3 Neurophysiology and Pharmacology of the Lower Urinary Tract
The two functions of the lower urinary tract are the storage of urine within the bladder and the timely expulsion of urine from the urethra. The precise neurologic pathways and neurophysiologic mechanisms that control these functions of storage and micturition are complex and not completely understood. However, an extremely important concept that is now appreciated for its application to treatment of lower urinary tract dysfunction is the principle of neuroplasticity, as it applies to the pathways and mechanisms. The nervous system is composed of varying sizes and types of nerves. They are classified generally by size. The largest, myelinated, fast-conducting A alpha nerves are sensory (afferent) nerves conveying touch, or motor (efferent) nerves activating large muscles. The smallest, nonmyelinated C nerve fibers are slow-conducting nerves that convey pain and temperature on the sensory side or act as postganglionic autonomic nerves on the motor side. The bladder afferent nerves are largely C fibers at birth, until maturation changes the afferents to A delta (lightly myelinated, small) fibers. Many now appreciate that bladder insults, such as obstruction, bladder inflammation, or spinal cord disease, affecting pathways involved in lower urinary tract function lead to neuroplastic changes in which the afferents again become C fibers as the bladder’s response to the insults. Understanding the anatomy and physiology of the basic reflex pathways and central voluntary control involved in lower urinary tract function is necessary, but appreciating the dynamic ability of the neurons to modify these pathways is essential to application of modern therapies.
GENERAL NERVOUS SYSTEM ARRANGEMENTS
The nervous system is arranged into the central and the peripheral systems. The central nervous system (CNS) includes the brain and spinal cord. Within the brain and cord, nerve cell bodies are arranged in groups of various sizes and shapes called nuclei. Fibers with a common origin and destination are called a tract; some are so anatomically distinct that they may be called fasciculus, brachium, peduncle, column, or lemniscus. Synaptic relationships in the central nervous system are very complex, with contacts occurring between axons and cell bodies, axons and dendrites, cell body and cell body, or dendrite and dendrite.
NEURAL CONTROL OF THE LOWER URINARY TRACT
Local innervation is chiefly by parasympathetic and sympathetic autonomic and peripheral somatic motor and sensory systems. A summary of the neural pathways involved in bladder filling and voiding is shown in Figures 3-1 and 3-2.
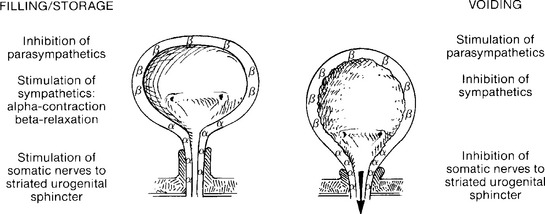
Figure 3-2 Actions of the autonomic and somatic nervous systems during bladder filling/storage and voiding.
Autonomic Nervous System
SYMPATHETIC ACTIONS ON DETRUSOR MUSCLE AND GANGLIA
The sympathetic neural pathways are as follows: Sympathetic preganglionic fibers from thoracolumbar spinal segments form white rami communicantes to synapse in the paravertebral or prevertebral sympathetic pathways, the latter predominating. Postganglionic neurons reach inferior mesenteric ganglia by the lumbar splanchnic nerves and continue through the hypogastric plexus to the presacral fascia, across the upper posterior lateral pelvic wall, 1 to 2 cm behind and below the ureter. After these neurons join the pelvic nerves, the pelvic plexus is formed, running below and medial to the internal iliac vessels overlying the anterior lateral lower rectum near the anorectal junction. The plexus spreads in the lateral wall of the upper one third of the vagina beneath the uterine artery, medial to the ureter and 2 cm inferolateral to the cervix. Within the vesicovaginal space, the plexus supplies the upper vagina, bladder, proximal urethra, and lower ureter. Sympathetic preganglionic neurons generally use acetylcholine neurotransmitter acting on nicotinic receptors. The sympathetic postganglionic fibers are primarily noradrenergic, with norepinephrine being the chief neurotransmitter. Norepinephrine stimulation of β adrenergic receptors located in the bladder body causes relaxation of the smooth muscle, as the β receptors are stimulated by lower norepinephrine doses. Stimulation of α1 receptors in bladder base and urethral smooth muscle by higher doses of norepinephrine causes muscle contraction. Norepinephrine also acts as a neurotransmitter at the parasympathetic ganglia, and α-adrenergic receptors, when stimulated, depress parasympathetic pelvic ganglion transmission by suppression of presynaptic cholinergic neurotransmitter release. Thus, sympathetic relaxation of detrusor body smooth muscle, contraction of bladder base and urethral smooth muscle, and depression of parasympathetic ganglionic transmission, all act to promote urine storage. Other sympathetic modulators of neurotransmission include purinergic, peptidergic, and nitrergic factors.
PARASYMPATHETIC ACTIONS: DETRUSOR
At the detrusor muscle, postganglionic, parasympathetic detrusor nerve fibers diverge and store neurotransmitter agents in axonal varicosities called synaptic vesicles. The agent is diffused to neuromuscular bundles of 12 to 15 smooth muscle fibers enclosed in a collagen capsule that acts similarly to the tendon insertion of a muscle. Stimulating electrical pulses produce two episodes of depolarization, suggesting the release of two neurotransmitters. The chief neurotransmitter is cholinergic, with muscarinic receptors, and the second neurotransmitter is noncholinergic and nonadrenergic. This observation accounts for detrusor atropine resistance. The studies of Burnstock et al. in 1972 demonstrated that adenosine triphosphate (ATP) was the mediator of these noncholinergic, nonadrenergic contractions. Variability is present between species, and some studies suggest that the purine (ATP) pathway responses lead to more rapid bladder contractions, perhaps being useful in animals that use short, quick squirts of urine for property marking.
SMOOTH MUSCLE OF TRIGONE AND URETHRA
The proximal urethral smooth muscle is rich in α-adrenergic receptors responsive to norepinephrine neurotransmitter. Acetylcholine, substance P, vasoactive intestinal polypeptide, and histamine are all additional potential transmitters in the urethra. Nitric oxide (NO) is prominent in the parasympathetic postganglionic innervation of the urethra, and exogenous NO or parasympathetic nerve stimulation relaxes urethral smooth muscle. Parasympathetic stimulation has been thought by Khanna (1981) to cause contraction of urethral smooth muscle, using acetylcholine neurotransmission and muscarinic receptors. However, McGuire (1978) thought this increase in urethral “resistance” was due to simple transmission of intravesical pressure. He demonstrated, by diverting the urinary stream, that pelvic nerve stimulation caused urethral smooth muscle relaxation. The relaxation effect was blocked by propranolol, indicating a β-adrenergic response.
SKELETAL MUSCLE OF LOWER URINARY TRACT: SOMATIC INNERVATION
In the lower urinary tract, the somatic system involves skeletal muscle in the lower urinary tract outlet. The neuronal cell bodies for the urethral sphincter and for the distal periurethral striated muscles and pelvic floor muscles are located in Onuf’s somatic nucleus in the lateral aspect of the anterior horn of the gray matter of the sacral spinal cord from S2 to S4. This nucleus gives rise to the pudendal nerve, which is classically thought to provide the efferent innervation of the striated sphincter. Thor (2004) has shown that serotonin and norepinephrine enhance the effects of glutamate, the primary excitatory neurotransmitter for pudendal motor neurons. The pudendal nerve, using acetylcholine, activates nicotinic cholinergic receptors to contract the rhabdosphincter.
The exact neuropathways supplying the urethral sphincter skeletal muscle are controversial. The proximal intramural component of the striated urogenital sphincter muscle (urethral sphincter, rhabdosphincter) is variably innervated by somatic efferent branches of the pelvic nerves, a component of the pelvic plexus. Elbadawi (1983) believed this intramural component to have somatic and autonomic (both cholinergic and adrenergic) innervation. However, the more distal periurethral striated muscles (compressor urethra and urethrovaginal sphincter) are innervated by the pudendal nerve, as is the skeletal muscle of the external anal sphincter and perineal muscles.
The pudendal nerve passes between the coccygeus and piriformis muscles, leaves the pelvis through the greater sciatic foramen, crosses the ischial spine, and reenters the pelvis through the lesser sciatic foramen. Here the nerve accompanies the pudendal vessels along the lateral wall of the ischiorectal fossa in a tunnel formed by a splitting of the obturator fascia, called Alcock’s canal. At the perineal membrane, the nerve divides into the inferior rectal nerve, supplying the external anal sphincter, the perineal nerve, and the dorsal nerve to the clitoris (see Fig. 2-8). The perineal nerve splits into a superficial branch to the labia and a deep branch to the periurethral striated muscles. The branching of the pudendal nerve shows considerable variation.
The urethral sphincter muscle is an integral part of the urethral wall and is made up of all slow-twitch (type 1) fibers. The periurethral muscles (compressor urethra and urethrovaginal sphincter) are composed of mostly slow-twitch fibers with a variable concentration of fast-twitch (type 2) fibers. These fibers combine to provide constant tonus, with emergency reflex activity mainly in the distal half of the urethra.
Sensory Innervation
Urethral sensation is carried principally by the pudendal nerve, although the pelvic nerve also contributes (Fig. 3-3). Urethral smooth muscle sensory innervation, like that of the detrusor, has both a contralateral and an ipsilateral supply.