Fig. 6.1
The microbiota organ
Different species and quantities of bacteria are found at different points along the digestive tract. The stomach pH is deadly for most microbes, the acid-resistant Lactobacillus and Streptococcus are predominant and account for 102–3/ml. In the duodenum and jejunum up to 104–5 bacteria/ml (Lactobacillus, Escherichia coli, Enterococcus faecalis) can be found despite the toxicity of bile and pancreatic secretions. The number of bacteria rises in the ileum (107 bacteria/ml) and in the colon (1012 bacteria/ml). The large intestine contains the largest number of bacteria, and is characterized by a complex and dense microbial community mainly made up of anaerobic species [3].
Three bacterial divisions, the Firmicutes (Gram-positive), Bacteroidetes (Gram-negative), and Actinobacteria (Gram-positive) dominate the adult human gut microbiota. The Firmicutes is the largest bacterial phylum (60 %) and contains more than 200 genera, including Lactobacillus, Mycoplasma, Bacillus, and Clostridium; the Bacteroidetes (20 %) include about 20 genera; the Actinobacteria (Gram-positive) are frequently missed by RNA gene sequencing and can be detected only by fluorescent in situ hybridization (FISH). It is worth noting that human species and murine species have similar microbiota composition [4].
Newborn babies are germ free. The development of the gut microbiota starts at birth and its composition changes substantially at three stages in life: from birth to weaning; from weaning to attaining a “normal” diet; during old age. Sterile inside the uterus, the newborn’s digestive tract is quickly colonized by microorganisms from the mother (vagina, feces, skin, breast, etc.) and from the environment in which the delivery takes place. Nutrition is a driving factor in shaping gut microbiota composition and its functional maturation, from birth to adulthood. From the third day, the composition of the intestinal flora is directly dependent on how the infant is fed: in breastfed babies, compared to infant formulas nourished babies, gut microbiota is dominated by Bifidus bacteria. Breast milk is rich in oligosaccharides, fermented in the distal gut and promoting the growth of beneficial microbes as bifidobacteria. At the age of 4 years, the gut microbiota in host individuals has fully matured.
Transformation to the adult-type microbiota is triggered by multiple host and external factors. Carnivore microbiomes are enriched in protein degradation genes, while herbivore microbiomes are enriched in genes necessary to break down starch, thus, a Prevotella-type community is associated with fiber intake and a Bacteroides-type community with high protein intake.
After transformation to the adult type and until the seventh decade, the gut microbiota remains remarkably constant. However, the inter phyla numerical proportions may have important fluctuations around an individual core of stable colonizers. Microbes in the human gut undergo selective pressure from the host as well as from microbial competitors. This typically leads to a homeostasis of the ecosystem in which some species occur in high and others in low abundance (the “long-tail” effect), with some low abundance species, like methanogens, performing specialized functions beneficial to the host [5, 6].
6.1 Functions of Microbiota
The gut microbiota has multiple functions related not only to the gastro-intestinal system but also to the central and autonomic nervous system, the immune system. Alterations of the microbiota may lead to anxiety and depressive status: a significant correlation between gut inflammatory status and anxiety traits has been documented [7, 8].
Although the most abundant molecular functions generally trace back to the most dominant species, some of them may be contributed to primarily by low-abundance genera. Because of this factor and of additional ones such as the elevated number of species, the numerical variations induced by host and external factors, the multiple functions, investigations on microbiota are difficult and necessitate specific and sophisticated methods to answer specific questions: nucleic acid studies (which are they?), metatranscriptomics, metaproteomics, metabolomics (what are they doing?), and metagenomics. The study of “clusters” of functionally related groups is of help in elucidating microbiota’s role [5, 9].
6.2 Microbiota and Obesity
The gut microbiota is highly vulnerable to changes in the gut microenvironment. Under normal conditions, commensal microbes and their hosts enjoy a symbiotic relationship. A qualitative and/or quantitative imbalance in the intestinal flora, in their metabolic activities or changes in their local distribution leads to a condition known as dysbiosis where microbial imbalance exerts adverse effects on the host. A pivotal role of gut microbiota has been evidenced in the development of gastrointestinal diseases, such as Clostridium difficile infection (CDI), other inflammatory bowel diseases, and colorectal cancer [10, 11].
Recent studies have highlighted a role for gut microbiota dysbiosis in the genesis of obesity and of the metabolic syndrome. There are a number of linking arguments: dysbiosis occurs in diabetic and obese versus lean individuals; some components of the gut microbiota (e.g., lipopolysaccharides, LPS) play a harmful role in obesity and diabetes; the diet takes part in the modulation of the gut microbiome and microbiota composition/function in obesity and metabolic syndrome [12–20].
In obese subjects (human and murine), the gut microbiota has a higher proportion of the phylum Firmicutes and a lower proportion of the phylum Bacteroidetes, the reverse occurs in lean subjects [15, 16]. A direct relationship between Bacteroidetes abundance and body weight has been demonstrated.
Gnotobiology, which is the selective colonization of germ-free animals, has given insight into the role covered by the commensal microbiota in a series of patho-physiological mechanisms, such as obesity, insulin resistance, and diabetes.
The host-bacterial association possesses a beneficial mutuality enhancing the host digestive and metabolic efficiency and ensuring a stable metabolic supply for the microorganisms.
In fact, germ-free mice show a less body fat content than conventional raised mice. High-fat Western-type diet in conventional mice induces obesity and insulin resistance. When given the same diet, germ-free mice are resistant to obesity and to insulin resistance. The effect is FIAF (fasting-induced adipose factor) dependent (Fig. 6.2) [12, 17].
Colonization of germ-free mice with conventional microbiota leads to a 60 % increase in the body fat that likely depends on the greater capacity to extract energy from food which, otherwise, would be only partially utilized, together with an increased insulin resistance [12, 13].
In fact, the microbiota metabolizes complex carbohydrates present in the fibers, which could not be otherwise digested, to oligo- and mono-saccharides, which in turn are fermented to short-chain fatty acids, including butyrate, propionate, and acetate. Short-chain fatty acids are absorbed in the colon, where butyrate provides energy for colonic epithelial cells. Through the portal circulation acetate and propionate reach the liver and peripheral organs, where they become substrates for gluconeogenesis and de novo lipogenesis, thus determining the accumulation of triglycerides in the liver [12, 18, 21] (Fig. 6.3).
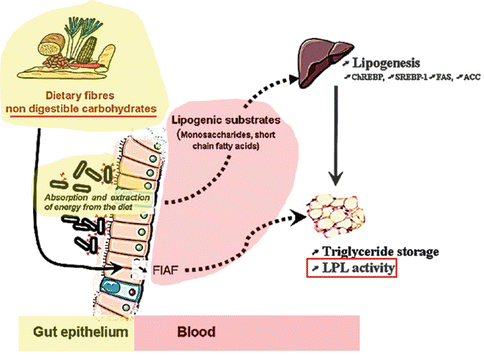
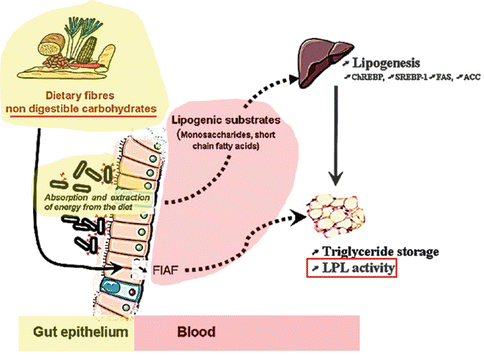
Fig. 6.3
In obese individuals, the microbiota is capable of extracting extra energy from the food by fermenting, otherwise indigestible, carbohydrates to mono saccharides and to short-chain fatty acids (SCFA) thus increasing fat storage [12, 21]. FIAF: fasting-induced adipose factor; LPL: Lipoprotein Lipase (from Cani and Delzenne [21] modified)
Besides representing an energy source, short-chain fatty acids modulate intestinal gene expression by inhibiting the enzyme histone deacetylase and regulate energy metabolism through G-protein-coupled receptors (GPCRs), such as GPR41 or GPR43 [17, 18].
The effect of increasing body weight is enhanced if germ-free mice are colonized with flora derived from obese rather than from lean donors (Fig. 6.4) [14].
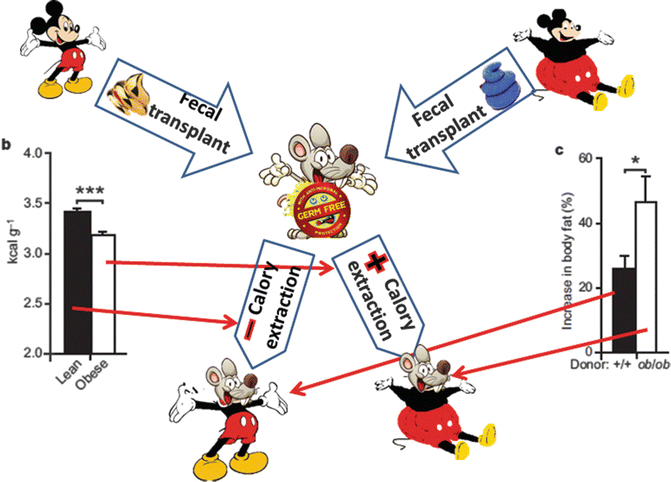
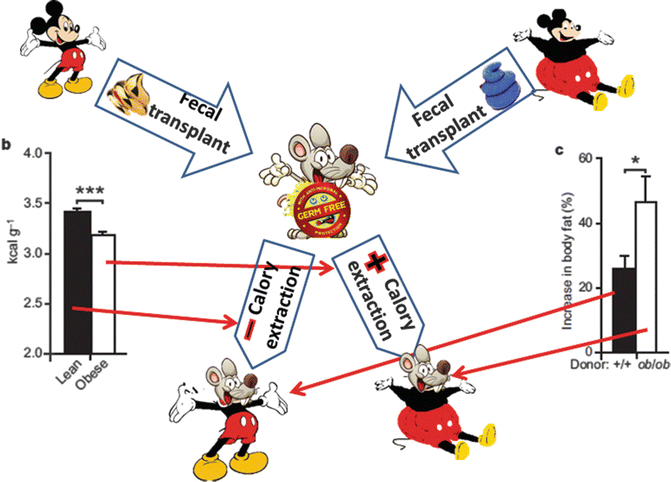
Fig. 6.4
Germ-free mice colonized with microbiota derived from obese donors gain more weight than germ-free mice colonized from lean donors [14]
Furthermore, germ-free mice “humanized” by microbiota from human donors become sensible to western-type high-fat diet (Fig. 6.5) [22].
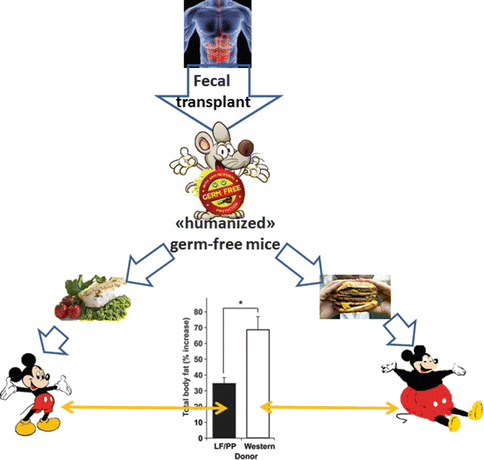
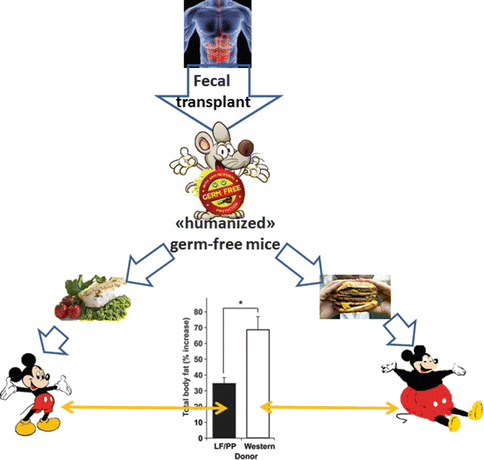
Fig. 6.5
Germ-free mice “humanized” by microbiota from human donors become sensible to western-type high-fat diet [22]
In addition, obese mice have an inversion of the proportional intestinal content of Bacteroidetes and Firmicutes with a net reduction of the former and an increase of the latter [15, 16]. Interestingly, germ-free animals show a decreased lipogenic-related gene expression which can contribute to their leaner shape [13].
6.3 Microbiota and Metabolic Syndrome
High-fat diet induces significant changes in the composition of the gut microbiota by decreasing the population of bifidobacteria. Decreased bifidobacteria determine loosening of the tight junctions between cells thus being responsible for increased gut permeability to a major component of the outer membrane of Gram-negative bacteria, the LPS (Fig. 6.6), an endotoxin that possesses pro-inflammatory actions and blunts insulin signaling [18], ensuing low-grade inflammation (metabolic endotoxemia), diminished insulin sensitivity and, finally, metabolic syndrome.
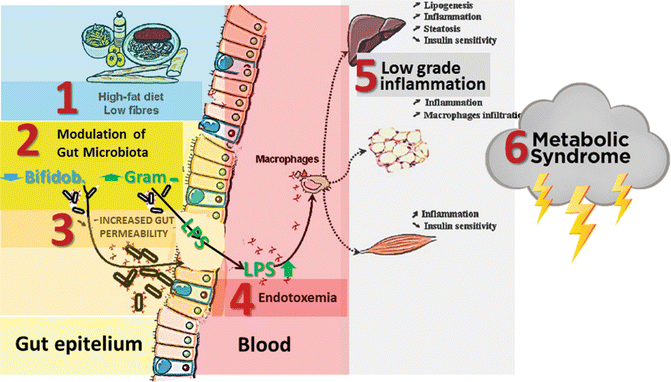
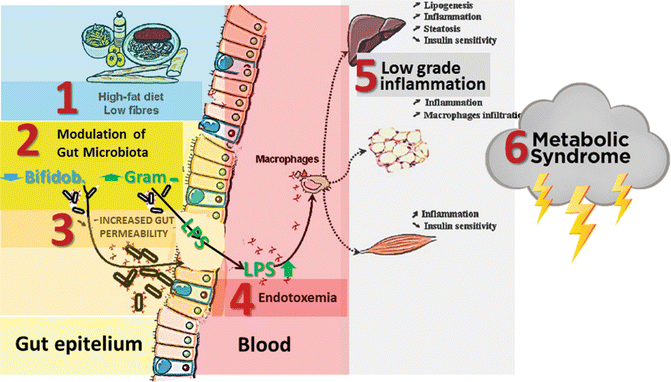
Fig. 6.6
Microbiota modulation is responsible for increased gut permeability to lipopolysaccharides (LPS), thus inducing endotoxiemia, low-grade inflammation and metabolic syndrome (From Cani and Delzenne [21] modified)
Correlation between LPS endotoxemia and fasting insulinemia, glycemia, and triglycerides has been demonstrated in type 2 diabetic patients [23].
6.4 Microbiota and Surgery
Bariatric surgery results in weight loss, reduced adiposity, and improved glucose metabolism, not simply attributable to decreased caloric intake or absorption. Gut microbiota share some of these effects.
Surgery induces changes in environmental and systemic factors, as well as in the anatomy of the digestive tract, all of which might have an effect on the composition of the gut microbiota [24].
Acid production: the diminished acid secretion, because of gastric resection (sleeve gastrectomy [SG], bilio-pancreatic diversion [BPD]) or gastric bypass [GBP]), determines marked changes in the intestinal pH that affect genus and species relative proportions of the intestinal flora rather than the overall cell number. Achlorhydria is associated with an increased number of Gram-positive bacteria and with modifications of microbiota ecology in the lower gastrointestinal tract: microbial community composition and short-chain fatty acid production [25].
Food transit time: in SG and in GBP the food reaches rapidly in the small intestine stimulating the secretion of entero-hormones (PYY, GLP-1). In malabsorption operations, portions of the small bowel are bypassed and undigested food reaches the colon.
Entero-hepatic cycle: in operations with Roux limb (GBP, BPD) the bile acids follow a different route from that of food. GBP seems to be associated with increased primary bile acids in the blind loop and secondary bile acids further down in the gastrointestinal tract, both of which have antimicrobial properties.
Antimicrobial prophylaxis for patients undergoing bariatric surgical procedures determines an acute although transient effect on microbiota composition.
Time spent masticating: in all procedures with a restrictive component, increased chewing time produces larger quantities of saliva and promotes gastric secretion and motility.
Food choices and preferences: after bariatric surgery diet changes in terms of quantity and quality. From soft diet in the first weeks, the patients switch gradually to a regular diet but with foods different from those consumed before surgery.
The mechanisms linking rearrangement of the gastrointestinal tract to the metabolic outcomes are largely unknown, a role for microbiota has been advocated.
Very few studies report the effect of bariatric surgery on the microbiota composition. To date only four studies in humans and five studies in animals have been reported (Table 6.2).
Table 6.2
Literature overview on bariatric surgery and microbiota
Author | Year | Subjects | N | Type of surgery |
---|---|---|---|---|
Zhang et al. [26] | 2009 | Humans | 9 | GBP |
Furet et al. [27] | 2010 | Humans | 43 | GBP |
Graessler et al. [28] | 2013 | Humans | 6 | GBP |
Kong et al. [31] | 2013 | Humans | 15 | GBP |
Li et al. [29] | 2011 | Rats | GBP | |
Li et al. [31] | 2011 | Rats | GBP | |
Liuo et al. [32] | 2013 | Mice | GBP | |
Osto et al. [44] | 2013 | Rats | GBP | |
Ryan et al. [33] | 2014 | Mice | SG |
In 2009, Zhang et al. were the first to report on the effect of GBP on gut microbiota in man [26]. They examined microbial 16S rRNA genes from PCR amplicons by using the pyrosequencing technology to compare the microbial community structures of nine individuals, three normal weight, three morbidly obese, and three post-GBP. In normal-weight and in obese individual Firmicutes were dominant, in GBP patients Firmicutes were significantly decreased with a proportional increase of Gammaproteobacteria. Most importantly, in obese subjects, the population number of Archaea hydrogen utilizing H+ extracted from indigestible polysaccharides and thus increasing energy uptake, was higher than in normal weight subjects and in subjects with GBP (Fig. 6.7
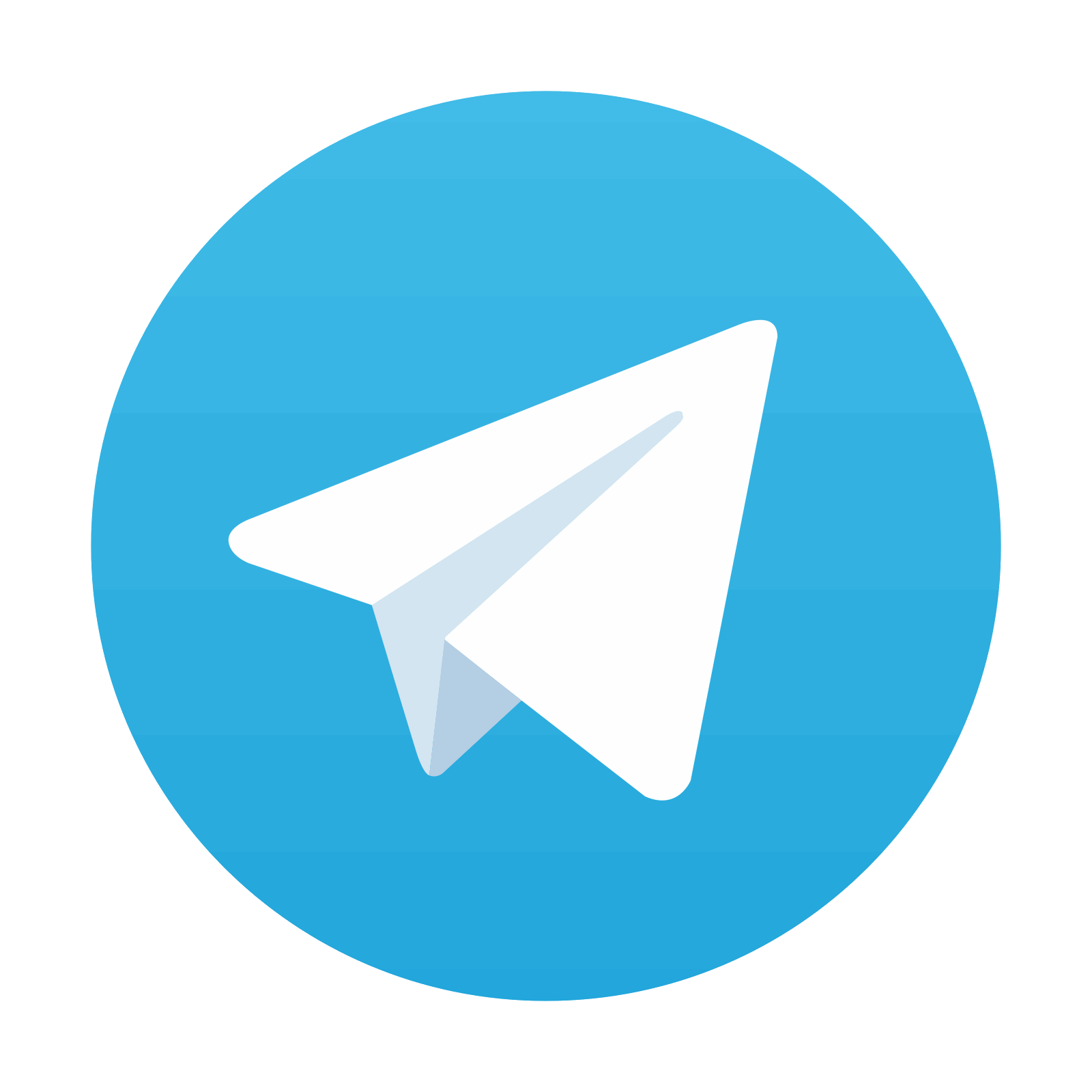
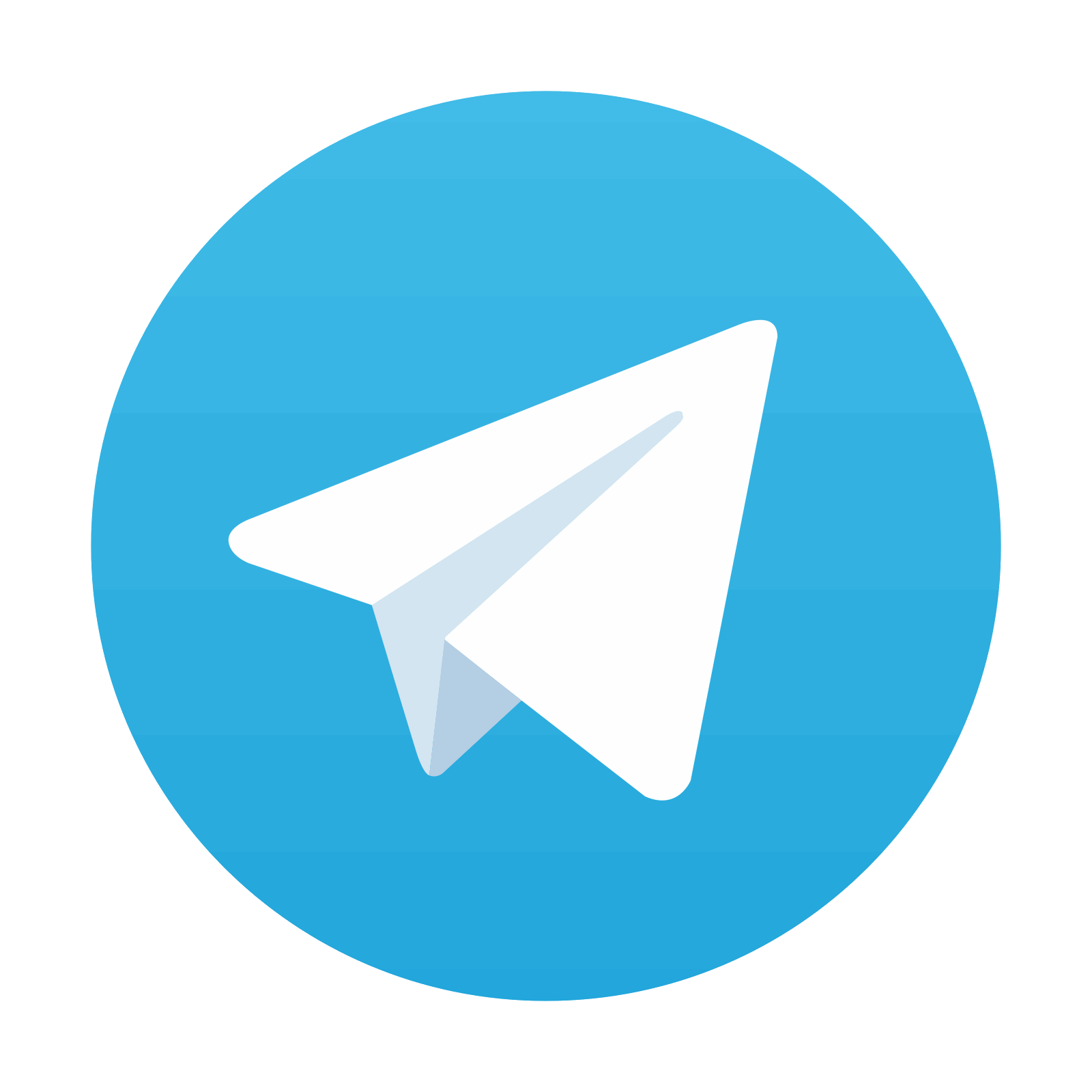
Stay updated, free articles. Join our Telegram channel

Full access? Get Clinical Tree
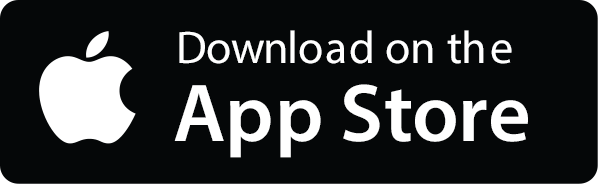
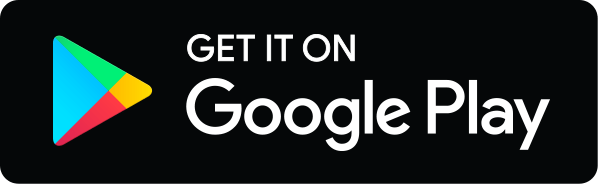