This article reviews the history of hair follicle regeneration from follicular fragments and dissociated cells. The challenges of trichogenic in vitro culture and subsequent delivery into the patient are discussed, as well as cosmetic acceptance, recent achievements on regeneration of human hair follicles, and new potential cell sources for hair regeneration.
Key points
- •
Hair follicles can be regenerated from cultured cells.
- •
Culture conditions impact the trichogenic property of cells.
- •
The delivery method impacts the potential for a cell-based therapy to produce follicle outgrowth.
- •
Advances in cell biology provide the possibility of follicle regeneration from additional cell sources.
Introduction
The most common form of hair loss is androgenetic alopecia (AGA). Those affected by AGA display an increased sensitivity to androgens, which leads to reduced follicular diameter. Orientreich was the first to show that transplanted hair follicles retain the characteristics of their original location (namely androgen resistance when placed into an androgen-sensitive area). This concept is termed donor dominance. The growth pattern dominance of the follicular origin over recipient site placement has enabled surgical hair transplantation to be one of the most effective treatments to date. Despite the effectiveness of modern hair transplantation, there are challenges associated with this method such as the limitation of donor hair follicles. Currently, medical treatments attempting to bypass the need for additional donor follicles include minoxidil, a potassium channel agonist, and finasteride, an inhibitor of 5 alpha- reductase. These drug therapies require continual use and can be associated several adverse effects. More recently, hair loss therapies based on the cellular components of hair follicles and the growth factors that drive the behavior of those cells have become foci of research efforts. Autologous cell-based therapies offer a unique solution requiring the potential of single treatment and the possibility of no significant systemic adverse effects. Several advances have been made in the fields of stem cell biology and regenerative medicine in the last decade. These advances have laid the foundation to develop new strategies to treat hair loss. The possibility now exists to harvest a small number of hair follicles from the occipital area of the patient, expand the trichogenic, or hair follicle growth-inducing, cell population from this tissue, and transfer these cells back to the patient as a regenerative treatment. Aderans Research Institute Incorporated (ARI, Marietta, GA) and Replicel Life Sciences Incorporated (Vancouver, Canada) are currently conducting separate clinical trials with cell-based solutions for alopecia. Similar clinical trials have also been undertaken by Intercytex, Incorporated. Additionally, Histogen Incorporated (San Diego, CA) and Follica Incorporated (Cambridge, MA) are both conducting phase 1 clinical trials exploring the use of molecular growth factors aimed at the reinvigoration of dormant follicles. These small molecules are known agonists of follicular formation and growth pathways (eg, Wingless-related integration site [WNT], Sonic hedgehog [SHH], and fibroblast growth factors [FGF]).
Unlike most organs, hair follicles do not reach homeostasis once they mature. Instead there is a continuous cycle of growth, regression, shedding, and regeneration throughout life. This inherent ability of hair follicle cycling lends credence to the idea of regenerative cell populations existing in the hair follicle itself. Several groups have demonstrated regeneration of hair follicles using cells dissected from the dermal papilla and dermal sheath of the hair follicle, as well as follicular epidermal cells to form all lineages of the hair follicle in animal models. This article addresses the history of hair follicle regeneration from follicular fragments and dissociated cells. The challenges of trichogenic in vitro culture and subsequent delivery into the patient are discussed, as well as cosmetic acceptance, recent achievements on regeneration of human hair follicles, and new potential cell sources (skin derived precursor cells [SKPs], Induced pluripotent stem cells [iPS]) for hair regeneration.
Introduction
The most common form of hair loss is androgenetic alopecia (AGA). Those affected by AGA display an increased sensitivity to androgens, which leads to reduced follicular diameter. Orientreich was the first to show that transplanted hair follicles retain the characteristics of their original location (namely androgen resistance when placed into an androgen-sensitive area). This concept is termed donor dominance. The growth pattern dominance of the follicular origin over recipient site placement has enabled surgical hair transplantation to be one of the most effective treatments to date. Despite the effectiveness of modern hair transplantation, there are challenges associated with this method such as the limitation of donor hair follicles. Currently, medical treatments attempting to bypass the need for additional donor follicles include minoxidil, a potassium channel agonist, and finasteride, an inhibitor of 5 alpha- reductase. These drug therapies require continual use and can be associated several adverse effects. More recently, hair loss therapies based on the cellular components of hair follicles and the growth factors that drive the behavior of those cells have become foci of research efforts. Autologous cell-based therapies offer a unique solution requiring the potential of single treatment and the possibility of no significant systemic adverse effects. Several advances have been made in the fields of stem cell biology and regenerative medicine in the last decade. These advances have laid the foundation to develop new strategies to treat hair loss. The possibility now exists to harvest a small number of hair follicles from the occipital area of the patient, expand the trichogenic, or hair follicle growth-inducing, cell population from this tissue, and transfer these cells back to the patient as a regenerative treatment. Aderans Research Institute Incorporated (ARI, Marietta, GA) and Replicel Life Sciences Incorporated (Vancouver, Canada) are currently conducting separate clinical trials with cell-based solutions for alopecia. Similar clinical trials have also been undertaken by Intercytex, Incorporated. Additionally, Histogen Incorporated (San Diego, CA) and Follica Incorporated (Cambridge, MA) are both conducting phase 1 clinical trials exploring the use of molecular growth factors aimed at the reinvigoration of dormant follicles. These small molecules are known agonists of follicular formation and growth pathways (eg, Wingless-related integration site [WNT], Sonic hedgehog [SHH], and fibroblast growth factors [FGF]).
Unlike most organs, hair follicles do not reach homeostasis once they mature. Instead there is a continuous cycle of growth, regression, shedding, and regeneration throughout life. This inherent ability of hair follicle cycling lends credence to the idea of regenerative cell populations existing in the hair follicle itself. Several groups have demonstrated regeneration of hair follicles using cells dissected from the dermal papilla and dermal sheath of the hair follicle, as well as follicular epidermal cells to form all lineages of the hair follicle in animal models. This article addresses the history of hair follicle regeneration from follicular fragments and dissociated cells. The challenges of trichogenic in vitro culture and subsequent delivery into the patient are discussed, as well as cosmetic acceptance, recent achievements on regeneration of human hair follicles, and new potential cell sources (skin derived precursor cells [SKPs], Induced pluripotent stem cells [iPS]) for hair regeneration.
Regeneration of the hair follicle from dissected fragments
One solution to increase the number of possible donor follicles is to divide up the hair follicle into dissected fragments in the hope that upon transplantation each fragment would develop into a complete follicle. While horizontal division of the hair follicles has resulted in a complete hair follicle from the upper two-thirds, the lower one-third was not able to produce a follicle. A second study using transverse division produced hair follicles from only one of the upper implants. The studies of these approaches were limited in size.
Another approach, using microdissected follicular dermal papillae (DP) or connective tissue sheaths (CTS), was able to regenerate follicles upon insertion into afollicular skin. In people, the transplantation of male scalp dermal sheath (DS) fragments into female forearm skin resulted in follicular growth with male dermal lineage.
The major caveat to these methods is the poor efficiency of regeneration (ie, they did not result in increase in the resultant number of hairs compared with the number of follicles used). The numbers of hairs were not increased, because at least 1 DP, DS, or CTS is required to form a new hair follicle. Hence, the major contribution of this research was the realization that cells within dissected follicular fragments are able to reorganize and induce new hair follicles or follicular growth. These experiments prompted additional research investigating the cells derived from these follicular fragments and the development of in vitro culture methods directed at trichogenic cell proliferation and maintenance of their inductive capabilities.
Regeneration of the hair follicle from dissociated cells
A second approach, designed to create more hair follicles from the same number of donor follicles, consists of dissociated cell culture. Regeneration using dissociated cells has been tested in various models, mostly involving embryonic and neonatal mouse cells. The chamber assay uses dissociated mouse neonatal dermal (MND) and mouse neonatal epidermal (MNE) cells combined (to a total of 10 million cells) and delivered into a silicon chamber already grafted onto the back of an immunocompromised (nu/nu) mouse. After 1 week, the chamber is removed to ultimately yield tufts of mouse hair on the back of the host mouse after 1 month, thereby indicating the trichogenic potential of the cells ( Fig. 1 ). Zheng and colleagues reported on the patch assay in 2005, which consists of a mixture of MND and MNE injected into the dermis of nu/nu mouse dorsal skin to generate hair after 10 to 14 days. Upwards of 10 samples were injected into a single mouse, with each injection using only 2 million total cells. Variations included placement of the cells into a kidney capsule or into a trachea. In 2008, Qiao and colleagues used a flap assay (a modification of the chamber assay) involving the use of a skin flap.
Recently, Lee and colleagues combined aspects of the chamber assay and trichogenic patch assay to create a reconstituted hair assay in a nu/nu mouse model. Hair was formed using dissociated cells placed under a membrane over a wound created on the dorsal side of the mouse. The technique is simpler and more efficient than the chamber assay and, although less efficient than the trichogenic patch, this method results in hair follicles observable from the surface of the skin. The graft can be made with flexibility into different shapes and sizes depending on cosmetic needs.
Hair follicles regenerated using dissociated cells in the patch assay contain pigmented shafts complete with sebaceous glands and are characteristic of the donor ( Fig. 2 ). The newly generated follicles cycle normally through anagen, catagen, and telogen. Generation of hair follicles in the patch assay as well as the chamber assay continues through multiple cycles when they are allowed to egress to the surface of the skin.
Trichogenic maintenance of dissociated cells
Initial studies took advantage of the intrinsic trichogenicity of cells isolated from dissociated tissue. Most successful in vivo hair reconstitutions from dissociated cells are from freshly isolated cells not cultured, expanded cells. Therefore, it is crucial to maintain inductive activity of cells in vitro as the cell number is expanded.
Rat vibrissa dermal papilla cells were first cultured in 1981 by Jahoda and Oliver and later by Messenger in 1984. Although DP and DS cells can generate new hair follicles when implanted, they lose their trichogenic potential rapidly following in vitro culture. Maintenance of trichogenicity in vitro has concentrated on culture medium supplementation and the retention of a follicular DP gene expression profile.
The addition of Wnt3a protein to culture media extended the inductivity of dermal cells in vitro. Adding bone morphogenetic protein (BMP) to cultured mouse DP prolonged in vitro DP-specific marker expression and trichogenicity. Osada and colleagues discovered that basic fibroblast growth factor (FGF2) is critical to mouse vibrissae maintenance in vitro.
An interesting characteristic of early DP culture is that the cells primarily grow in aggregates immediately following isolation from tissue. This phenomenon is lost throughout culture and may suggest a link between aggregation and trichogenic activity. Cultured mouse vibrissae DP cells lost their aggregation and trichogenicity over time in culture but recovered their trichogenicity, as well as DP-specific marker expression, following aggregation to spheres.
In the mouse model, the relationship between follicular dermal cells and aggregation was further exploited by Fernandes and colleagues. Miller’s group demonstrated that a highly multipotent cell type, termed SKPs, are derived from the follicular portion of mouse dorsal skin. These cells self-select under appropriate culture conditions to form spheres. The cells that make up the spheres trace their origin to the follicular DP and DS and also express DP-specific markers SOX2, nestin, fibronectin, and vimentin. SKP cells can be passaged multiple times and are able to form new hair follicles in the patch assay as well as homing to and incorporating into existing follicles in a wound assay.
Work by Shimizu and colleagues investigated the impact of aggregative culture on lung fibroblasts. The group discovered that aggregation upregulated LEF1, a transcription factor associated with the WNT pathway, a known follicular pathway. More striking was that long-term aggregative culture resulted in lung fibroblasts inducing hair formation in the patch assay, demonstrating the plasticity of cultured fibroblasts induced by their culture conditions.
Kang and colleagues used aggregative culture techniques (spheroid culture) to restore the inductive ability of human DP cells. In these experiments, late-passage DP cells (P10) regained their inductive ability when cultured and assayed as spheroids. Clearly the microenvironment created by a 3-dimensional culture helps to maintain and induce trichogenic properties in dermal cells.
The majority of the work investigating cell based follicular neogenesis has focused on retaining or enhancing the trichogenicity of the dermal follicular progenitor cells. Much progress has been made to maintain dermal follicular progenitor cell trichogenicity; however, very little attention has been paid to the epidermal cells. Recently, Atit’s group clearly showed that the original signal resulting in hair follicle morphogenesis is initiated by the epidermal cells; therefore, it will be crucial to study the role of epidermal cells in follicle neogenesis and develop appropriate assays to evaluate the trichogenicity of epidermal cells. Recently, ARI improved the epidermal culture conditions of mouse neonatal cells. The improved culture condition resulted in an epidermal cell that was able to form an epidermal basal layer when injected in vivo. With this enhanced epidermal cell preparation, ARI was able to create a mouse follicle using cultured mouse-derived epidermal and dermal cells for the first time (Xunwei Wu, unpublished data, 2012).
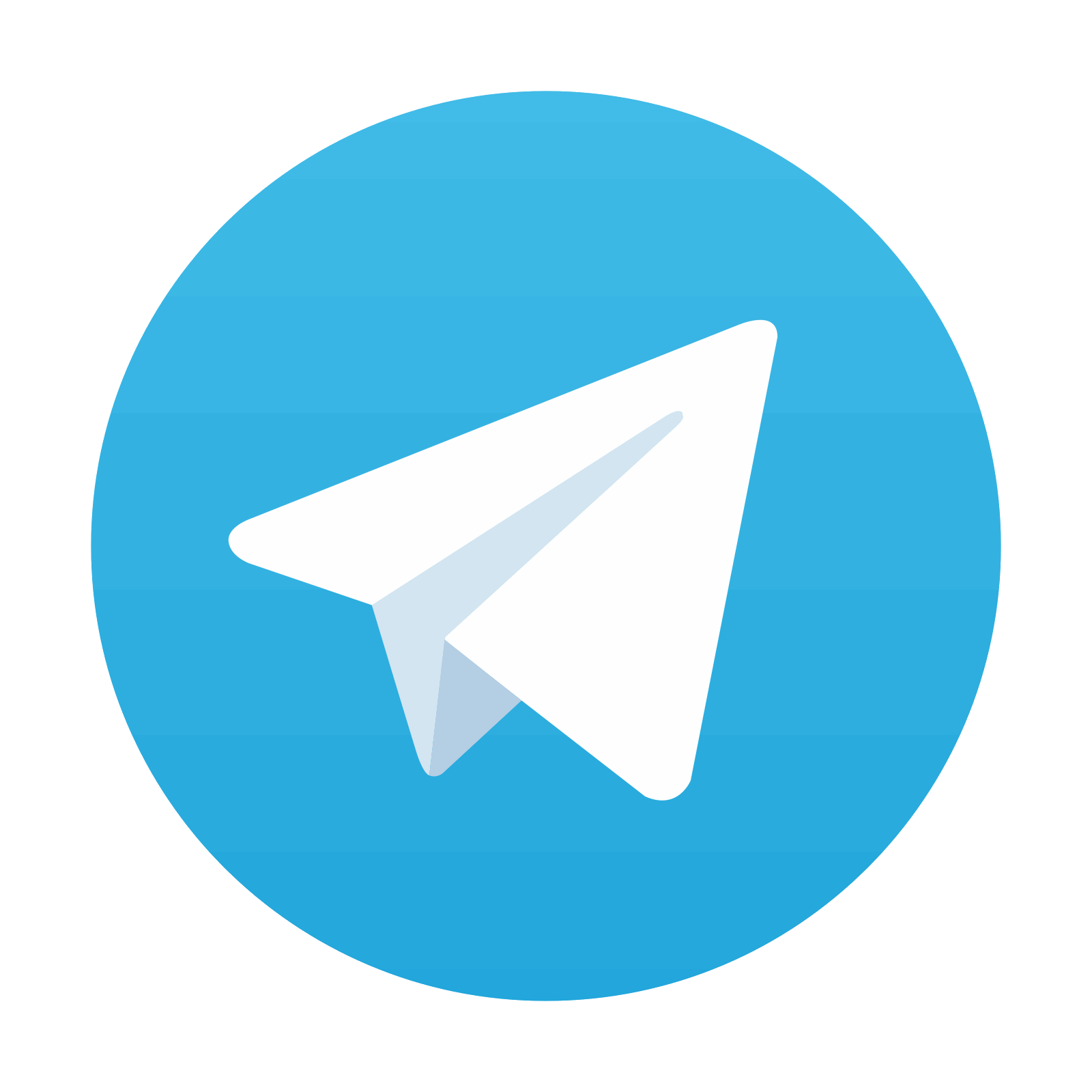
Stay updated, free articles. Join our Telegram channel

Full access? Get Clinical Tree
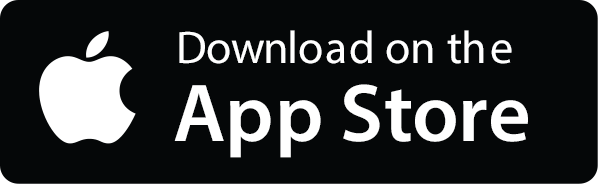
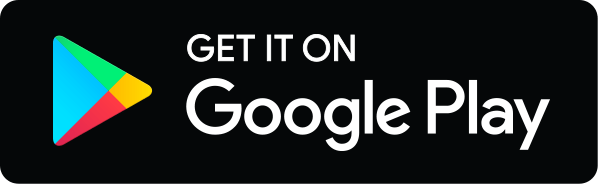