© Springer International Publishing Switzerland 2017
Anthony A. Gaspari, Stephen K. Tyring and Daniel H. Kaplan (eds.)Clinical and Basic Immunodermatology10.1007/978-3-319-29785-9_5151. Immunobiology and Immune Based Therapies of Melanoma
(1)
Department of Dermatology, University of Colorado Denver, Anschutz Medical Campus, 1665 Aurora Ct., MS F703, Aurora, CO 80045, USA
(2)
Department of Dermatology, University of Colorado School of Medicine, Aurora, CO 80045, USA
Abstract
Despite efforts in preventative care, the incidence and mortality rate of melanoma have continued to rise. However, in the past decade, an increasing understanding of melanoma immunology has resulted in tremendous bench to bedside advancements in melanoma therapies. Many of these new treatment modalities have proven efficacious, leading to seven new melanoma therapies receiving FDA approval within the past 5 years. This chapter explores our current understanding of melanoma immunology, with an emphasis on tumor microenvironment and the ability of melanoma to evade immune surveillance. The remainder of the chapter focuses on how this understanding of melanoma immunology has been translated into clinical therapies. These agents include cytokines such as interleukin-2 and interferons, checkpoint inhibitors such as anti-CTLA4 and anti-PD1 antibodies, and vaccines. For each melanoma therapy, mechanism of action, efficacy, side effects, dosing strategy and future directions will be discussed.
Keywords
CancerMelanomaSkin diseaseImmune based therapiesT-lymphocytesDendritic CellsAdhesion MoleculesChemotherapyGangliosidesBiochemotherapyWhile melanoma accounts for only a small percentage of all cutaneous malignancies, it remains an important health issue due to its high mortality rate. In 2016, there were 76,380 new cases of malignant melanoma diagnosed, and 10,130 deaths attributed to melanoma [1]. These statistics are particularly concerning, as they represent a greater than 25 % increase from data only 8 years ago [2]. Cutaneous malignant melanoma originates from melanocytes, neural crest derived pigment cells residing in the basal layer of the epidermis. Our current understanding of melanoma development involves a step-wise progression through stages of growth, including cellular atypia, radial growth, vertical growth, and the development of metastases [3]. This model corresponds to the American Joint Commission on Cancer (AJCC) staging classification of melanoma that defines primary cutaneous melanoma as Stage I or Stage II disease, lymph node metastases as Stage III disease, and distant metastases as Stage IV disease [4–6]. This transition of melanocytes into neoplastic melanoma cells that initially grow in the skin and later metastasize to distant sites involves complex host-tumor cell interactions. Our understanding of host-tumor cell interactions has progressed rapidly in recent decades, and translating these basic science developments into clinical treatment of melanoma has yielded promising results. Insights into the host immune response to malignant melanoma have led to the development of new approaches to melanoma therapy.
Melanoma Immunology
It is widely accepted that melanoma cells and the immune system are intricately connected. For instance, systemic immune suppression from human immunodeficiency virus infection is associated with an increased rate and more aggressive behavior of malignant melanoma [7–9]. Melanoma cells are capable of triggering host immunologic responses—depigmentation and regression of primary melanomas provide supporting evidence that host immune surveillance can occur [10, 11]. Finally, different types of immune cells have been noted to infiltrate tumor sites in malignant melanoma, and an increased number of tumor infiltrating immune cells has been associated with an improved prognosis, even in more advanced disease [12]. Indeed, different components of the immune system are activated in response to melanoma cells, and basic investigations of these immune responses are critical to the development of immune-based therapeutic approaches to melanoma.
T-Lymphocytes and Dendritic Cells
Numerous studies have shown that T-lymphocytes play a major role in melanoma immunology and immunotherapy. T-lymphocytes have been observed to accumulate in melanoma lesions and lyse autologous tumor cells in vitro, as well as to cause tumor regression when transferred in vivo in 30 % of melanoma patients [13]. Cytotoxic T-lymphocytes (principally CD8+) cells respond primarily to target cells with specific antigens associated with class I major histocompatibility complex (MHC) proteins. Activation of cytotoxic T-lymphocytes results in destruction of target cells that have altered MHC I antigen expression. Helper T-lymphocytes (CD4+ cells) respond primarily to class II MHC proteins and the associated antigens on the antigen presenting cells (APCs). Activated CD8+ cytotoxic T-lymphocytes and CD4+ helper T-lymphocytes produce a variety of cytokines that function to amplify and modify the immune response to the growing tumor [14, 15]. Melanoma cells, however, may thrive and proliferate by evading host immune surveillance and defense, as demonstrated by the fact that brisk immune activation in clinical trials does not always result in successful clinical response [16]. This evasion occurs by a number of mechanisms, including antigen loss, downregulation of MHC expression, and reduction of the T-helper 1 (Th1) arm of the immune response [17]. In addition, a subpopulation of human regulatory T-lymphocytes (Tregs), characterized as CD4+CD25+ T-lymphocytes, typically has the function of suppressing self-reactive T-lymphocytes [18]. However, Tregs may suppress anti-tumor immune responses, as seen in animal models of melanoma [19]. Patients with metastatic melanoma have increased numbers of these CD4+CD25+ T-lymphocytes that may influence responses to various immune modulating therapies [20]. More recently, T-lymphocytes were demonstrated to express cytotoxic T-lymphocyte antigen 4 (CTLA-4), which when bound to the B7 target on APCs, can lead to T-lymphocyte anergy, a T-lymphocyte poor microenvironment, and immunologic ignorance of tumor, in melanoma and other solid tumors [21]. Even in a T-lymphocyte rich environment, melanoma evasion may occur due to T-lymphocyte exhaustion and dysfunction due to the expression of programmed death-1 (PD-1) receptor on T-lymphocytes and the binding of its ligands, PD ligand (PD-L) 1 and PD-L2 that are expressed on tumor cell surface [21–23].
The role of B cells and humoral immunologic responses to melanoma cells remains undefined. Natural killer cells also appear to play a role in melanoma immune surveillance and the destruction of neoplastic cells with reduced MHC expression [24, 25].
Dendritic cells are active participants in the host immune response to melanoma. Dendritic cells are bone marrow derived leukocytes, which are potent APCs and play a critical role in initiation of T-lymphocyte immune responses [26]. In the skin, APCs are also known as Langerhans cells. Dendritic cells express high levels of both MHC class I and class II molecules, as well as T-lymphocyte costimulatory molecules [i.e., CD40, CD80 (B7-1), CD86 (B7-2)] [27]. Dendritic cells are capable of secreting a variety of cytokines, such as Interleukin (IL)-1 and IL-12, which serve as accessory factors for T-lymphocyte activation. Activated dendritic cells are mobile, monocyte-derived cells that traffic to lymphoid organs to present foreign or tumor cell antigens to specific populations of T-lymphocytes, which results in the activation of antigen specific effector T-lymphocytes [28]. It has been documented that dendritic cells themselves can infiltrate melanomas, although the purpose of this infiltration still needs to be defined [29]. Previous studies have suggested that melanoma-derived factors may convert dendritic cells from potent immune response inducers into tolerance-inducing APCs [26], which may limit the development of dendritic cell melanoma immunotherapies.
Adhesion Molecules
A number of distinct cellular adhesion molecules and cytokines have been implicated in the local growth and metastatic progression of melanoma cells. Tumor progression and the development of metastatic disease are often associated with loss of normal cellular controls. The alteration of tumor cellular adhesion molecule expression and function allows neoplastic cells to separate from the primary tumor mass to establish metastatic lesions. With separation of the metastatic cells from the primary tumor mass, neoplastic cells are freed from local cell contact inhibitory mechanisms. Altered expression of adhesion molecules in neoplastic cells can also improve their ability to adhere to vascular endothelium facilitating metastases to distant sites. Many different tumor-associated cell adhesion molecules have been implicated in the progression of melanoma from radial growth, to vertical growth and then to metastases. These key adhesion molecules include integrins, cadherins and immunoglobulins such as ICAM-1 [30]. In vitro and in vivo studies have shown that melanoma cells alter their cell surface adhesion molecules as they progress through the different stages of growth, and that expression of certain cell surface molecules by melanoma can yield prognostic information [30, 31].
One example of a cell adhesion molecule associated with melanoma pathogenesis is MUC18/MCAM/CD146, a cell surface glycoprotein that mediates adhesion to different cell types. MUC18 is expressed on both vascular endothelia and activated T-lymphocytes, facilitating the extravasation and/or homing of activated T-lymphocytes [32, 33]. In contrast, the expression of MUC18 on melanoma cells is associated with tumor progression and increased metastatic potential in both humans and mice [34, 35]. The level of expression of MUC18 on primary melanoma tumors increases as the tumors increase in thickness and develop metastatic potential [34, 36]. The ability of human melanoma cell lines to form metastases in mice has been correlated with the level of expression of MUC18 [37]. In light of these findings, a human monoclonal antibody against MUC18, ABX-MA1, has demonstrated therapeutic promise in mouse models of melanoma [38].
Cytokines
Melanoma cells are capable of producing a variety of cytokines, which not only modulate the growth of tumor cells, but also act to promote or inhibit the host antitumor inflammatory and immune response [39]. These melanoma-derived cytokines have different biologic activities and can act as autocrine growth factors, growth inhibitors, growth promoters, or immunosuppressive factors. Some of these cytokines allow the melanoma cells to grow independently of exogenous growth factors.
Basic fibroblast growth factor (bFGF), IL-6, and chemokines such as IL-8 are capable of acting as melanoma autocrine growth factors. bFGF is expressed by melanoma cells, but not by melanocytes, and appears to provide a growth advantage to tumor cells [40, 41]. Some melanoma cell lines produce IL-6, which is a major immune and inflammatory mediator that appears to have conflicting roles in regulating melanoma growth and progression [42–44]. Previous studies indicate that IL-6 may be both a growth inhibitor and an autocrine growth stimulant for melanoma cells depending on the stage of tumor progression [45]. In vitro studies also demonstrate the ability of IL-8 to function as an autocrine growth factor for melanoma cells [46]. A positive correlation between melanoma IL-8 production and tumor thickness in primary cutaneous melanoma has been reported [47]. Additionally, IL-8 production has been shown to correlate with melanoma metastatic potential in nude mice [48] and with the induction of migration by melanoma cells [49].
Tumor derived transforming growth factor-β (TGF-β) and colony stimulating factors (CSFs) may also modulate the growth and progression of melanoma. The TGF-βs are potent regulators of the immune response, cellular proliferation, differentiation, and extracellular matrix synthesis and deposition [50]. The proliferation of most melanoma cell lines is inhibited by TGF-β [40, 51]. However, some melanoma cell lines established from metastatic tumors have been shown to become resistant to the growth inhibitory effects of TGF-β. This cytokine may even act as a growth stimulator in some of these advanced melanoma cell lines [52]. Since TGF-β has potent immunosuppressive activities, the production of this cytokine by melanoma cells may inhibit the host immune responses to the tumor. Some melanoma cells are capable of producing a number of CSFs including granulocyte-colony stimulating factor (G-CSF), granulocyte-macrophage-colony stimulating factor (GM-CSF), stem cell factor/mast cell growth factor, and monocyte-colony stimulating factor [43, 53]. Host anti-melanoma effects have been most clearly demonstrated with GM-CSF. Studies have shown that GM-CSF produced by melanoma cells can induce monocyte Tumor Necrosis Factor α (TNF-α) production, which may contribute to host anti-tumor responses [54]. GM-CSF gene transfection into melanoma cells results in significant inhibition of melanoma progression by activating host immune responses [55, 56]. In human pathological specimens, melanoma tumor thickness inversely correlates with GM-CSF melanoma cell production, suggesting that GM-CSF may have an anti-melanoma role in early primary lesions [47].
IL-10, which typically has a number of immunosuppressive properties, is another cytokine produced by some melanoma cells. Studies have also demonstrated that, in some circumstances, IL-10 appears to act as a growth factor for melanoma cells in vitro and can downregulate the expression of HLA-I, HLA-II, as well as ICAM-I [57]. Melanomagenesis is known to be involved in the IL-10 cytokine pathway. Ultraviolet (UV) radiation can decrease epidermal Langerhans (dendritic) cells and stimulate production, by both T-lymphocytes and a small population of melanoma cells, of immunosuppressive and tumor growth promoting IL-10. The result is a milieu that allows the initial expansion of melanoma cells [58, 59]. Patients with advanced metastatic melanoma have also been found to have elevated serum IL-10 levels, which is rarely observed in healthy patients [60]. Thus, IL-10 predominantly has immunosuppressive and tumor promoting activities in the setting of melanoma [61].
Melanoma Associated Antigens
A number of antigens located on the cell surface of melanomas have been referred to as melanoma associated antigens (MAA). These antigens can be divided into two major categories: HLA-associated antigens that are recognized by T-lymphocytes and cell surface antigens such as gangliosides that do not directly activate T-lymphocytes [17, 62]. Unlike gangliosides, melanoma associated antigens recognized by T-lymphocytes are derived from proteins that do not normally reside on the surface of normal cells, and thus can evoke an immune response in association with particular MHC molecules on the cell surface. These peptide-based antigens can be grouped into three different categories: antigens expressed only on cells of melanocytic lineage (i.e. tyrosinase, TRP-1, TRP-2, Melan-A/MART1, and gp100), antigens expressed in different kinds of tumor cells but not in normal adult tissues outside of the gonads (i.e. MAGE, BAGE, GAGE, NY-ESO-1 and PRAME), and unique antigens found only on some tumor cells [17]. Interest in MAAs focuses around potential immunotherapies for melanoma, particularly vaccine therapy.
Cytotoxic T-lymphocyte clones that have been identified and expanded from melanoma patients may recognize specific antigens expressed on both melanoma cells and normal melanocytes [63]. Melanoma associated cytotoxic T-lymphocyte antigens include tyrosinase, tyrosinase-related protein 2, tyrosinase-related protein 1/gp75, silver/gp100 protein, Melan-A/MART1, and the Lerk proteins (lerk-1/protein B61 and Lerk-5/Eplg5/Elf2/HTK-L). Tyrosinase is an enzyme that converts tyrosine into dihydroxyphenylalanine (DOPA), the precursor of melanin. The gene that codes for tyrosinase is found in all melanoma cells as well as normal melanocytes, but is not expressed in other cell types. The silver/gp100 protein (also referred to as Pmel [64]) is a melanosomal matrix protein that acts as a solid-phase substrate for the accumulation of melanin [65]. The gp100 peptide is a melanocyte differentiation antigen that is recognized by HLA-A2 restricted T-lymphocytes [66]. Another gene that is expressed only in cells of melanocytic origin is Melan-A [67]. The antigen encoded by Melan-A is called MART-1. MART-1 is only found on normal melanocytes, melanoma cells and retinal cells [68]. MART-1 is presented by HLA-A2 and is capable of causing a strong T-lymphocyte immune response [69].
Antigens that are expressed almost exclusively on tumor cells and extensively on melanoma cells include products of the MAGE gene family. MAGE genes are all located on the long arm of the X chromosome and are only found on the testis in normal adult tissue. The MAGE proteins are commonly expressed on melanoma cells and less frequently on the surface of other tumor cells. The genes MAGE-1, 2, 3, 4, 6, and 12 are expressed in different tumors such as breast carcinomas, non-small cell lung carcinomas, head and neck tumors, sarcomas, and ovarian carcinomas [70–73]. The products of MAGE-1 and MAGE-3 have been found to produce proteins that bind the different MHC class I molecules expressed on melanoma tumor cells. Products of MAGE-1 have been reported to be expressed on 48 % of metastatic melanomas, while products of MAGE-3 are expressed in >90 % of metastatic melanomas [74, 75]. Even though some proteins produced from MAGE genes have been characterized, their function on the surface of tumor cells is unknown.
Melanoma Gangliosides
Melanoma cells also express a number of gangliosides on their cell surfaces. Gangliosides are glycolipids that are characteristically found in neural crest-derived tissues [14]. Gangliosides regulate cell growth by altering growth factor signals and are involved in cell adhesion and cell matrix interactions [76]. The monosialoganglioside GM3 makes up 90 % of the gangliosides found on normal melanocyte cell surfaces, while the disialoganglioside GD3 comprises only 5 % [76]. It has been shown that quantitative and qualitative changes occur in the expression of gangliosides during oncogenic transformation [77]. The predominant gangliosides in melanoma are GM3 and GD3. GM3 is found on virtually all melanoma cells. GD3 is found on few normal melanocytes and at lower levels than on melanoma cells. The minor gangliosides are expressed on most melanoma cells but less often in normal tissues. These minor gangliosides include GM2, GD2, GT3, and 9-O-Ac-GD3 [78]. As a result, melanoma gangliosides are a possible target for immunotherapy against melanoma, and work has focused on several of these molecules in vaccine therapy.
Treatment Strategies for Melanoma
When detected early, melanoma responds well to treatment. However, it becomes a difficult cancer to treat once it extends beyond the skin. In 2014, more than 9700 people are estimated to have died from malignant melanoma in the US, and the incidence of the disease continues to increase yearly [2, 79]. Surgical excision of thin primary cutaneous melanoma that is detected early in melanomagenesis remains the most effective therapy. The 5 year and 10 year survival rate is 97 % and 93 %, respectively, for fully excised cutaneous melanoma that is 1 mm or less in thickness, without ulceration, having less than 1 mitotic figure per millimeter squared, and without nodal involvement or distant metastases [4, 6]. In contrast, patients with melanoma that has metastasized to the viscera have a drastically reduced survival: the 1 year survival rate is 33 % and the 5 year survival is only 10–15 % [4, 6]. Novel therapies for melanoma have begun to improve the poor prognosis associated with thicker, more invasive melanomas, as well as metastatic disease. Recent advances in our understanding of the immunobiology of malignant melanoma offer the potential for new and more effective approaches for disease management. As immunological treatments are being combined with non-immunological therapy clinically, we begin with a brief discussion of non-immunological treatments.
Non-immunological Treatments
Surgical Treatments
As noted above, melanoma has an excellent prognosis when detected early and treated with surgical intervention. The depth of the tumor determines the local surgical margins, and for most thin tumors surgery is considered curative [80]. Tumor depth is reported as Breslow depth, the measurement in millimeters of the tumor invasion from the granular layer of the epidermis. However, the presence of local lymph node metastases (Stage III disease) is a strong predictor of poor clinical outcomes. Lymph node involvement in patients with melanoma has a 5-year survival rate of 46 %, compared to greater than 90 % for patients without lymph node involvement. The technique of sentinel lymph node biopsy (SLNB) has been developed as a way to identify patients with clinically occult nodal involvement, and is a far less morbid surgical intervention than empiric complete lymph node dissection (CLND) [6, 81–86]. The SLNB technique involves the injection of a vital blue dye or radiolabeled agent into the area of the primary tumor. This labeling allows for identification and subsequent sampling of the sentinel node (or nodes) during or prior to wide local excision [87]. Studies have shown that if the sentinel node is negative, there is less than a 1 % chance of positive nodes elsewhere in the lymph node basin [87]. This technique has been primarily studied in melanomas that are at high risk for metastatic disease but still amenable to early intervention – melanomas of intermediate depth of 1–4 mm [81, 85, 88]. Based heavily on the data from the Multicenter Selective Lymphadenectomy Trial-1 (MSLT-1), the clinical practice guidelines from the American Society of Clinical Oncology and the Society of Surgical Oncology recommend that SLNB should be performed in patients with melanoma of intermediate depth, followed by CLND if the SLNB is positive. This trial has demonstrated that SLNB improves relapse free survival and regional disease control in melanoma, as well as providing prognostic and staging information for patient management [83, 85, 88–90]. However, in spite of significant optimism for this technique in the treatment of melanoma, clinical trials have not shown an overall survival benefit from SLNB, and, as a result, the technique remains a subject of much debate [91].
Traditional Chemotherapy
Dacarbazine (DTIC) is the only traditional chemotherapeutic agent approved for the treatment of advanced melanoma by the Food and Drug Administration (FDA). Clinical trials demonstrate that approximately 10–20 % of patients with metastatic melanoma have a measurable clinical response to the drug, but fewer than 5 % achieve complete responses [92]. Innumerable combinations of different chemotherapeutics have been tried in the treatment of metastatic melanoma [93]. In spite of this effort, a recent meta-analysis failed to show a survival benefit in several different multi-drug chemotherapy regimens when compared to DTIC alone [93]. There has also been interest in the drug temozolomide, an oral formulation of DTIC, in the management of melanoma. Temozolamide has not been shown to have improved efficacy over DTIC, but has been used for palliative treatment of melanoma with brain metastases, due to its improved ability to penetrate the central nervous system [94–97]. While traditional chemotherapy agents have not been shown to improve overall survival, they remain useful in select clinical cases as palliative options [96, 97].
Small Molecule Inhibitors
The mitogen-activated protein kinase (MAP-Kinase) pathway is a cellular signal transduction pathway that regulates cell division and differentiation. The majority of melanomas carry an activating mutation in the upstream serine-threonine protein kinase BRAF in this pathway, leading to uncontrolled cell growth and eventually tumor development. Genetic studies have shown that up to 90 % of the BRAF mutations in melanoma result from a substitution of glutamic acid for valine at amino acid position 600 (V600E mutation) [98]. This discovery has led to the development of small molecule inhibitors against the V600E target. The first of these agents, vemurafenib, demonstrated significant improvement in overall survival in patients with metastatic melanoma harboring the mutation as compared to DTIC in Phase III trials, leading to FDA approval for treatment of V600-mutated melanoma in 2011 [99–101]. Dabrafenib, another V600E small molecule inhibitor, was approved by the FDA for similar indications after demonstrating success in its Phase III clinical trial [102]. Vemurafenib is given orally at a dose of 960 mg twice per day, while dabrafenib is given orally at a dose of 150 mg twice per day.
V600E-mutated melanomas eventually become resistant to BRAF inhibition, due to the development of bypass neuroblastoma RAS oncogene homolog (NRAS) pathway or downstream mitogen activated protein kinase kinase (MEK) mutation [103]. The discovery of this resistance prompted the development of trametinib, a small molecule agent that blocks downstream MEK targets. Trametinib is now approved by the FDA for salvage monotherapy and for use in combination with BRAF inhibitors in the treatment of metastatic melanomas harboring the V600E mutation [104, 105]. Trametinib is prescribed as 2 mg by mouth daily. Cobimetinib is another MEK inhibitor that has been shown to increase overall and progression-free survival when used in conjunction with BRAF inhibitors [106, 107].
C-KIT is a receptor tyrosine kinase that can activate downstream MAP-kinase pathway. Small molecule inhibitors of C-KIT, such as imatinib and nilotinib, were developed for the treatment of malignancies with c-kit mutations, most notably chronic myelogenous leukemia [108]. Recent discoveries of c-kit mutations, found in mucosal and acral melanomas, and melanomas arising from chronically sun-damaged skin, have led to studies of imatinib and nilotinib in melanoma therapy, although these remain in clinical trials at the present [109–111]. An overexpression of vascular endothelial growth factor (VEGF) has been found in some melanomas, and humanized monoclonal immunoglobulin bevacizumab, which targets VEGF-ligand, is also in clinical trials in patients with advanced melanoma [112, 113].
Immunological Therapies
Overview
Immunotherapeutic approaches to melanoma can be classified as those designed as adjuvant therapy after treatment of the primary lesion to prevent subsequent metastatic disease and those designed as immunotherapy for clinically apparent metastatic tumors. Adjuvant therapy is administered to patients at high risk for the development of metastatic disease, but who have no detectable disease at the time of surgical resection of the primary melanoma or after surgical resection of limited metastatic lesions such as to regional lymph nodes. High-risk melanoma patients include those with thick primary lesions in the skin, those with ulcerated melanomas, or those in which tumor cells are detected in regional lymph nodes. Current melanoma adjuvant treatment options are limited and, for the most part, ineffective.
Adjuvant therapeutic approaches for high-risk cutaneous melanoma have included clinical trials with interferons, specific and nonspecific immunotherapy, adjuvant chemotherapy and biochemotherapy, isolated limb perfusion, adjuvant radiation therapy, adjuvant hormonal therapy, and adjuvant retinoid therapy [114–116]. The development of active specific immunotherapy has resulted in melanoma vaccines employing purified gangliosides, shed tumor antigens, specific isolated tumor peptides, mechanical or viral melanoma cell lysates, antigen-primed dendritic cells, and allogeneic or autologous whole tumor cell preparations [117, 118]. More recently, vaccines are being made that target tumor-specific missense mutations, and these have been shown to increase anti-tumor immunity on a personalized level, bringing about the possibility for individualized melanoma immunotherapy that targets private tumor mutations [119].
Research into immune-based management of melanoma has focused not only on adjuvant therapy, but also in the treatment of advanced metastatic melanoma [80, 120]. These patients are a significant therapeutic challenge from an immunologic standpoint, because they are often immunosuppressed and debilitated. Nevertheless, a number of clinical immunotherapeutic trials for metastatic melanoma are currently in progress for patients with Stage IV disease, with some reaching FDA approval. The role of various cytokines as immunotherapy for melanoma treatment remains an active area of investigation [121], as well as the use of tumor vaccines and techniques such as adoptive immunotherapy [49, 118]. Antibodies that inhibit T-lymphocyte surface receptors, which when activated have a downregulatory effect, have also shown promise in melanoma therapy [122]. Whereas prior immunotherapies have shown little survival advantages over surgical therapy alone, novel new immunotherapy agents have shown great promise in demonstrating a survival benefit with an acceptable tolerability profile.
Immunotherapeutic Agents
Cytokines
The role of cytokines in immunotherapeutic approaches to cancer treatment remains an active area of investigation. Most of the data dealing with the role of melanoma-derived cytokines in the immunology of melanoma has been obtained by transfecting immunomodulating cytokine genes into neoplastic cells and then observing the biologic response in murine model systems. This approach has been useful to evaluate the in vivo consequences of tumor cell cytokine production in an animal host in a number of different tumor systems [123–127]. The in vivo consequences of tumor cell cytokine production have been evaluated for a number of cytokines including IL-2, IL-4, IL-18, IL-12, G-CSF, GM-CSF, M-CSF, IFNγ, IL-6, and IL-10 [55, 56, 123–131]. Although IL-2 and IFN-α are the only FDA approved cytokines for the treatment of melanoma, several other molecules in this family have also entered early clinical trials. IL-12 has shown promise in early studies, although systemic administration resulted in significant toxicities, including arthralgias, neutropenia, arrhythmias, and hypotension [132]. However, new methods of targeted delivery, including electroporation and transfection of IL-12 plasmids directly into tumor, or the use of a fusion protein of IL-12 and an antibody that recognizes tumor-specific antigens, hold promise in minimizing side effects by limiting the action of IL-12 to the area immediately surrounding the tumor [133–135]. Recombinant human IL-18 had gone through Phase I and II testing and demonstrated only limited efficacy, although there remains interest in its use as an adjuvant agent [136, 137].
Interleukin-2
Currently, one of the few FDA approved treatments for metastatic melanoma is high dose systemic IL-2. High dose systemic intravenous IL-2 has been shown to induce a clinical response in 16 % of patients with metastatic melanoma, with 10 % of patients achieving partial response (reduction of tumor) and 6 % achieving complete response (resolution of tumor) [138]. While response rates to systemic IL-2 are low, recent studies demonstrate that the treatment appears capable of inducing durable responses in a small percentage of patients [139]. Follow-up data at 5 years indicates that, of the patients with metastatic melanoma who responded to IL-2 therapy longer than 30 months, none have had disease progression [121]. In order to determine which patients might benefit most from the treatment, attempts have been made to identify clinical, genetic or immune characteristics of melanoma patients who have a vigorous response to IL-2 [140, 141]. For instance, the majority of patients with metastatic melanoma receiving standard high dose IL-2 had an increase in immunosuppressive CD4+CD25+ Tregs, but those patients who achieved an objective clinical response had a significant decrease in Tregs [20, 142, 143]. Targeting patients who would be most likely respond to IL-2 would be beneficial, as IL-2 does not have a favorable toxicity and side effect profile. Most significantly, treatment results in severe vasodilation and capillary leak, leading to a septic shock profile with hypotension in 64 % of patients. These toxicities limit both the patients who can receive the medication and the medical centers eligible to administer this therapy [139]. Of note, intralesional IL-2 injection has been used with success and with decreased side effect profiles in case series to induce regression of in-transit metastases [144]. High dose systemic IL-2 is dosed as 600,000 international units (IU)/kg, given intravenously over 15 min every 8 h for a maximum of 14 doses. This is followed by 9 days of rest, with a maximum of 14 more doses. The patient is retreated if tumor shrinkage is observed.
Interferon
The FDA approved high dose interferon α-2b as an adjuvant treatment for thick melanoma lesions or positive nodal disease in 1995. The FDA approval stemmed from a Phase III trial demonstrating an improvement in disease free survival from 1.1 to 1.7 years and overall survival from 2.8 to 3.8 years with adjuvant interferon treatment after surgical excision [145]. However, subsequent trials have not supported these results and a recent meta-analysis concluded that interferon is associated with an increase in disease free survival, but not an improvement in overall survival [146, 147]. Additionally, attempts to modify the dosing of interferon in order to decrease toxicity and improve efficacy have not been successful. Trials with both intermediate and low dose levels of interferon-α have failed to show an improvement in survival [148–150]. In addition, side effects of interferon-α, including fever, chills, myelosuppression, neurologic deficit, and hepatotoxicity, are poorly tolerated, and affect a significant percentage of patients, with less than 40 % of patients able to complete at least 80 % of a full course of therapy [145]. As a result, the role of interferon-α as an adjuvant treatment in melanoma remains limited and controversial. Interferon-β had been shown to have inhibitory effect on melanoma cells in the Japanese population. However, genetic therapy with interferon-β has not shown significant efficacy in the treatment of metastatic melanoma in the United States [151, 152]. Today, efforts on combining interferon therapy with other immune-based therapies, as will be described later on, are underway [153]. The current standard dosing for interferon-α is an induction phase of 20 million IU/m2 body surface area, through IV infusion for 5 consecutive days per week for 4 weeks, followed by a maintenance phase of 10 million IU/m2 body surface area, through subcutaneous injection 3 times per week for 48 weeks.
GM-CSF
Granulocyte-macrophage colony-stimulating factor (GM-CSF) is a growth factor that functions as a potent inhibitor of the growth and progression of melanoma [55, 56]. This effect is mediated by the host immunological system and appears to involve activation of dendritic cells [154]. The anti-melanoma effects of GM-CSF have been evaluated in patients with high-risk Stage III and Stage IV disease, demonstrating an increase in disease-free and overall survival in a Phase II study of 48 patients [155]. Other studies have looked at GM-CSF as an adjuvant immunotherapy [156] and in perilesional injection of melanoma sites [157, 158]. Most current work focuses on the use of GM-CSF as an adjuvant therapy to increase immune response in vaccine trials [159].
Imiquimod
Imiquimod is an imidazoquinoline that is prepared in a topical formulation. Imiquimod binds to toll-like receptor 7 (TLR-7) on the surfaces of antigen presenting cells, inducing a signaling cascade that activates nuclear factor-kB (NF-kB). This activation leads to an upregulation of Th1 response cytokines, including TNF-α, IFN-α, IL-12, and IL-18, which can then activate CD8 cells to become cytotoxic T-lymphocytes [160]. Imiquimod is FDA approved for use in the treatment of actinic keratosis, genital warts, and superficial basal cell carcinoma. Experimentally, imiquimod was found to be capable of inducing apoptosis in melanoma in initial in vitro and in vivo studies [161]. Subsequently, the off-label use of topical imiquimod for the treatment of melanoma, primarily the lentigo maligna variant of melanoma in situ, has been pursued. To date, the data on imiquimod’s success has remained largely anecdotal case series, and studies have been hindered by insufficient patient follow-up time [162–165]. To date, no randomized controlled trials exist that compares imiquimod use versus surgical intervention, traditionally considered the gold standard for treatment of melanoma in situ [166]. Nevertheless, imiquimod remains an off-label option in patients with lentigo maligna who are not candidates for surgical therapy. Though case reports exist on the use of imiquimod for the treatment of metastatic melanoma, imiquimod is not recommended as therapy for invasive primary melanoma or metastatic melanoma [161, 166–169].
Biochemotherapy
Researchers have combined immunotherapy with other treatment modalities in an attempt to increase response rates. Several groups have administered IL-2 and IFN with traditional chemotherapy (i.e. dacarbazine or tamoxifen) for ‘biochemotherapy.’ Although some initial results were promising, larger trials have failed to show that the combinations selected result in longer survival times [170–173]. Pooled trial data has indicated increased response rates with biochemotherapy, but this comes at the cost of decreased quality of life and increased treatment related toxicity for patients [173].
Cytotoxic T-Lymphocyte Antigen 4 Blockade
Cytotoxic T-lymphocyte antigen 4 (CTLA-4) is a surface glycoprotein that functions as an inhibitor to T-lymphocyte activation, working in opposition to CD28 [174]. Specifically, T-lymphocyte activation requires a costimulatory signal of the CD28 molecules on their surfaces binding to B7/CD80 on APCs. However, in locations such as the microenvironment surrounding melanoma, T-lymphocytes can express CTLA-4 that binds B7/CD80 instead, inducing T-lymphocyte anergy by preventing the CD28-B7 costimulatory signal. This T-lymphocyte anergy then allows melanoma cells to evade the immune system [21, 175].
Initial studies in murine models demonstrated that antibody inhibition of CTLA-4 could enhance the destruction of certain tumors, particularly those that were more immunogenic [176]. In vitro studies in humans also evaluated if inhibition of CTLA-4 could enhance the immune cell response against ‘self’ antigens, such as gp100 and Mart-1, which are expressed by melanoma cells [177–179]. Overall response rates, defined as tumor shrinkage of at least 30 %, in early trials were found to be around 15 % after treatment with an antibody to CTLA-4 administered alone or with other agents, such as vaccines against tumor antigens, and, as expected, response rates appear to correlate with the development of auto-immunity [180, 181]. In human trials, ipilimumab, a recombinant human monoclonal antibody against CTLA-4, demonstrated a median overall survival benefit of 4 months by itself or in combination with gp100 peptide vaccine, compared to the active control arm that used gp100 peptide vaccine alone [179, 182, 183]. Compared to dacarbazine monotherapy, ipilimumab with dacarbazine increased overall survival [184]. These promising data led to FDA approval of the medication in 2011 for metastatic melanoma treatment. Long-term studies of ipilimumab have also demonstrated a surprisingly durable response with this treatment, with a 3-year survival of 22 % in a multi-study pooled analysis, and a 5-year survival of 18 % in a smaller single trial analysis. Of note, the survival analyses was done irrespective of tumor shrinkage [185, 186]. However, the autoimmune side effects induced by CTLA-4 blockade can be severe, ranging from uveitis, gastroenteritis, colitis, dermatitis, and hypophysitis. Over half of patients treated with ipilimumab developed autoimmune side effects, with 20 % discontinuing treatment due to drug-related adverse events, bringing into question the tolerability of CTLA-4 blockade for a significant number of melanoma patients [182, 187]. Furthermore, ipilimumab may have a very slow onset of action. Prieto et al. demonstrated that in clinical trials of ipilimumab, patients who achieved clinical remission did so on average 30 months after the start of their therapy [188, 189]. Ipilimumab is given as an intravenous injection at 3 mg/kg every 3 weeks for a total of 4 doses. In a recent Phase III trial evaluating the use of adjuvant ipilimumab following resection of Stage III melanoma, a dose of 10 mg/kg was used, and while recurrence-free survival was improved, 52 % of patients had to discontinue treatment due to drug-related adverse events and 5 of 475 patients in the treatment arm died from therapy related adverse events [190].
Tremelimumab was another anti-CTLA-4 antibody evaluated as possible immune modifying therapy for melanoma. In clinical trials, while the treatment was tolerable to patients and the durability of treatment response was longer than traditional chemotherapy (i.e. DTIC), tremelimumab offered no survival advantage over DTIC [191, 192].
Programmed Cell Death Receptor (PD) 1 and PD-Ligand (PD-L) 1 Blockade
The PD-1 receptor is a T-lymphocyte surface receptor that binds two ligands, PD-L1 and PD-L2, that are found on the surfaces of tumor cells, including melanoma, renal cell carcinoma, colorectal cancer, and non-small cell lung cancer [193, 194]. PD-1 and PD-L1 binding in the tumor microenvironment leads to T-lymphocyte exhaustion and inhibition, allowing for tumor evasion of the immune system [195–197]. Nivolumab, a humanized monoclonal antibody targeting the PD-1 receptor to prevent PD-L1 binding, was developed to prevent T-lymphocyte inhibition, thus allowing for continued immune response to tumor. In the initial Phase I trial of nivolumab, 28 % of patients with advanced melanoma enrolled in the study attained tumor regression, with the majority having a durable response to therapy of more than 1 year [198]. In a follow-up study of advanced melanoma patients treated with nivolumab, median overall survival was noted to be 16.8 months, with 1 year and 2 year survival rates of 62 % and 43 %, respectively, in all patients enrolled [198, 199]. Maintenance of response off-therapy was found to be at least 16 weeks in patients who had discontinued treatment [200]. Impressively, nivolumab was noted to be efficacious in patients who had previously failed ipilimumab and vemurafenib [199, 201–203]. Adverse events associatedwith nivolumab therapy in melanoma patients include fatigue, rash, and diarrhea, and rarely thrombocytopenia [200]. Of all patients treated with nivolumab, 1 % died of drug-induced pneumonitis, although none were patients with melanoma [200]. Nonetheless, nivolumab appears extremely well tolerated in patients with melanoma, with only 7 % of patients requiring discontinuation of treatment due to treatment associated side effects, a significant improvement compared to other melanoma therapies such as dacarbazine or ipilimumab [199, 202, 203]. Recently, a Phase III trial of nivolumab confirmed its high response rate, durable and rapid response, and improved safety profile, leading to FDA approval in December 2014 for melanoma refractory to ipilimumab, vemurafenib, and other available melanoma treatments [202, 204–206]. Dosing of nivolumab is 3 mg/kg intravenously every 2 weeks, until disease progression or side effects become unacceptable.
Pembrolizumb, formerly known as lambrolizumab, is another anti-PD-1 humanized monoclonal antibody. In a Phase I trial, pembrolizumab was shown to have an overall response rate of 38 %, with the response rates reaching 52 % in the study cohort that received the highest dose treatment [205]. This trial did not exclude patients who had previously been exposed to ipilimumab and demonstrated that ipilimumab exposure did not affect response rate to pembrolizumab. In Phase II trials, pembrolizumab increased progression free survival was comparable to traditional chemotherapy in patients with metastatic melanoma who had previously failed ipilimumab and/or BRAF inhibition [207]. Through the FDA Fast Track Program, pembrolizumab was approved in September 2014 [208]. Phase III trials for pembrolizumab are also underway. Pembrolizumab is given to the patient as a 2 mg/kg intravenous infusion every 3 weeks until disease progression or when side effects become unacceptable.
Other attempts to target this pathway include the development of antibodies to PD-L1. A Phase I trial of BMS-936559, a fully humanized monoclonal antibody against PD-L1, demonstrated efficacy in patients with metastatic melanoma, with an average response rate of 17 % in all treated patients with various dosing regimens, and a maximum of 29 % response rate for patients treated at a dose of 3 mg/kg, with more than half of responders having a durable response for more than 1 year [209]. Adverse reactions attributed to treatment included fatigue, infusion reaction, rash, and potential immune mediated reactions such as hypothyroidism, hepatitis, myasthenia gravis, sarcoidosis, endophthalmitis, and diabetes mellitus. 61 % of patients had adverse reactions, although most reactions were low grade, and only 11 % of patients required discontinuation of therapy due to side effects [209].
Combination Therapy
Significant interest exists in combining CTLA-4 blockade with BRAF inhibition in the treatment of BRAF V600E harboring melanoma because of the differences in their mechanisms of action. In addition, pre-clinical studies have shown that BRAF inhibition enhances T-lymphocyte recognition of melanoma, suggesting the combination of the two treatments may be synergistic [210–212]. A Phase I study in the United States of this concurrent therapy was unfortunately terminated due to hepatotoxicity [212]. However, an Italian study has shown that sequential treatment with vemurafenib followed by ipilimumab, or ipilimumab followed by vemurafenib, appears to be more tolerable while retaining improved efficacy over single agent therapies [213]. Hodi et al. also demonstrated benefits with combining ipilimumab and GM-CSF. In the study arm with ipilimumab plus GM-CSF, overall survival at 1 year was 68 %, versus 51 % in the study arm with ipilimumab alone. There was no significant difference in adverse events between the different treatment arms in this trial [214].
Combination blockade of both CTLA-4 and PD-1 has been studied in a recent Phase I trial of nivolumab and ipilimumab. Wolchock et al. reported that, at maximum acceptable doses of nivolumab and ipilimumab, 53 % patients with metastatic melanoma had an objective responses, all with tumor reduction of greater than 80 % [215].
Similarly, there are significant interests in combining CTLA-4 blockade with PD-1 inhibitors, taking advantage of a complementary blockade of different T-cell checkpoint pathways to enhance antitumor immunity [216]. In a large multicenter, randomized controlled Phase III study of 945 patients with unresectable stage III or IV melanoma, combination nivolumab and ipilimumab therapy led to a median progression-free survival of 11.5 months, compared to 2.9 months with ipilimumab alone, and 6.9 months with ipilimumab [217]. In a subgroup analyses of patients with tumors positive for PD-L1, combination of nivolumab and ipilimumab therapy led to a progress-free survival of 14.0 months [217]. This combination of therapy, however, was noted to have increased risks of treatment-related adverse events, most commonly diarrhea, fatigue, pruritus, and rash, and these adverse events led to discontinuation of therapy for 36 % of patients, compared to 8 % for nivolumab therapy alone, and 15 % for ipilimumab therapy alone [217].
Adoptive Immunotherapy
Adoptive cell therapy (ACT) against melanoma antigens is achieved by the isolation of immunocompetent T-lymphocytes from patients, in vitro expansion of specific subsets of these cells, and then re-infusion of these T-lymphocytes back into patients. The source of infused lymphocytes can be derived from the tumor itself, which yields tumor infiltrating lymphocytes (TILs), from lymph nodes draining the site of the primary lesion, or from the peripheral blood. Several clinical trials have investigated various types of adoptive immunotherapy as treatment for metastatic melanoma, as well as a possible adjuvant therapy for less advanced disease [218–221]. Early studies demonstrated that tumor specific cytotoxic T-cells harvested from lymph nodes draining areas of melanoma showed little or no tumor specific cytotoxic activity, but that culture of these cells with irradiated autologous tumor cells in the presence of IL-2 rendered them cytotoxic to autologous tumor [222]. Treatment regimens for melanoma have included intravenous infusion of tumor infiltrating lymphocytes with IL-2 and intralesional injection of IL-2-cultured lymphoid cells. These methods have shown promise in small clinical trials [223–226]. IFN-α has also been tested in Phase I/II clinical trials as a suitable replacement for IL-2, with a far less toxic side effect profile [227]. More recently, in a pooled analysis, response rates to ACT for metastatic melanoma have been reported to be 50 %, with complete response in 20 % of patients. For complete responders, 95 % remain disease free at 5 year follow-up [228, 229].
One primary limitation of ACT has been the inability to generate a sustained level of tumor infiltrating lymphocytes in patients undergoing therapy. This is a significant setback, as the persistence of tumor infiltrating lymphocytes has been correlated with clinical response for in vitro trials [230]. Circulating myeloid cells in the peripheral blood, as well as Treg cells, suppress CD8+ T-lymphocyte function and proliferation, decreasing the clinical response to ACT [231, 232]. As a result, myeloablation and lymphodepletion prior to T-lymphocyte transfer have been shown to improve success with ACT [233, 234].
Chimeric antigen receptors (CARs) are constructed of antibody single-chain variable fragment combined with T-cell receptor (TCR) and T-lymphocyte costimulatory domains. The variable fragment of the antibody single chain can be engineered to target a tumor surface antigen, allowing T-lymphocytes to recognize tumor cells and proliferate independent of MHC function and without the need for additional costimulatory signals [235]. This approach has been successful in hematologic malignancies and studies are ongoing to investigate the coupling of ACT with CAR in metastatic melanoma [228, 235, 236].
Specific Immunotherapy
Specific immunotherapy agents stimulate the host immune system to recognize and destroy neoplastic cells bearing tumor-associated antigens. Also referred to as active specific immunotherapy, this approach often involves vaccination with modified melanoma cells or melanoma-derived antigens. Melanoma vaccines are currently under investigation for the treatment of advanced metastatic melanoma (Stage IV) and as an adjuvant treatment of patients at high risk for metastatic disease after surgical treatment (Stage II and III). Multiple trials with melanoma vaccines have been completed in the past decade, although there has been minimal overall success in Phase III clinical trials.
Autologous Vaccines
One approach to generate a specific host immune response is to vaccinate patients with their own tumor cells or cellular lysates made from their own tumor cells, known as autologous vaccination. For this procedure, melanoma tumor cells are harvested from the patient, processed in vitro (often in combination with an immunostimulatory agent), and injected subcutaneously back into the patient as an autologous vaccine. The theoretical advantage of this technique is that it targets the specific antigens found on the patient’s tumor cells. Some of the major limitations to this approach are the number of tumor cells required for vaccine preparation and the labor-intensive and technically challenging nature of culturing and expanding the patient’s melanoma cells.
Early randomized trials with autologous whole tumor cells plus BCG (Bacille Calmette-Guérin) failed to demonstrate improvement in overall outcomes [237–239]. Modulating a patient’s tumor cells to increase the immune response has been attempted by haptenation of tumor cells with dinitroflourobenzene (DNFB). Administering these modified tumor cells, after sensitizing the patient to DNFB, appears to increase immune response and potentially survival in patients who develop cell-mediated immunity to tumor antigens [240, 241]. Additional work has focused on extraction of a patient’s heat shock proteins from melanoma cells to create an autologous vaccine [242]. The vaccine, known as OncophageTM, consists of autologous tumor-derived heat shock protein gp96. In clinical trials, HLA restricted T-cells against the vaccine were induced in 50 % of patients, but the tumor response was modest at best [243, 244]. Taking advantage of the anti-tumor properties of GM-CSF by transfecting autologous melanoma cells with the gene for this molecule has also been explored [245, 246]. Using retrovirus mediated gene transfer for transfection, investigators have been able to create an autologous vaccine that increases tumor necrosis and invasion by immune cells in early studies [245, 246].
Allogeneic Vaccines
An alternative approach to autologous melanoma vaccines is the use of tumor vaccines derived from allogeneic melanoma cells. The goal of this approach is to stimulate the host immune system with a variety of common melanoma antigens. In addition to making a vaccine that would be widely available to many patients, the foreign MHC expression on the surface of these tumor cells further augments the patient’s antitumor immune response. Multiple investigators have initiated clinical trials utilizing allogeneic vaccines as monotherapy for metastatic disease, as well as adjuvant therapy after surgical excision of the tumor. Improvement in time to disease progression was shown in a double-blind, placebo controlled trial of a polyvalent shed antigen vaccine. This small trial of 38 patients demonstrated a 2.5 times increase in time to disease progression, from 0.6 to 1.5 years, in the vaccine group [247]. Melacine, a vaccine derived from two melanoma cell line lysates administered with a detoxified end toxin adjuvant, has been studied in Phase III clinical trials, with no improvement in outcomes in advanced disease as compared to chemotherapy [248]. In a large trial studying this vaccine as adjuvant therapy in Stage II disease, no improvement was noted in relapse free survival [249], although a statistically significant improvement was seen in patients expressing HLA A2 or HLA C3 [250]. Canavaxin, an allogeneic vaccine derived from three melanoma cells lines mixed with BCG, also failed to show success in Phase III trials [251].
Viral Oncolysates
Administration of viral oncolysates is another form of specific immunotherapy that has shown potential in stimulating a clinical anti-melanoma response when used as adjuvant therapy [252]. Viral oncolysates are produced by infecting cultured melanoma cells with a virus to generate a mixture of lysed cells plus virion that can be administered as vaccines. Vaccinia virus melanoma cell oncolysates have shown promise in Phase I/Phase II studies in patients with Stage I and Stage II melanoma, but not in a Phase III study with Stage III melanoma patients [253]. Previous studies have demonstrated that patients with Stage III metastatic melanoma with palpable lymph node disease have responded to post-surgical vaccination with Newcastle disease virus (NDV) melanoma cell oncolysate [254, 255]. This group of patients treated with allogeneic melanoma cell oncolysates produced by the 73 T NDV strain had a 55 % survival at 15 year follow-up as compared to historical controls with 6–33 % 10 year survival rates [256]. The anti-tumor mechanisms of action triggered by NDV oncolysate are unknown, although some studies indicate that it may act in part through the induction of certain cytokines with anti-tumor properties [257].
Talimogene laherparepvec (T-VEC, OncoVEX) takes a different approach to viral oncolytic vaccination. T-VEC is a bioengineered strain of herpes simplex virus type 1 (HSV-1) that expresses human GM-CSF. After injection directly into melanoma, the virus replicates and causes a direct lytic effect, while the GM-CSF produced by the virus attracts and matures APCs [258, 259]. The APCs, after phagocytosing lysed melanoma cells, prime the T-lymphocyte mediated immune response to melanoma [259]. Of note, the virus has been engineered so as not to infect host cells [260]. In Phase II clinical trials, a 26 % response rate was cited, with 1 year overall survival of 58 % and 2 year overall survival of 52 % [261]. The Oncovex Pivotal Trial in Melanoma (OPTiM) trial is an on-going randomized Phase III trial; preliminary data suggests clinical response with T-VEC is 26 %, compared to 6 % in the treatment group of treatment with GM-CSF. Fatigue, chills, and pyrexia were the most common adverse events [262].
Peptide Vaccines
The identification of genes encoding melanoma specific antigens has led to their use in specific immunotherapy. As previously discussed, these are antigens that are expressed on cells of melanocytic lineage or melanoma cells that have been shown to play a role in tumor immunology. Multiple clinical trials have studied the effect of immunization against melanoma peptides such as gp100 [263], MAGE [264], MART [265] and tyrosinase in patients with advanced melanoma [266]. Positive clinical outcomes with peptide vaccines have been few, as obstacles such as tumor escape, preexisting neutralizing antibodies and antigen loss variants hamper vaccine success [17]. A multicenter randomized controlled trial demonstrated some success with the gp100 vaccine combined with IL-2 in patients with localized advanced Stage III melanoma or Stage IV metastatic melanoma. Clinical responses were 16 % in the treatment group, compared to 6 % in the control group that received IL-2 only, with progression free survival increased from 1.6 to 2.2 months [267].
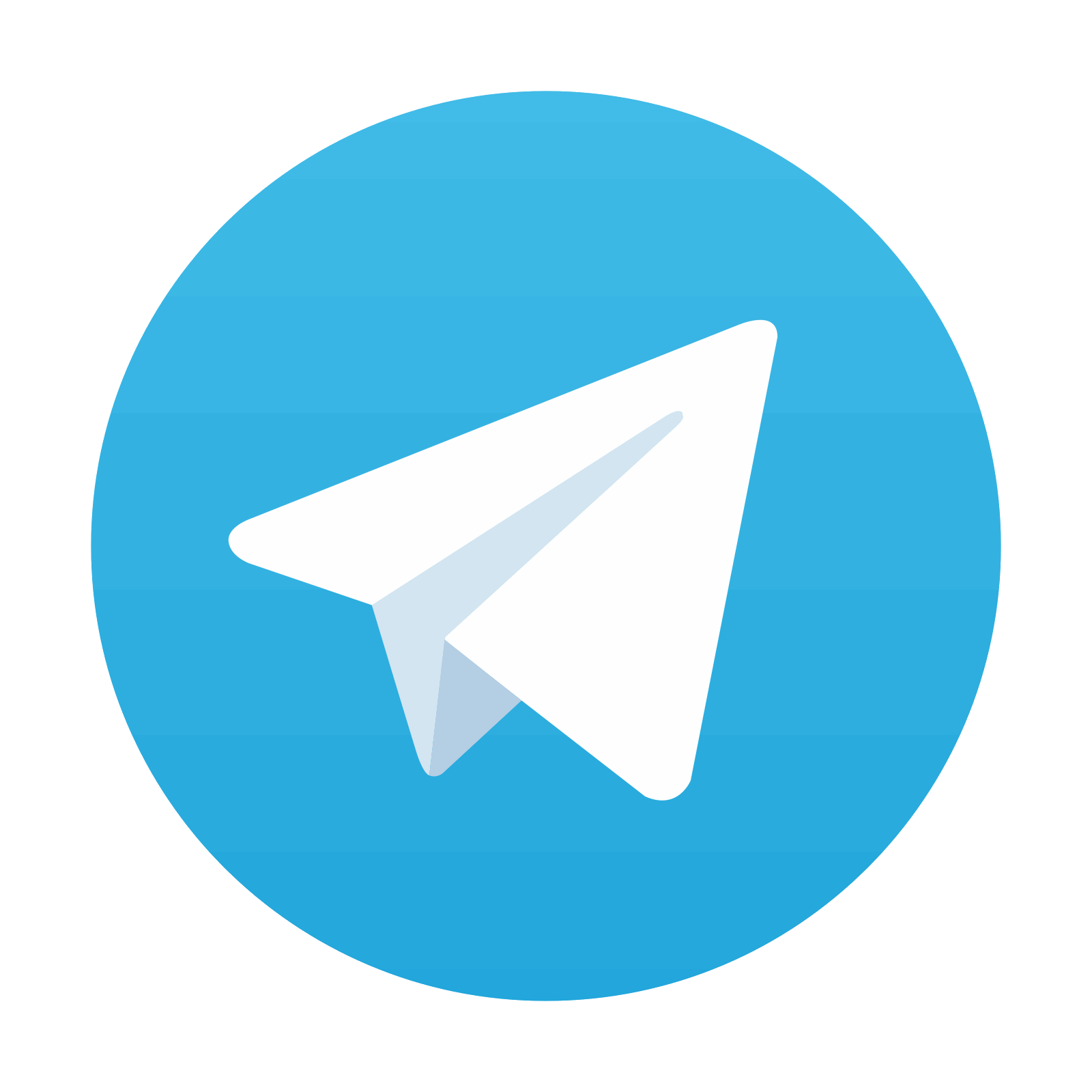
Stay updated, free articles. Join our Telegram channel

Full access? Get Clinical Tree
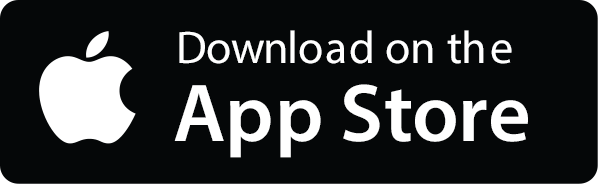
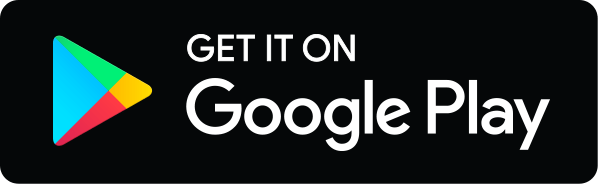
