Fig. 16.1
The degree of stratum corneum removal after treatment with microdermabrasion. (a) Partial stratum corneum removal, (b) complete stratum corneum removal with intact epidermis, and (c) complete epidermis removal. The depth of the tissue removed depends on the pressure, crystal particle flow rate, and exposure time
Removing the stratum corneum using microdermabrasion has been shown to increase the skin’s permeability to water-soluble molecules that range in size from 130 D to 6 kD in vitro and in vivo (Andrews et al. 2011a, 2013; Fang et al. 2004a; Gill et al. 2009; Lee et al. 2003, 2006). One in vitro study showed that microdermabrasion increased the delivery of sulforhodamine, a low molecular weight dye, by 430-fold as compared to intact untreated skin (Andrews et al. 2013). While in vitro experiments have been successful in demonstrating that microdermabrasion can be used to deliver water-soluble compounds, in vivo studies have evaluated the efficacy of drugs delivered using microdermabrasion. An in vivo study that delivered insulin to diabetic rats after microdermabrasion showed that the blood glucose was significantly lowered in rats whose skin was microdermabraded compared to control animals and had a lower mortality throughout the experiment (Andrews et al. 2011a).
In the following sections, we describe the mechanism of microdermabrasion, the parameters that affect microdermabrasion, its biophysical effects on the skin, in vitro and in vivo drug delivery studies using microdermabrasion, challenges in the field, and conclusions.
16.2 Mechanism and Factors Affecting Microdermabrasion
16.2.1 Mechanism of Microdermabrasion
Microdermabrasion involves impingement of sharp, multifaceted hard crystalline particles on the skin surface to microscopically abrade the skin layers. The mechanism is schematically described in Fig. 16.2. Typically, aluminum oxide particles measuring approximately 200 μm are used. The flow of particles is achieved by using a vacuum pump, which aspirates fresh crystals from a clean crystal container and bombards them at the skin via a disposable hand tip attachment (Fig. 16.3a, b). The hand tip contains an inlet port for the particles to enter, a small opening (Fig. 16.3c) that contacts the skin to allow the user to direct the particles at the skin, and an outlet port for removal of spent particles. A scanning electron micrograph of the alumina particles is shown in Fig. 16.3d. The particles achieve high kinetic energy due to the pressure differential between the outlet and inlet ports, which combined with the sharp particle edges can help cut through skin layers creating microfragments of the tissue. These microfragments and the waste particles are aspirated into a waste container via the outlet port.



Fig. 16.2
Schematic describing microdermabrasion of the skin. Sharp multifaceted crystal particles hit the skin surface. The kinetic energy of the microparticles coupled with their sharp edges causes micro-cutting action to abrade layers of the skin. The microparticles after hitting the skin and the abraded tissue fragments so produced are removed under vacuum (Image not to scale)

Fig. 16.3
Microdermabrasion equipment. (a) Photograph of DermaMed MegaPeel® microdermabrasion device. Dotted arrows indicate direction of microparticle flow. (b) Photograph of hand tip applied to a black paper while equipment is operating. (c) Photograph of hand tip showing an 8 mm diameter opening that touches the skin during microdermabrasion. (d) Scanning electron micrograph of aluminum oxide microparticles
Using microdermabrasion the stratum corneum layer can be completely removed and a drug or vaccine patch can be applied to achieve delivery via the skin.
In an alternate approach called microscissioning, instead of using vacuum, high-pressure gas has been used as a carrier fluid to bombard sharp microparticles onto the skin to achieve abrasion (Herndon et al. 2004). In this study in vivo lidocaine delivery and glucose sampling were demonstrated.
16.2.2 Equipment Manufacturers
Multiple manufacturers offer microdermabrasion equipment catering to the cosmetic industry. Some manufacturers are (i) DermaMed Solutions, 394 Parkmount Road, PO Box 198, Lenni, Pennsylvania, USA; (ii) Mattioli Engineering Corporation, 8300 Greensboro Drive, Suite 800, McLean, Virginia, USA; and (iii) Bell Products, Inc., 27136 Burbank, Foothill Ranch, California, USA. The authors have experienced using the MegaPeel® Gold Series microdermabrasion equipment made by DermaMed. Figure 16.3 shows the MegaPeel device and the associated hand tip used for microdermabrasion.
16.2.3 Operating Parameters that Affect Microdermabrasion
16.2.3.1 Pressure
The vacuum pressure in the microdermabrasion device provides multiple functions. Importantly, the vacuum pressure provides a driving force to create a continuous flow of microparticles to bombard the skin surface and to aspirate the abraded skin microfragments into a waste container. The vacuum pressure also controls the kinetic energy of the particles that hit the skin surface, thus providing a means to modulate microdermabrasion to suit different skin types. Various in vitro and in vivo studies have demonstrated the need to optimize the operating pressure to different skin types. A study by Andrews et al. (2011c) using porcine skin in vitro demonstrated that vacuum pressure (30–60 kPa) does not significantly affect the degree of tissue removal from porcine skin. In contrast a study by Gill et al. (2009) performed in vivo in humans and rhesus macaques showed a dependence of stratum corneum and viable epidermis removal on pressure. Figure 16.4 reproduced from this study shows that while at a vacuum pressure of 30 kPa (3 s exposure), there is little to no removal of the stratum corneum, an increase in vacuum pressure to 45 kPa (exposure time of 3 s) results in complete abrasion of the stratum corneum and the viable epidermis. A similar effect of pressure is seen in rhesus macaques (Gill et al. 2009). This species-dependent effect of vacuum pressure may be due to species-dependent differences in adhesion between the viable epidermis and the dermis. As seen in Fig. 16.4, microblisters can be formed at high vacuum pressures for human and rhesus macaque skin (Gill et al. 2009) and hairless rats (Zhou and Banga 2011). Microblisters may represent an early stage in the formation of macroscopic suction-based blisters, a technique which is clinically used to harvest the epidermis for transplantation or to study inflammation of the skin (Gupta et al. 1999; Gupta and Kumar 2000; Suthar et al. 2010). Thus, at high vacuum pressures, the viable epidermis may simply be tearing away from the dermis rather than being removed by particle-based abrasion effects. Microblisters were however not seen in pig skin (Andrews et al. 2011a, b, c). It is thus critical to optimize the microdermabrasion vacuum pressure for use in humans versus other laboratory animal models.


Fig. 16.4
Mobile- and stationary-mode microdermabrasion in humans. (a) Bright-field images of hematoxylin- and eosin-stained human skin sections from biopsies obtained from an untreated control site. (b, c) Sites exposed to mobile mode of microdermabrasion with 40 kPa vacuum pressure and seven passes. (d, e) Sites exposed to stationary-mode microdermabrasion with an exposure time of 3 s and a vacuum pressure of 30 kPa (d) and 45 kPa (e). Dotted rectangles indicate areas of selective yet full-thickness removal of the stratum corneum, double–lined arrows point to microblisters, and single–lined arrows point to residual aluminum oxide particles (Reproduced from Gill et al. 2009 with permission from Elsevier)
16.2.3.2 Flow Rate
The flow rate of the device as a function of pressure is dependent on the device used and can be controlled via an external knob, which internally controls a valve in the flow path of the microparticles. In a study that used a MegaPeel® (DermaMed Solutions, LLC, USA) microdermabrasion device, the particle flow rate was found to be constant at all times at a specific pressure (Andrews et al. 2011a, b, c). Figure 16.5 shows the flow rate at a vacuum pressure of 40 kPa at three, six, and nine turns of the microparticle flow rate knob. Three and six turns of the flow rate knob induced a mass flow rate of 0.36 ± 7.70 × 10−3 g/s and 0.21 ± 2.1 × 10−2 g/s, respectively. The microparticle flow rate at nine turns was zero. Higher flow rates resulted in more tissue removal.


Fig. 16.5
Particle flow rate as a function of time. Particle flow rate at a vacuum pressure of 40 kPa at three, six, and nine turns of the particle flow rate knob is shown. Three turns of the knob is the most aggressive and nine is the least. Abrading skin with a higher flow rate results in a greater amount of tissue removal
16.2.3.3 Stationary Versus Mobile Hand Tip
The hand tip can be held stationary (stationary mode), or it can be moved on the skin surface at a fixed speed (mobile mode). In either case, the time of contact can affect rate and degree of microdermabrasion (depth of skin abraded). In the mobile mode, the speed of hand tip movement and the number of times it is repeatedly moved across the treatment area can further modulate the degree of microdermabrasion.
The effect of stationary vs mobile mode of the hand tip has been compared in vitro (Andrews et al. 2011a, b, c) and in vivo in humans, rhesus macaques, and hairless rats (Gill et al. 2009; Zhou and Banga 2011). The in vitro and in vivo studies offer similar conclusions. The different parameters investigated in the two in vivo studies are summarized in Table 16.1.
Table 16.1
Conditions of microdermabrasion investigated in vivo for mobile and stationary modes of hand tip operation
Species (reference) | Mobile mode | Stationary mode | Conclusions |
---|---|---|---|
Human (Gill et al. 2009) | 40 kPa – seven passes at 13 mm/s | 30 kPa and 45 kPa each for 3 s application time | Mobile mode: thickness of the stratum corneum layer removed increases with vacuum pressure and number of passes. At high pass numbers, the viable epidermis can also be removed Stationary mode: thickness of the stratum corneum layer removed increases with vacuum pressure and application time. At high vacuum pressures, the viable epidermis can separate from the dermis creating “microblisters” |
Rhesus macaque (Gill et al. 2009) | 25 kPa – 100, 200, and 300 passes at 40 mm/s 50 kPa – 10, 30, 50, 80, and 100 passes at 40 mm/s | 30 kPa and 50 kPa each for 3 s and 6 s application time | |
Hairless rat (Zhou and Banga 2011) | 50.8 kPa – 1, 3, 5, 10, and 20 passes at 3 s/pass | 50.8 kPa for 3, 5, or 10 s |
Using histological analysis of the skin biopsies from humans and macaques, Gill et al. (2009) quantified the effect of different microdermabrasion operating parameters on skin layers. It was found that in the mobile mode, the degree of tissue removal increases with vacuum applied, number of passes, and the speed of tip movement, while in a stationary mode, the degree of skin removal increases with an increase in vacuum pressure and time of application. Figure 16.4 shows the representative effects observed at the different microdermabrasion conditions in humans. In stationary mode, microblisters (separation of the viable epidermis from the dermis) were also observed (Fig. 16.4, double-lined arrows), which are undesirable for most drug delivery applications. A study by Fujimoto et al. has proposed a model to predict the flux of drug across the skin based on the different microdermabrasion conditions (Fujimoto et al. 2005).
16.2.3.4 Microdermabrasion Masks
Microdermabrasion has proven to be a safe procedure for routine cosmetic applications wherein the stratum corneum is only partially removed from the skin. In contrast, for drug delivery applications, it is desirable to remove full thickness of the stratum corneum. However, for safety concerns full-thickness removal of the stratum corneum should be limited to microscopic islands rather than bulk areas of the skin. In principle because the stratum corneum is the major rate-limiting barrier, even if the stratum corneum can be removed from microscopic areas of the skin, a significant enhancement in skin permeability should be observed. Indeed creation of discrete micron-sized holes in the stratum corneum using microneedles (Gill and Prausnitz 2007a, b; Wermeling et al. 2008), lasers (Fang et al. 2004b), and thermal ablation (Lee et al. 2011) has been shown to increase skin permeability by orders of magnitude. To create such discrete and localized areas of microdermabrasion, a mask with micron-sized holes has recently been developed (Andrews et al. 2011a, b, c). This mask when placed between the skin and the hand tip protects the skin from particles except in areas where the skin is exposed via the mask holes. The masks can be fabricated using metals or polymers and can have different hole sizes. An example of a mask used with microdermabrasion is shown in Fig. 16.6a. The mask is fabricated out of stainless steel and has holes that are 250 μm in diameter. The abraded areas of the skin were stained with a dye and are shown in Fig. 16.6b. A histological section of the skin of one of the sites of abrasion is shown in Fig. 16.6c. Using a mask allows greater control over the area, amount, and depth of skin abrasion and improves reproducibility. The mask approach has been used in vivo to achieve a therapeutically effective delivery of insulin in diabetic rats (Andrews et al. 2011a).


Fig. 16.6
Mask for microdermabrasion. (a) Photomicrograph of stainless steel mask array with 250 μm diameter holes. (b) Photomicrograph of porcine skin surface after abrasion through the mask. Green dye was used to stain the areas of abrasion. (c) Photomicrograph of a histological section of the skin with arrows pointing to the area of abrasion
16.3 Biophysical and Molecular Effects of Microdermabrasion
16.3.1 Loss of Skin Barrier Property
A major effect of microdermabrasion on the skin is the partial or complete removal of the stratum corneum, which leads to reduction in barrier property of the skin. The conventional methods to probe skin integrity can be used to assess skin barrier reduction and healing kinetics following microdermabrasion.
16.3.1.1 Transepidermal Water Loss
Transepidermal water loss (TEWL) is a measure of the amount of water permeating from the skin outward toward the surroundings. TEWL increases with loss of skin integrity and thus offers a convenient method to study microdermabrasion. TEWL has been used in different microdermabrasion studies (Lee et al. 2003; Rajan and Grimes 2002; Zhou and Banga 2011) and has been shown to increase and to correlate with the extent of microdermabrasion performed.
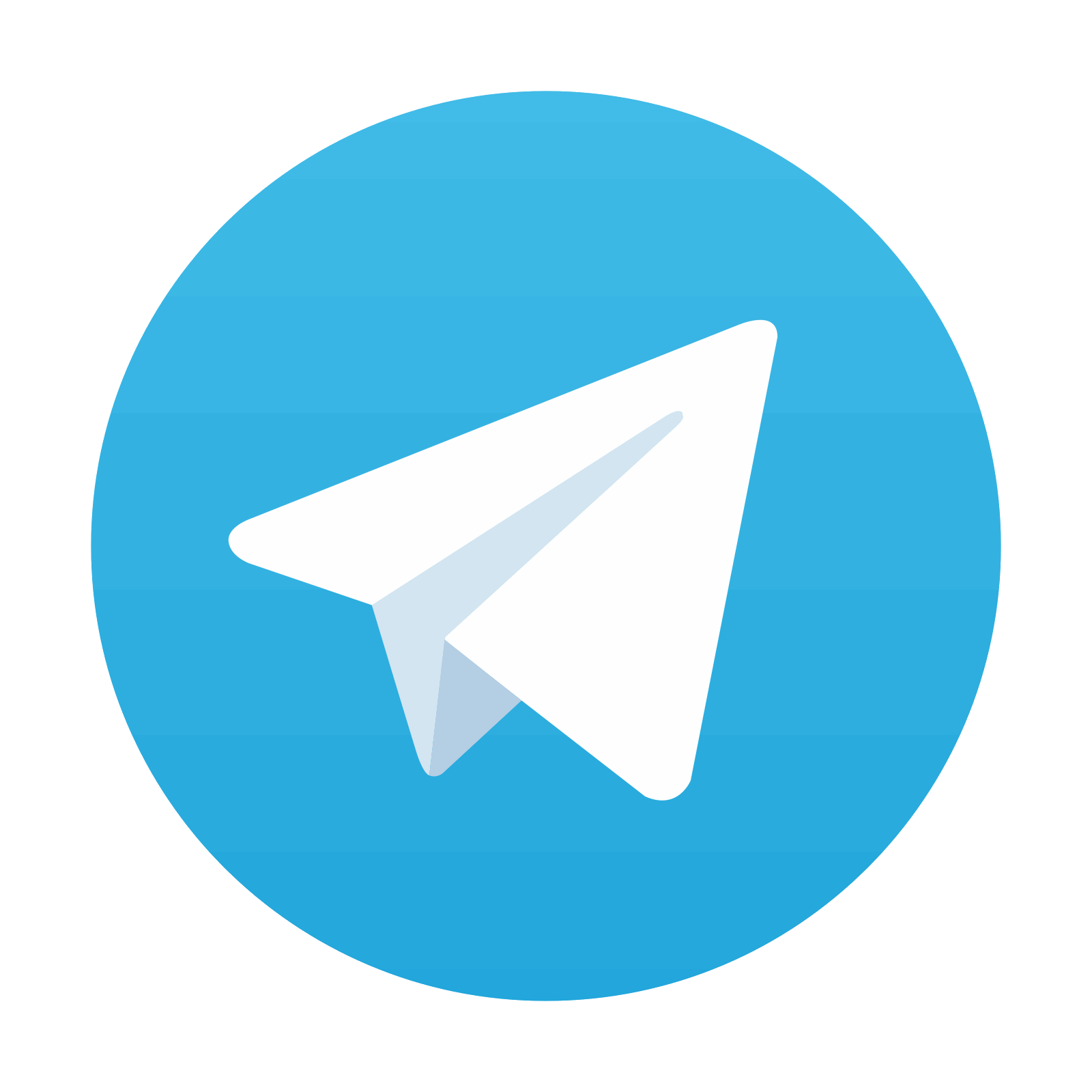
Stay updated, free articles. Join our Telegram channel

Full access? Get Clinical Tree
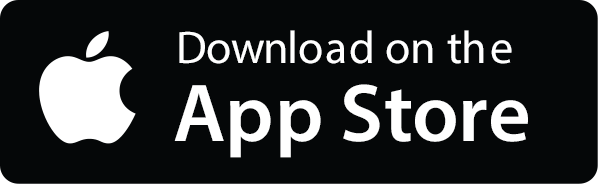
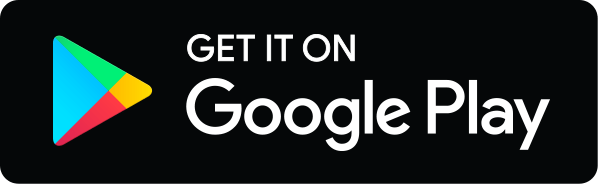