoriasis, age of onset of psoriasis for the cohort was listed with standard deviation and range (22.5 ± 11.3; 1–59). Participants had a washout period from other biologic agents of at least 3 months, but no mention was made of preceding or concurrent systemic medication. Statistical analysis for the categorical data was performed with a simple χ 2 test, with the only report of multivariate logistical regression analysis that age of onset of psoriasis was not related to response to ustekinumab. There is no discussion of the high rates of ustekinumab response in HLA-Cw*0602–negative patients nor a comparison of investigation data with published ustekinumab clinical trial response data. In a cohort of Chinese patients with psoriasis treated with ustekinumab (n = 66), significantly more HLA-Cw*0602–positive patients achieved a PASI 50, 75, or 90 response than HLA-Cw*0602–negative patients. However, the study was at risk of significant bias, with participants selected retrospectively.
Translation of Pharmacogenomic Studies in Psoriasis
There is no convincing predictive biomarker for any drug used to treat psoriasis. Problems with methodologic quality may in part be responsible for the lack of replication present in the field of pharmacogenomics. There are several methodologic areas that may be more thoroughly considered in the planning and conducting of future pharmacogenomic studies to improve the quality of results of any one study. Furthermore, the generation of huge datasets from newer technologies and the open access of such data for effective meta-analysis are crucial to future translation of potential biomarkers.
Standardization of Approach to Pharmacogenomic Studies
Future pharmacogenomic studies in psoriasis should adhere to a standardized approach, using a checklist, which includes reference to several previously published standards ( Table 1 ). Examples already exist of the implementation of criteria to standardize studies, such as in drug-induced skin injury, in which efforts have been made to standardize phenotypes of participants. In psoriasis research, this approach is feasible by using simple clinical criteria, for example, a diagnosis of psoriasis vulgaris confirmed by a dermatologist and designation of age of onset of psoriasis vulgaris and exclusion of erythrodermic psoriasis, pustular psoriasis, and palmo-plantar pustulosis.
Checklist Item | Subgroup | Details of Minimum Requirements | Ideal Requirements |
---|---|---|---|
Study design | Study protocol | Applied to all participants, especially where study conducted at multiple sites | Protocol or details published |
Power calculation | Description of determination of sample size | Power calculation declared Declare sample size where cannot reach the required and discuss | |
Outcomes | Outcomes fully defined Where response to treatment, use of objective validated outcome measure, such as PASI at each visit Where numerous outcome measures recorded, clarify which score used as assessment of response in advance and which correlated with genomics | PASI 75 and 90 as measures of response to treatment, less than PASI 50 as nonresponse | |
Proposed laboratory studies | Defined in advance | For genetic tests, biological plausibility discussed | |
Participant characteristics | Selection of participants | Description of selection process | |
Accurate phenotyping of psoriasis participants | Consultant diagnosis of psoriasis vulgaris Exclusion of other forms of psoriasis such as erythrodermic psoriasis and associated conditions, such as palmoplantar pustulosis | Designation type I or type II psoriasis ± HLA-C type | |
Participant demographics | Participant details including presence or absence of PsA, washout from prior treatments and concurrent medication | ||
Ethnicity | Ethnicity and ancestry of participants recorded | Ethnicity and ancestry of participants recorded and accounted for in analysis | |
Methodology | Methodology | Publish full repeatable methodology | DNA studies to refer to checklist of requirements for pharmacogenetic study, to include genotyping procedures and testing for Hardy-Weinberg equilibrium |
RNA studies to include reference to minimum standard of used technology, where exists RNA studies to include details of technology used, eg, microarray chip or RNA-Seq platform, validation of microarray results using low-throughput techniques, eg, RT-PCR, assessment of RNA integrity and quality control of individual hybridizations | |||
Results and analysis | Results reporting | Consistency of reporting | Consistency of reporting of genes, gene products, and SNPs, with gene ontology listed as compliant with the HUGO Gene Nomenclature Committee and SNPs listed by accession number or reference SNP ID (rs) number |
Access to raw data | Open access to all raw data (for DNA and RNA studies) | For microarray studies, MIAME-compliant microarray data deposited in a database GEO/ArrayExpress | |
Statistical considerations | Genomic/transcriptomic considerations | RNA studies to include listing of effect size criterion applied and derivation of P values | |
Correction for multiple testing | Validated methodology |
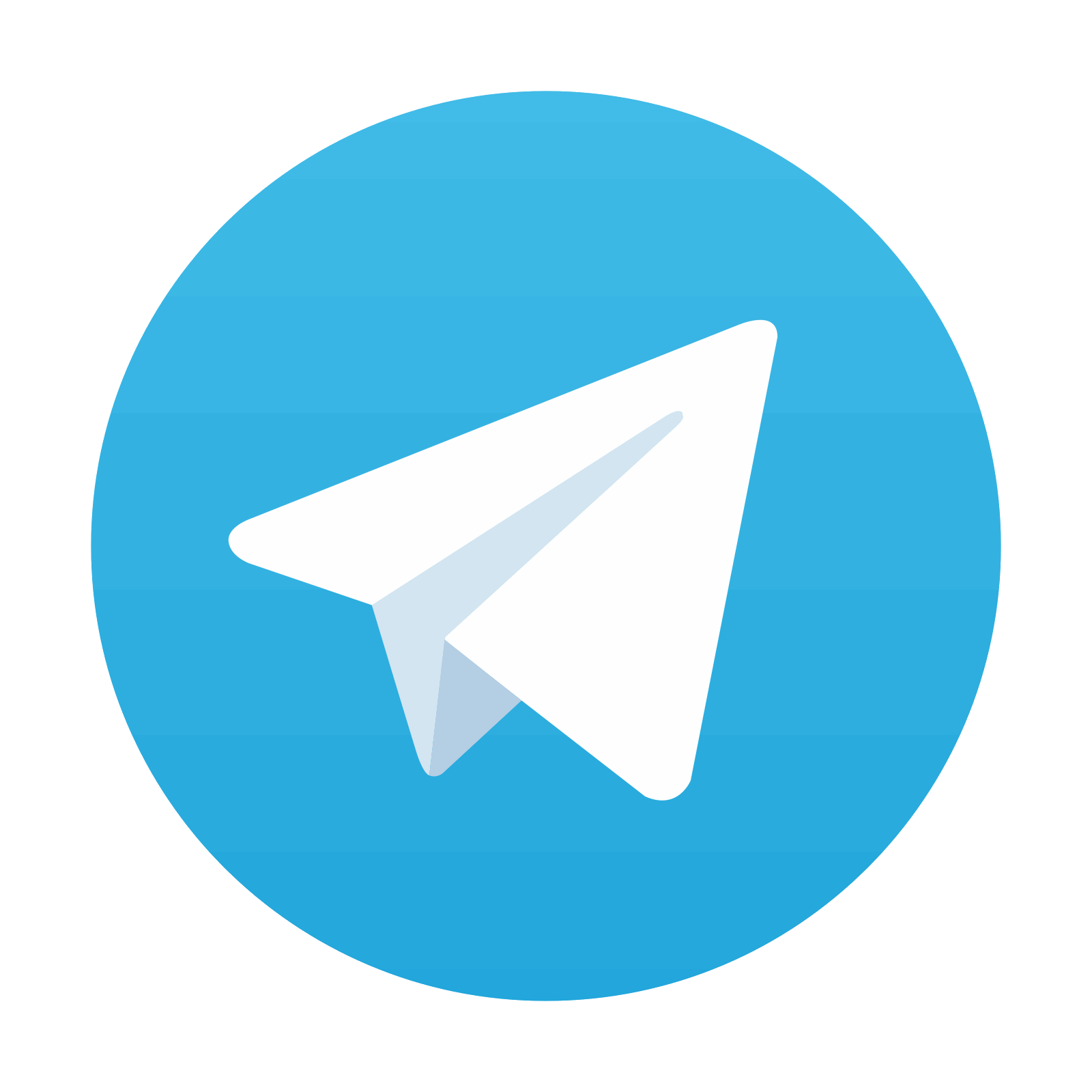
Stay updated, free articles. Join our Telegram channel

Full access? Get Clinical Tree
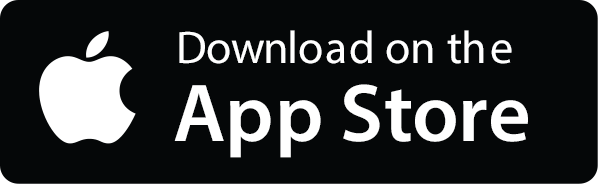
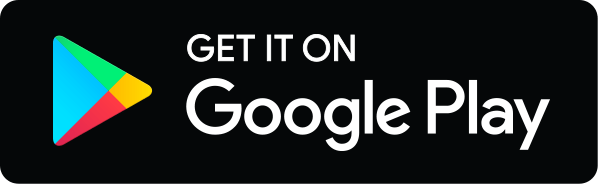
