Fig. 19.1
Possible pathways for immunologic responses stimulated by fungi. (a) Most fungi are detected and destroyed within hours by innate nonspecific defense mechanisms mediated by phagocytes such as macrophages, neutrophils, immature dendritic cells, and opsonins (antibodies, Ab) through the involvement of distinct pattern-recognition receptors (PRRs). Fungal organisms on the skin surface also release antigens (Ag) that penetrate the skin and are captured by an antigen-presenting cell (APC) such as dendritic cells (DCs). Cross-linking of PRR on the surface of immature DCs by fungal antigen lead to their maturation. In addition, production of inflammatory cytokines such as IFN-γ and TNF-α by other innate cells such as NK cells further enhance activation of microbicidal functions of phagocytic cells as well as maturation of DCs. The DCs sampling fungal antigens from the skin and migrating to secondary lymphoid organs process and present antigens through class I or class II major histocompatibility complex (MHC) molecules to antigen-specific naive T cells endowed with the capacity to recognize the peptide epitopes through specific T-cell receptors (TCRs). This process lead to activation of different antigen-specific T helper (Th) effector cells, regulatory T (Treg) cells and B cells that specifically target the pathogen and induce memory cells. Differentiation of naive CD4+ Th cells in the peripheral lymphoid organs into Th1, Th2, or Treg depend on several factors, among which is the cytokine environment stimulated by different fungal morphotypes. Thus, the production of interleukin-12 (IL-12) by DCs leads to the outgrowth of T-helper-1 (Th1) cells that produce IFN-γ, TNF-α, or both. IFN-γ and TNF-α are required for further activation of fungistatic and fungicidal activities by phagocytes that results in clearance of infection with most, if not all, of these fungal pathogens. The induction of IL-4 (and failure to produce IL-12) by DCs leads to a Th2 response, which blunts the generation of protective immunity. (b) Progressive disease in immunodeficient or susceptible hosts is associated with a shift in the balance between Th1 and Th2, toward the Th2 response. The latter is characterized by upregulation in IL-4, IL-5 and IL-10, an increase in tissue eosinophils, antibody isotype switch and production of antigen-specific antibodies including IgG and IgE. The IgE antibodies bind to mast cells (MCs) and upon subsequent encounter with allergens, trigger degranulation leading to inflammation and clinical features of type I hypersensitivity reactions. IL-10 production by mast cells suppresses cell-mediated immune responses in certain cutaneous fungal diseases. Neutralization of IL-4, IL-5, and IL-10 in vivo can sometimes restore protective immunity. Thus, the activation of the appropriate Th-cell subset is critical in the generation of a successful immune response to fungi. Although IL-4 and IL-10 cytokines block the expression of a protective response against fungi, the elaboration of at least some Th2 cytokines also helps to balance the immune response. Finally, induction of T-regulatory (Treg) cells mediated by IL-10 might serve to dampen the excessive inflammatory reactions through cell contact or secretion of immune suppressive cytokines such as IL-10 (b)
Host innate defenses against fungi are mediated by professional phagocytes, including neutrophils, mononuclear leukocytes (monocytes and macrophages), and dendritic cells (DCs), natural killer (NK) cells, and nonhematopoietic cells, such as keratinocytes and epithelial and endothelial cells. The first step in the innate immunity involves fungal recognition and uptake by germline-encoded pattern recognition receptors (PRRs) expressed on the surfaces of several innate immune cells [8–11].
Figure 19.2 illustrates the different PRRs expressed on phagocytic cells such as macrophages and the downstream effector functions resulting from interaction of fungal antigens with these receptors.


Fig. 19.2
Recognition of fungal ligands by different pattern recognition receptors (PRRs) expressed on the surface of macrophages: the role of Toll-like receptors (TLRs) and other receptors as activators of innate and adaptive immunity to fungi. (a) Innate cells such as macrophages, monocytes, and dendritic cells express several pattern recognition receptors (PRRs) that recognize various fungal ligands, promote fungal internalization, activate intracellular fungicidal effector mechanisms, and play a role in the induction of the acquired immune response against fungi. Concomitant interaction of antibody and complement-coated fungal cells with Fc receptors (FcRs) and complement receptors (CRs) on host phagocytic cell membranes results in prompt ingestion of the fungal cell, which can lead to the death of the ingested fungal cell. Furthermore, phagocytic cells express several TLRs that bind to specific fungal ligands referred to as pathogen-associated molecular patterns (PAMPs). The signaling pathway for mammalian TLRs after ligation of PAMPs involves interaction with the adaptor molecule MyD88 (myeloid differentiation primary response gene 88) located in the cytosol. The activation of MyD88 results in activation and translocation of nuclear transcription factor
controls the activation of several downstream cytokines and chemokine genes; therefore, its activation is usually linked to production of proinflammatory and antiinflammatory cytokines and chemokines. Although all TLRs signal through MyD88, ligation of certain TLR can result in unique effector functions. For example, TLR2 stimulation leads to production of IL-10, which promotes the expansion and function of immunoregulatory T cells. On the other hand, stimulation of TLR4 or TLR9 leads to the activation of antifungal effector functions in phagocytes, such as respiratory burst and degranulation, and production of interleukin-12p70 (IL-12p70) by dendritic cells. This leads to inflammatory and protective antifungal T-helper-1 (Th1)-cell responses. However, the differential TLR responses could also function by unidentified MyD88–independent pathways (b)

The most important classes of PRR are the Toll-like receptors (TLRs) [10, 11], dectin-1 [12], the lectin-like receptors [13, 14], Fc receptors [15], complement receptors, the mannose receptor [16], and integrins [17].
The microbial ligands of these receptors are called pathogen-associated molecular patterns (PAMPs). Fungal structures such as β-1,3/β-1,6 glucans [17], glucuronoxylomannan, phospholipomannan, and galactomannan function as ligands for TLR2, TLR4, and TLR6 [18, 19]. The signaling pathway for mammalian TLR after ligation of PAMPs involves interaction with the adaptor molecule MYD88 (myeloid differentiation primary response gene 88) located in the cytosol [19, 20]. The activation of the MYD88 adaptor culminates in the activation and nuclear translocation of nuclear factor kB (NF-kB), which leads to activation of several cytokine and chemokine genes. Recognition of pathogens by TLR in a Myd88-dependent, and sometimes in a Myd88-independent, manner leads to release of proinflammatory cytokines, chemokines, activation of antibacterial mechanisms, and enhancing the T-cell priming ability of professional antigen presenting cells (APCs) such as dendritic cells. Compared to individual TLRs, Myd88−/− mice had higher levels of fungal growth than control animals [19, 21]. The more severe phenotype of Myd88−/− mice compared with mice deficient in individual TLRs probably reflects the broad function of Myd88 as an adaptor for multiple TLR-dependent responses.
Toll-like receptors discriminate between distinct fungal morphotypes. For example, TLR4 and CD14 expressed on human monocytes appear to recognize Aspergillus hyphae but not Candida hyphae [17, 22].
Similarly, the production of pro-inflammatory and Th1 cytokines such as tumor necrosis factor-α (TNF-α) and interferon-γ (IFN-γ) by macrophages in response to C. albicans phospholipomannan expressed on yeast cells depends on TLR2 and TLR4 signaling, whereas hyphal cells trigger these cytokines in a TLR2-dependent manner only [19, 22–24].
In vivo, the role of TLR2 in systemic fungal infection such as systemic candidiasis is less clear: one study reported that TLR2−/− mice are more sensitive to primary infection than control mice [25], while another study reported no difference between TLR2−/− and TLR2+/+ mice, regardless of whether the animals were inoculated with yeast or hyphal forms [26, 27]. TLR2− mediated recognition of Candida has also been shown to expedite differentiation of hematopoietic stem and progenitor cells into macrophages, which likely helps boost the body’s innate immune response [28]. In addition to their function in fungal recognition, uptake, and production of proinflammatory cytokines, signaling through individual TLR signaling can determine the type of acquired immune responses against fungi. TLR2 ligation by fungal zymosan, and possibly β-glucan, leads to the prevalent production of anti-inflammatory cytokines such as interleukin-10 (IL-10), which can suppress macrophage microbicidal functions and also drive the induction of Th2 response [26, 27, 29, 30].
Lectin receptors are other PRRs on phagocytes that not only play a role in fungal recognition but also mediate distinct downstream intracellular events related to clearance of fungi. Recognition of fungal PAMPs by lectin receptors induces rapid and broad host defense responses such as opsonization, activation of complement, activation of coagulation cascades, phagocytosis, inflammation, and direct microbial killing [31, 32].
Among several lectin-like families, galectin-3 binds to β-1,2-linked oligomannan, an uncommon PAMP present on the surface of C. albicans but absent on Saccharomyces cerevisiae [33, 34]. The binding of galectin-3 to yeast cell walls of C. albicans is inhibited by C. albicans mannans but not by S. cerevisiae mannans. More importantly, binding of galectin-3 results in opsonization of Candida expressing different combinations of β-1,2-linked oligomannosides and death of yeast cells [34] and as such gal3−/− mice are more susceptible to infection, have larger fungal burden and die faster than wild type controls [35]. In addition to its direct effects on innate immunity, galectin-3 also plays an important role modulating the Th1 versus Th2 response in infected tissues [36].
Other fungal receptors such as complement receptors (CRs), mannose receptors (MRs), and dectin-1 receptors mediate fungal internalization following binding of various fungal ligands such as complement-associated products, mannosylefucosyl glycoconjugate ligands, and β-glucans, respectively. Internalization through MRs does not lead to effective clearance of fungi in the absence of opsonins. However, MRs expressed by DCs activate specific programs that are relevant to the development of antifungal acquired specific immune responses as will be discussed later. Ligation of CR3 (also known as CD11b/CD18) is one of the most efficient means of engulfing opsonized fungi, but it also has broad recognition capacity for diverse fungal ligands. Interestingly, yeasts such as Histoplasma capsulatum establish intracellular fungal parasitism within macrophages when they enter the cells through the CR3. In contrast, a concomitant ligation of both CR3 and FcγIIIR on macrophages triggers an effective phagocytosis and respiratory burst that interferes with fungal infectivity and mediates elimination of fungal pathogens [37, 38].
Neutrophils, macrophages, and monocytes constitute the major cellular effectors of innate immunity against fungal pathogens [39–42]. Following phagocytosis, fungi are killed by intracellular microbicidal effector molecules produced by macrophages and neutrophils, including oxygen-dependent (i.e., nitric oxide, reactive oxygen intermediates, reactive nitrogen intermediates, and peroxynitrite) and oxygen-independent (i.e., release of cationic proteins, lysozyme, and antimicrobial peptides such as defensins, arachidonic acid, myeloperoxidase, and iron sequestration) [42–45]. Enzymes such as the reduced nicotinamide adenine dinucleotide phosphate (NADPH) oxidase and inducible nitric oxide synthase initiate the oxygen-dependent pathways known as respiratory burst that produces toxic reactive oxygen intermediates (ROIs) [43–45]. In retribution, fungi have evolved strategies to selectively inhibit the respiratory burst through the production of specific scavengers of oxidative killing by phagocytes, such as catalase, mannitol and melanin.
Patients with inherited X-linked chronic granulomatous disease, resulting from a deficiency in oxidant formation due to mutations in any of the four genes that encode the subunits of NADPH oxidase, have increased susceptibility to fungal infection, mainly aspergillosis [45]. These patients could be treated effectively with IFN-γ, which increases the non-oxidative as well as the oxidative intracellular microbicidal mechanisms mediated by phagocytic cells such as macrophages and neutrophils. The involvement of neutrophils or macrophages in host defense against fungi depends on the morphotype of the fungi causing infection. For example, neutrophils play a predominant role in phagocytosis of filamentous fungi [40–42, 45], while macrophages play a predominant role in host defense against fungal yeast [39, 46]. In addition to the ability of macrophages to ingest organisms that have been opsonized with antibody, or complement, they are also able to phagocytose unopsonized fungal elements through recognition receptors such as the integrins. Although the main contribution of neutrophils and macrophages resides in their phagocytic and microbicidal functions, they can produce cytokines and chemokines that can modulate the protective immune response. Furthermore, macrophages function also as APCs that activate CD4+ and CD8+ T cells through presentation of fungus-derived peptides in the context of major histocompatibility complex (MHC) class II and I, respectively as well as providing co-stimulatory signals as illustrated in Fig. 19.3. Nevertheless, for some intracellular fungal pathogens, such as H. capsulatum, their intracellular location protects them from host defenses, and these organisms thrive within macrophages [37, 38].


Fig. 19.3
Different functions of macrophages in innate and acquired immune responses against fungal infection. Macrophages play a pivotal role in innate and acquired immune responses against fungal pathogens. Local activation of macrophages at early stages of infection by fungal antigens and later by IFN-γ and TNF-α produced by NK cells or effector CD4+ Th1 cells results in (1) increased oxygen–dependent and independent fungicidal activities; (2) production of proinflammatory cytokines and chemokines that enhance migration of effector immune cells to the skin as well as play a role in activation of T cells. In addition, macrophages can also function as professional antigen presenting cells (APC) that process fungal antigens and present fungus-derived peptides in the context of MHC class II and I to CD4+ and CD8+ T cells, respectively
To overcome the fungal immune evasion mechanism within phagocytic cells, other innate immune cells such as NK [46–48], NKT, and γδ T cells [46, 49, 50] play a pivotal role in host defense against fungi. These cells mediate their antifungal response through different mechanisms that include the following: (1) early production of cytokines such as IFN-γ and TNF-α that are important for full activation of macrophage phagocytic and antimicrobial effector functions; (2) direct cytotoxic killing of pathogens or growth inhibition; (3) activation of dendritic cells through either cytokines or cell–cell contact, which in turn mediate activation and differentiation of specific CD4+ and CD8+ T cells as described later. Evidence that supports the protective role of NK, NKT, and γδ T cells in immunity against fungi stems from studies conducted in knockout mice that lack a particular cell subset. These mice are susceptible to various fungal infections, mainly C. albicans [48, 49].
Langerhans cells (LCs) and immature dermal DCs are some of the first cells to encounter fungi and play pivotal roles in induction of acquired responses against fungi as well as restriction of fungal growth [51–53]. Immature DCs constantly monitor the epidermal microenvironment by taking up antigen and processing it into fragments that can be recognized by cells of the adaptive immune response. However, receptors on the surface of DCs may be selective for only certain species, or for certain forms within that species, and may explain in part the ability of fungi to survive within the host. Compared to yeasts, hyphae cause DCs to produce cytokines leading to local induction of T regulatory cells (Treg), which serve to limit the immune response [54].
Because of their unique migratory ability, DCs can transport fungal antigen from the epidermis or dermis to regional lymph nodes, where they initiate specific immune responses. Fungal infection provides danger signals, leading to a local production of proinflammatory cytokines that induce local DC maturation [55, 56]. Maturation of DCs is associated with a selective change in chemokine receptor profile [57–65]. For example, immature DCs express a number of chemokine receptors for inducible chemokines, such as IL-8 (CXCL8), RANTES (regulated on activation, normal T-cell expressed and secreted; CCL5), macrophage inflammatory protein 1α (MIP-1α; CCL3), or monocyte chemoattractant protein 3 (MCP-3; CCL7), by which immature DCs are attracted to the site of inflammation (i.e., skin) [61–63].
Following maturation, DCs downregulate these receptors, which may allow them to leave the inflammatory site (i.e., the site with the highest chemokine concentration). At the same time, maturing DCs upregulate receptors for constitutively expressed chemokines such as the CXC chemokine receptor 4 and CCR7 [62, 63]. Interestingly, the CCR7 ligand secondary lymphoid tissue chemokine (SLC; CCL21) is constitutively expressed by stromal cells in T-cell zones of lymph nodes and by lymphatic endothelial cells in the dermis [61–63]. Thus constitutive expression of SLC by lymphatic endothelium seems to provide the first chemotactic gradient for activated CCR7 positive DCs, leading to a selective recruitment of DCs from the epidermis to the afferent lymphatics [63–65]. Once they enter the lymphatics, they are likely to be transported passively with the lymph to the subcapsular region, where they then encounter an additional chemotactic gradient of SLC that directs their migration into the paracortical T-cell zone of the lymph node where they activate T cells specific for the invading fungal pathogen. In the draining lymph nodes, these DC-capturing antigens initiate T-cell immune responses by virtue of their abilities to present fungal antigens to T cells, provide lymphocytes co-stimulatory molecules, and secrete cytokines. The DCs use distinct receptors to recognize each form of a particular fungus, thereby activating different signaling pathways with distinct functional consequences [55, 65–67].
Finally, the noncellular effectors of innate immunity comprise complement and natural antibodies [68–71]. As described before, these molecules mediate opsonization and therefore promote the ingestion of fungi by phagocytes. However, the fate of opsonized fungi can differ from that of unopsonized organisms, because in phagocytes, opsonized fungi may traffic through a different pathway than do the unopsonized organisms [37, 38].
Adaptive Immunity
For many fungal pathogens, the effective immune response to invasion is a cell-mediated immune response. The role of CD4+ T lymphocytes in protection against fungal infections is underscored by the susceptibility of patients with acquired immunodeficiency syndrome (AIDS) caused by HIV to fungal infections caused by C. albicans, H. capsulatum, Cryptococcus neoformans, and Aspergillus fumigatus. For all of the pathogenic fungi, a T-helper-1 (Th1) response characterized by production of IL-2 and IFN-γ is the dominant adaptive protective response. The production of IL-12 by DCs leads to expansion and increased number of Th1 cells that produce IFN-γ or TNFα [39, 56, 57, 72, 73]. Interestingly, IL-12 secretion seems to be dependent on the morphologic form of the fungi where the yeast form of C. albicans stimulates IL-12 production, while the hyphal form inhibits such production [67, 73]. Interferon-γ production by Th1 cells is essential for optimal activation of phagocytes (e.g., macrophages, neutrophils, and immature DCs) at sites of infection and enhances their fungicidal effector functions [39, 66]. Therefore, deficiency of this cytokine or Th1 response might predispose patients to overwhelming fungal infections, and also favor fungal persistence. On the other hand, the Th2 response characterized by production of Th2 cytokines (IL-4, IL-13, IL-5, and IL-10) is often associated with a subversion of the host response to fungi. Increases in the Th2 cytokines are commonly observed in progressive disease, and neutralizing their activity restores protective immunity [73–77].
The role of Th1/Th2 paradigm in outcome of fungal disease is exemplified in skin infection with Paracoccidioides brasiliensis (PCM), which stimulates the formation of granulomatous lesions in the skin [77, 78]. The presence of well-formed granulomas and local Th1 responses in the skin of PCM patients is associated with mild disease, while the presence of poorly formed granulomas and local Th2 responses is associated with progressive and severe disease. These observations suggest that well-organized granulomas and cutaneous Th1 response reflect a better cellular immune response, while the presence of Th2 cells expressing Th2 cytokine such as IL-4 and IL-5 indicate an ineffective response in PCM skin lesions.
More recently, Th17, a distinct CD4+ T cell outside the family of Th1 and Th2 cells, has been discovered and like Th1 cells can confer a protective effect in fungal infections [79]. In the same manner that different fungal pathogens can induce a Th1 versus Th2 immune response, certain fungal PAMPs predominantly activate a Th17 immune response. Thus far, the understanding of Th17 activation pathways relies on the same signaling pathways (e.g. TLR, dectin-1), though further research may elucidate receptors unique to Th17 development [80]. If not regulated, Th17 cells can yield a deleterious effect [54, 80].
Compared to CD4+ T cells, the role of CD8+ T cells during fungal infection has not been defined as clearly [81]. CD8+ T cells mediate protection against fungal infection mainly via the production of IFN-γ. However; the role of CD8+ T lymphocytes in mediating cytotoxic lysis of fungus-infected target cells is not well delineated [82]. Although CD8+ T cell activity against filamentous fungi such as A. fumigatus has not been demonstrated in mice, the expansion of cytotoxic, class I–restricted, A. fumigatus–specific CD8+ T cell clones from human peripheral blood suggests that CD8+ T cells might contribute to cell-mediated defense [82]. Interestingly, unlike infection with bacteria and viruses, the priming of fungus-specific CD8+ T cells does not appear to require CD4+ T-cell help; in fact, CD8 T-cell responses are enhanced in CD4-knockout mice. In the absence of CD4+ T cells, CD8+ T cells can protect mice from H. capsulatum infections by secretion of IFN-γ [83–85].
Although Th1-biased responses to fungal infections are protective, Th2-biased responses are deleterious; an excess or unregulated Th1 response may also generate unnecessary tissue damage. The Th1 response can be downregulated by simultaneous elaboration of Th2 cytokines or suppression of Th1 and Th2 responses that usually are associated with either chronic fungal infection or overwhelming infection, respectively. Thus, the induction of regulatory mechanisms in immunity to fungi is pivotal as they ensure that under physiologic conditions, an effective protective antifungal immunity is generated while avoiding immune pathology. One important immunoregulatory cytokine is IL-10, which is a potent immunosuppressive cytokine, produced in a non–antigen-specific manner by innate cells such as macrophages and DCs or in an antigen (Ag)-specific manner by regulatory CD4+ T cells [86, 87].
Interleukin-10 acts by impairing (1) the antifungal effector functions of phagocytes; (2) the secretion of proinflammatory cytokines such as TNF-α, IL-1, and IL-6; and (3) the production of Th1-promoting cytokines such as IL-12, IFN-γ, and IL-23 [86–89].
The IL-10-mediated suppressive functions would result in defective protective antifungal cell-mediated immunity. Production of IL-10 at early stages of infection suppresses immune responses and enhances susceptibility to fungal infection.
Histopathologic and double immunohistochemical examination of skin lesions from patients with severe PCM infection revealed an increased number of mast cells expressing IL-10 [90, 91]. Early production of IL-10 by mast cells, as part of the innate system, could contribute to an ineffective response against fungal antigens. It is of interest that IL-10 expressing mast cells were detected only in skin lesions characterized by loose granulomas and local Th2 response, but not in lesions that are characterized by compact granulomas and Th1 response. Other studies have shown that patients treated with anti–TNF-α antibodies, which resulted in increases in IL-10 production are susceptible to fungal pneumonia. On the other hand, production of IL-10 at later stages of infection by CD4 T regulatory cells is beneficial by contributing to resolution of excessive inflammatory responses, thus avoiding immune-mediated tissue damage [88–92].
Role of Antibodies in Protective Immunity Against Fungi
Nearly all fungi elicit an antibody response; however, the role of these antibodies in pathogenesis or protective immunity is not completely clear. Initially, the presence of specific antibodies in patients with progressive fungal infections and the lack of increased susceptibility to fungal infections in patients with antibody deficiencies argued against a protective role. However, studies involving opportunistic fungal diseases, mainly candidiasis and aspergillosis, provided evidence that supports a role for antibodies in protective immunity against fungal diseases [96–99]. More recent evidence suggests that the role of fungal antibodies is dependent on the specificity and isotype, which can yield three distinct host responses: protective, non-protective or indifferent, and disease-enhancing [3]. Possible targets for protective antibodies included fungal cell wall polysaccharides of Candida and Aspergillus species, heat shock protein [94], histone-like proteins, and mannoprotein in C. albicans [96–100].
Recently, another fungal cell-wall polysaccharide, β-glucan, has been identified as a possible target for the induction of protective antibodies [101]. The conserved structure suggested that β-1,3-glucan could be part of a universal antifungal vaccine. In the light of known inverse relationship between Th1 and Th2 cytokines and immunoregulation of the Th1 and Th2 responses, a role for antibodies in defense against fungal disease might seem to be in conflict with the underlying Th1-directed response that is the most widely accepted explanation for host-acquired specific immunity against fungi. However, some studies show that Th2-derived antibodies have a protective effect by augmenting cell-mediated immunity.
Antibodies can function as opsonins, promoting fungal ingestion and even killing by phagocytes, and several antibodies are directly fungicidal [102–104]. These kinds of activities are complementary, rather than exclusive, to cell-mediated mechanisms. The central importance of granulocytes and macrophages in innate defense against opportunistic fungal pathogens, and of activated neutrophils and macrophages against fungi in general, is consistent with an expectation that opsonic antibodies facilitate host defense. Antifungal antibodies can contribute to the activation of the classic complement system and antibody mediated cytotoxicity by NK cells [105, 106] and can change the gene expression making the fungus more vulnerable to antifungal therapy [3].
Th1-derived antibodies are usually of immunoglobulin G2 (IgG2) isotype, which are excellent opsonins that promote fungal ingestion and even killing by phagocytes. In contrast, the association of antibodies with nonprotective responses or progressive fungal diseases could be due to the possibility that these antibodies are of Th2 isotype (usually IgG1), which are produced by antigen-specific B cells interacting with antigen specific CD4+ Th2 cells producing Th2 cytokines such as IL-4 or IL-10. In that case, Th2-derived antibodies are associated with a Th2 response that causes suppression of protective Th1 and cell-mediated immunity [103, 107].
Cutaneous Fungal Diseases
Cutaneous Candidiasis
Clinical Manifestations and Pathogenesis of Cutaneous Candidiasis
Cutaneous candidiasis is an infection of the skin that is caused by the yeast C. albicans and can be either acute or chronic in nature. Cases of cutaneous candidiasis caused by other Candida species such as C. parapsilosis or C. tropicalis are sometimes seen, but these are rare. Candida albicans is part of the normal flora of the gastrointestinal tract rather than of the skin, although it can be found on the skin [108–110]. This organism can grow as either yeast cells or filamentous forms, with mixtures of the two phases generally seen in tissue infections. Acute cutaneous candidiasis may present as lesions with intense erythema, edema, creamy exudate, and satellite pustules within folds of the skin. Other infections may be more chronic, as in the feet, where there can be a thick, white layer of infected stratum corneum overlying the epidermis of the interdigital spaces. Candida paronychia is marked by infections of the periungual skin and the nail itself, resulting in the typical swelling and redness of this type of candida infection. The virulence of C. albicans has been attributed to various causes, including its ability to grow at particular temperatures, its ability to produce filamentous forms, its adherence capabilities, and the activity of different enzymes. In some cases superficial C. albicans infections may be particularly severe and refractory to treatment, producing the uncommon disorder known as chronic mucocutaneous candidiasis (CMC) [111–113], which consists of persistent and recurrent infections of the mucous membranes, skin, and nails. Oral thrush and Candida vaginitis are fairly common in patients with CMC. There is often infection of the esophagus, although further extension into the viscera is unusual. The typical skin lesions are generally red, raised, and hyperkeratotic but usually are not painful. Epidermal neutrophilic microabscesses, which are common in acute cutaneous candidiasis, are rare in the lesions of CMC. Nail involvement can be severe in this condition, producing marked thickening, distortion, and fragmentation of the nails, with chronic swelling of the distal phalanx.
Immune Responses and Host Defenses Against Candida
The initial events in stimulation of both innate and acquired immune responses against cutaneous Candida infection include recognition of fungal ligands and phagocytosis. The immunoreactive components of C. albicans are thought to be the carbohydrate derivatives found on the yeast surface, including glucans, mannans, and chitin. The activation of TLRs by these fungal products presumably contributes to the inflammatory tissue injury seen in mucocutaneous candidiasis by signaling the release of proinflammatory cytokines and by directing the recruitment and activation of other inflammatory cells [114–119]. TLR2, TLR4, TLR6, and TLR9 play a role in host recognition of C. albicans. C-type lectin-like receptors (dectin-1 and 2) also recognize candida infections by binding to β-glucan or mannose, and patients with dectin-1 deficiency appear more susceptible to mucosal candida infections. When both dectin-1 and TLR2 or TLR4 are activated, there appears to be a synergistic effect on the host’s proinflammatory response [79].
Recognition of mannan by the mannose receptor on macrophages results in the internalization of Candida. Mannan drives TNF-α production by murine alveolar macrophages in vitro and increases the serum TNF-α level upon intravenous administration in vivo. Earlier studies of the immune response against yeasts have relied heavily on the use of zymosan, an insoluble fungal cell wall preparation, which contains large amounts of β-glucan and mannan [17, 21–27, 29]. It was shown that TLR6 and TLR2 formed heterodimers to coordinate the macrophage activation by zymosan [29, 120]. These findings also suggested that immunity against C. albicans requires the collaboration between receptors responsible for phagocytosis (e.g., mannose or complement receptors) and TLRs designed to induce proinflammatory cytokines. Recent studies highlighted the role of Myd88 [12–19, 121, 122], a signaling molecule for TLR in the phagocytosis of C. albicans [19–21]. Macrophages harvested from Myd88–deficient mice and challenged with live C. albicans showed a diminished capacity to engulf and kill the yeast, and a diminished capacity toproduce TNF-α when compared to wild-type cells [21].
In contrast to immune mechanisms involved in protection against widespread, systemic candidiasis, where cells of the innate immune system, particularly neutrophils, are crucial [17, 38], it has long been recognized, based on both clinical and experimental data, that in the mucocutaneous form of candidiasis, cell-mediated immunity is essential for protection. Chronic mucocutaneous candidiasis represents a group of syndromes with a variety of predisposing or secondary abnormalities in host defense function. The most common deficiency appears to be one of cell-mediated immune responses against candida antigens, although abnormalities in chemotaxis or phagocytic cell function have also been reported [111–117]. Other host defense mechanisms, such as humoral immunity and the complement system, have generally been found to be normal in these patients [118]. Although treatment of this condition with amphotericin B could be successful in prompt clearance of the cutaneous lesions, relapses usually occurred, presumably because of the underlying immunodeficiency state. HIV infection or severe combined immune deficiencies affecting cell-mediated immunity predispose to mucocutaneous candidiasis. Evidence of impaired cell-mediated immunity to Candida spp. in these patients is represented by negative delayed-type hypersensitivity skin tests, absent or low T-cell proliferation in vitro, and impaired production of leukocyte/macrophage inhibitory factor [123].
Several human and murine studies suggest that impaired clearance of Candida spp. in patients with CMC is due to imbalance between Th1 and Th2 responses with bias toward the Th2 phenotype. Appropriate and timely induction of Th1 responses characterized by production of Th1 cytokines such as IL-2, IFN-γ, TNF-α [124, 125], and IL-12 [39, 66, 126], which activate and recruit effector cells such as macrophages or CD8+ cytotoxic T cells, are of major importance for protection and clearance of the yeast [123, 124]. In contrast, induction of Th2 responses marked by production of Th2 cytokines such as IL-4 and IL-10, which are necessary for mounting an antibody response but also downregulate type 1 cytokine production, had opposite detrimental effects [125]. Neutrophils are thought to be the primary effector cells and their production of either IL-10 or IL-12 likely directs the immune system toward either a Th1 or Th2 response [126]. Recruitment of neutrophils to the site of candida infection is dependent upon recognition of mannans by TLR4 [127].
Peripheral blood mononuclear cells from patients with CMC produce high levels of IL-10, but normal levels of IL-4 and IL-5, upon in vitro stimulation with carbohydrate and mannan fractions of C. albicans. Although Th1 cytokines such as IL-12 and IFN are also decreased in CMC patients, receptors for IL-12 and IFN are shown to be intact [125]. Two possibilities can account for the bias toward Th2 response in CMC patients. The first possibility is that altered cytokine environment caused by interaction of the yeast or hyphal forms of Candida with innate immune cells could inversely influence the induction of CD4+ Th1 cells producing IFN-γ and IL-2. The second possible mechanism could lie more upstream, involving the co-stimulatory functions of APCs such as DCs or macrophages [30, 128].
In support of the first possibility, recent evidence in mice indicates that innate immune cells such as neutrophils and macrophages discriminate between the hyphal and yeast forms of the fungus through binding to different PRRs such as TLR and lectin, being able to produce IL-12 in response to C. albicans yeasts, and IL-10 in response to C. albicans hyphae [30, 66]. The production of IL-12 and IL-10 can thus bias the CD4+ Th response to Th1 or Th2 phenotype, respectively. Th17 cells have also been recently implicated in maintaining a balanced immune response to CMC, which are thought to control fungal growth in the epithelium. Th17 cells produce IL-12, IL-21, and IL-22, cytokines which play a role in neutrophil recruitment, and therefore appropriate recognition, to the area of candida infection [127].
Interestingly, in vitro culture of DCs with the two forms showed that hyphae escaped the phagosome and were lying free in the cytoplasm of the cells, while the yeast form remained within the phagosome. The differences in fungal localization within DCs, therefore, may influence the access of fungal antigens to MHC class I (cytoplasmic) or II (phagosomal) pathways of antigen presentation to CD8+ and CD4+ T cells, respectively, which influences the ability of each fungal form to stimulate CD4+ or CD8+ T cells. Therefore, DCs can influence the type of specific immune response by discriminating between yeast and hyphal forms of the fungus. This can be accomplished through different TLRs, the downstream effect of differential TLR ligation on the cytokine environment, and differential fungal localization within DC.
Nevertheless, it remains to be identified whether the differential immune responses to different phenotypes of Candida is due to altered responses of immune cells or to expression of different virulent genes that allow evasion of host immune response and persistence. In support of the latter possibility, several studies suggested that phenotypic switching represents a pathogenic strategy for the combinatorial expression of batteries of genes leading to a variety of pathologic states. This conclusion was based on the findings that the process of phenotype switching between yeast and hyphal forms, which is characterized by different colony morphology, regulates the expression of a number of phase-specific genes, including PEP1 (SAP1), SAP3, OP4, CDR3, CDR4, NIK1, WH11, and EFG1 [23, 129, 130]. High-frequency phenotypic switching has also been demonstrated to regulate a number of phenotypic characteristics that have been implicated in pathogenesis, including antigenicity, sensitivity to neutrophils and oxidants, adhesion, and susceptibility to common antifungal agents [130, 131]. Whether similar host-microbial interaction involving putative inherent defects of dendritic cells in terms of different dendritic cell subsets or receptors on the cells or differential gene expression upon phenotype switch exists in CMC patients is not yet known.
Dermatophytosis
Clinical Manifestations and Pathogenesis of Dermatophytosis
The dermatophytes include a group of fungi (ringworm) that under most conditions have the ability to infect and survive only on dead keratin, that is, the top layer of the skin (stratum corneum or keratin layer), the hair, and the nails. Dermatophytes cannot survive on mucosal surfaces such as the mouth or vagina where the keratin layer does not form. Very rarely, dermatophytes undergo deep local invasion and multivisceral dissemination in the immunosuppressed host. Dermatophytes are responsible for the vast majority of skin, nail, and hair fungal infections. These types of infections, termed “dermatophytoses,” are widespread and increasing in prevalence on a global scale [132, 133]. Reasons for this increase are not clear, although it may be due, in part, to an aging process. This observation is consistent with the view that changes in the immune response that occur with advancing age lead to disease susceptibility. In keeping with this, fungal nail infections are more frequent in immunocompromised patients such as those who are HIV positive and those who have diabetes [134, 135]. Alternatively, age-related changes in peripheral vasculature may be important in predisposing to infection. In addition to the effects of aging, genetic susceptibility has been proposed to contribute to infection. Although Trichophyton rubrum infection has been reported to show a familial pattern of autosomal dominant inheritance, more recent epidemiologic findings challenge this view [135]. Other factors that have been implicated include those associated with a modern lifestyle, including the use of footwear made from synthetic materials and exposure to dermatophytes in communal areas with damp environments that favor fungal growth, such as swimming pools and school gymnasiums [135–137]. Despite the identification of multiple predisposing factors, there is no consensus of opinion regarding a single mechanism to explain the increased incidence of foot disease that has occurred in recent years.
Dermatophytes are classified in several ways. The “ringworm” fungi belong to three genera: Microsporum, Trichophyton, and Epidermophyton. There are several species of Microsporum and Trichophyton, and one species of Epidermophyton. The inflammatory response to dermatophytes varies [133–138]. In general, zoophilic and geophilic dermatophytes elicit a brisk inflammatory response on skin and in hair follicles. The inflammatory response to anthropophilic fungi is usually mild. One very characteristic pattern of inflammation is the active border of infection. The highest numbers of hyphae are located in the active border, and this is the best area to obtain a sample for a potassium hydroxide examination. Typically the active border is scaly, red, and slightly elevated. Vesicles appear at the active border when inflammation is intense. This pattern is present in all locations except the palms and soles. Infections of the feet are particularly troublesome and affect both the skin (athlete’s foot) and nails (tinea unguium). Despite the availability of new systemic antifungal therapies, nail infections are difficult to eradicate, with recurrence reported in up to 25 % to 40 % of cases. Since lesions vary in presentation and closely resemble other diseases, laboratory confirmation is often required.
Innate Immune Responses to Dermatophytes
Interaction of dermatophytes with many dermal and epidermal immune cell types including keratinocytes results in a cascade of chemokines and proinflammatory and anti-inflammatory cytokine responses [139–142]. Activation of the alternative complement pathway by dermatophytes such as the case with Trichophyton mentagrophytes also results in production of chemotactic factors. These chemotactic events and inflammatory responses are thus responsible for the observed macroscopic changes associated with dermatophyte infections including scaling, vesicles, pustules, annular dermatitis, and severe inflammatory reactions (kerions). Microscopically, the lesions are characterized by an accumulation of neutrophils in the infected skin of acute infections or a mononuclear cell infiltrate in the dermis of the more chronic ones [139–142]. The acute inflammatory responses may be manifested as epidermal microabscesses, while chronic dermatophyte infection is usually manifested as epidermal hyperkeratosis and parakeratosis [135–139].
Similar to other cutaneous mycosis, neutrophils mediate elimination of dermatophyte infections by a variety of microbicidal processes, including (1) microbicidal oxidants such as superoxide, hydrogen peroxide, hypochlorous acid, and monochloramine; and (2) nonoxidative microbicidal granules such as cathepsins, defensins, lactoferrin, lysozyme, elastase, azurocidin, and a number of other proteins [140]. Furthermore, neutrophils inhibit the growth of dermatophytes through release of large amounts of calcium and zinc-binding protein, called calprotectin that has potent microbiologic static activity against these fungi. This protein is released into inflammatory exudates as neutrophils degranulate at sites of infection [140, 141].
Acquired Immune Responses Against Dermatophytes: Immediate and Delayed-Type Hypersensitivity Reactions
Several of dermatophyte antigens are cross-reactive with airborne molds. These antigens stimulate IgE production, which mediates immediate hypersensitivity (IH) reaction in infected patients, mainly those with chronic dermatophytosis. The IH reaction in these patients is mediated by IgE antibody, and is characterized by a local wheal and flare occurring 5–20 min after injection of antigen into the skin. In this process, binding of antigen to IgE antibody (Ab) on the surface of mast cells results in cross-linking of IgE Ab, which, in turn, triggers the degranulation of mast cells and release of histamine and other proinflammatory mediators [141, 142].
The IH skin tests to Trichophyton are associated with the presence of serum IgE and IgG Ab to Trichophyton antigens. IgG4 Ab is a major component of the IgG Ab response [136]. Thus, IH reactions to dermatophytes bear the hallmarks of a Th2 response. In this type of response, IL-4 produced by CD4+ Th2 cells induces antibody isotype switching to IgG4 and IgE [142, 143]. A role for fungal antigens (Ags) in allergic disease is strongly supported by the association between chronic dermatophytosis and allergic respiratory symptoms, and the improvement of late-onset asthma after antifungal therapy in patients with IH to Trichophyton.
By contrast, Trichophyton antigens are also able to induce delayed-type hypersensitivity (DTH) responses in some patients [133, 143]. Delayed-type hypersensitivity is a form of cell-mediated immunity in which the ultimate effector cell is the activated macrophage. In the classic DTH reaction, activation of macrophages is mediated by IFN-γ–producing Th1 CD4+ T lymphocytes [142–144]. However more recent studies have shown that both CD4+ and CD8+ T cells can have direct cytotoxicity to T. mentagrophytes and T. rubrum, although to a lesser extent [145].
These T cells recognize and respond to foreign antigen presented in the form of peptide complexed with MHC class II molecules expressed on the surface of APCs. This cell-mediated response is characterized by induration at the injection site, which is maximal at 48 h. There is considerable evidence that DTH is pivotal in the eradication of dermatophyte infection. This conclusion is based on data showing (1) the development of DTH in association with inflammatory responses in primary infections, (2) the association between acute highly inflamed lesions and DTH, and (3) the failure to develop infection after experimental inoculation when DTH is present. Interestingly, DTH to Trichophyton extract is associated with lower titers of IgG Ab to Trichophyton antigens and no IgE or IgG4 [142, 144, 146]. These observations suggest that humoral response to Trichophyton is less protective. A subset of patients also mounts “dual” skin test responses in which a DTH response follows the IH reaction. Whether this represents a transitional stage in DTH-to-IH conversion (or vice versa) is not known.
Several hypotheses have been proposed to explain the mechanism of induction or suppression of protective DTH responses in different individuals. One study suggested that development of IH suppresses DTH responses in patients with chronic dermatophytosis since most IH responses occur in the absence of DTH [146, 147]. Treatment of chronic dermatophytosis associated with IH responses with the systemic antifungal terbinafine can restore DTH responsiveness to intradermal trichophyton antigen. Another study proposed that prolonged antigen exposure can induce immunologic unresponsiveness, or anergy, by activating suppressor T cells, which then downregulate cell mediated responses. However, several studies have failed to demonstrate diminished T-cell proliferative responses to dermatophyte antigens in patients with IH [143, 146, 148, 149].
An alternative hypothesis was that the properties of the fungus itself may prevent the development of cell-mediated responses. For example, mannans derived from T. rubrum have been proposed to inhibit DTH by interfering with antigen-processing pathways required for T-cell activation. However, despite all these data, it is not yet clear whether chronic infection results from a lack of DTH or from the presence of an IH response. Thus, a major question is whether IH is a prerequisite for the development of chronic infection and, if so, whether it reflects a more broad-based immune dysregulation. The answer to this question is complicated by the fact that host factors, such as integrity of the skin barrier as well as immune status of the host, play a central role in determining the outcome of dermatophyte infection. Indeed, it is well recognized that patients with HIV infection or those receiving immunosuppressive therapy are predisposed to develop chronic dermatophytosis and sometimes an invasive disease. This observation is consistent with impairment of cell-mediated immunity associated with these conditions.
Changes in the balance between Th1 and Th2 responses with bias toward Th2 response have been implicated in the progression of several diseases such as HIV infection associated with chronic dermatophytosis [146]. Indeed, this would be consistent with the dogma that Th2 polarization contributes to disease progression in HIV-infected subjects. Similarly, chronic T. rubrum infection associated with IH and a markedly elevated total IgE level in the serum was proposed to contribute to the development of severe measles infection by favoring the development of Th2 responses [143, 144, 146, 147]. However, this does not explain the paradoxical association between chronic dermatophytosis and Th1-mediated diseases such as diabetes. Nevertheless, the association between diabetes and dermatophytosis is also complex, as diabetic complications such as peripheral vascular disease could contribute to persistent dermatophyte infection.
Although immune mechanisms involved in the natural resolution of infection have yet to be resolved, recent findings suggest that T cells with a defined specificity for Trichophyton antigens or epitopes are critical for development of DTH or IH responses in different individuals. This conclusion was based on comparison of the T-cell repertoire in subjects with distinct immune responses to a single Ag such as the 29-kd Tri-r-2 derived from T. rubrum. Interestingly, the differences in the T-cell repertoire between patients with IH and those with DTH were independent of human leukocyte antigen (HLA) haplotype [143, 144, 150–152]. The clinical relevance of different T-cell repertoires remains to be determined. However, it is possible that certain antigenic peptides derived from Trichophyton antigens contain an epitope that specifically promotes the development of a DTH response, making progression to chronic dermatophytosis unlikely [150, 151, 153].
Malassezia Infection
Clinical Manifestation and Pathogenesis of Malassezia Infection
Yeasts of the genus Malassezia undergo asexual reproduction by monopolar budding. The yeast cell is actually a phialide that has a small collarette at its apex, which gives it an overall bottle-shaped appearance. Some of the species are able to undergo a phase transition from yeasts to hyphae, although the factors that control this transition are not clearly understood. The genus Malassezia is known to include at least seven species of yeast (M. furfur, M. pachydermatis, M. sympodialis, M. globosa, M. obtusa, M. restricta, and M. slooffiae). Except for M. pachydermatis, the species require an exogenous source of lipid owing to their inability to synthesise C14–C16 saturated fatty acids because of a block in the de novo synthesis of myristic acid [152]. Although Malassezia can be found on normal human skin, it has been implicated in a range of both cutaneous and systemic diseases such as seborrheic dermatitis, dandruff, folliculitis, atopic dermatitis (recently renamed atopic eczema/dermatitis syndrome [AEDS]), psoriasis, confluent reticulate papillomatosis, and seborrheic blepharitis [154–161].
Malassezia is most frequently associated with pityriasis (tinea) versicolor (PV), which is one of the most common disorders of pigmentation seen in dermatologic clinics worldwide [154, 155]. PV is a chronic superficial fungal infection of the skin. The Malassezia species that have been isolated from patients with PV include M. furfur, M. globosa, M. restricta, M. slooffiae, and M. sympodialis, with more than one species of Malassezia being present. PV most often occurs on the trunk, neck, and proximal extremities. It is characterized by scaly hypopigmented or hyperpigmented macules and patches with minimal pruritic reaction [152, 154, 155].
Malassezia folliculitis is characterized by follicular papules and pustules localized to the trunk, upper arms, neck, and, less often, the face [159–161]. These lesions are generally pruritic. Diagnosis is based on clinical signs, cytology, and culture in combination with histopathology. Although it has been suggested that follicular occlusion was the primary cause of Malassezia folliculitis with a secondary overgrowth of Malassezia organisms, colonization of normal pilosebaceous units by these yeasts can also be extensive. The exact role of Malassezia in Malassezia folliculitis, therefore, awaits further elucidation. Therapy for Malassezia folliculitis is similar to that described for PV. As with PV, recurrence tends to be a common problem.
Seborrheic dermatitis and dandruff are other diseases caused by Malassezia. Seborrheic dermatitis is characterized by inflammation and desquamation in areas that are rich in sebaceous glands such as the scalp, face, and upper trunk, whereas dandruff is a noninflammatory scaling condition of the scalp. Dandruff is now generally considered the mildest form or a variant of seborrheic dermatitis [160, 161]. The decreased severity of these diseases upon fungal therapy, the increase in the number of Malassezia organisms, and relapse upon discontinuation of therapy support a role of Malassezia as the etiologic agents in these two conditions. The species that have been isolated from patients with seborrheic dermatitis are similar to those causing PV, with M. furfur and M. globosa being most common. Atopic dermatitis is another chronic, multifactorial, inflammatory allergic skin disease associated with abnormal immunologic regulation and is associated with Malassezia infection [161–163].
Similar to dermatophyte antigens, allergens from Malassezia organisms have been implicated in its pathogenesis [162–164]. For atopic patients with a hypersensitivity response to Malassezia spp., antifungal therapy should be included in the treatment regime. In addition to the diseases described above, Malassezia spp. have also been shown to cause more deep-seated infections, including mastitis, sinusitis, septic arthritis, malignant otitis externa, fungemia, pulmonary vasculitis, peritonitis, and meningitis [159–162].
Immunology of Malassezia-Associated Diseases
There have been large numbers of studies examining the innate and acquired (cellular and humoral) immune responses to Malassezia in patients with many Malassezia-associated diseases [165]. For many of the diseases, the responses reported have varied widely. The innate response against Malassezia involves several components such as complement, phagocytosis, and NK-mediated lysis. Several groups have reported the ability of Malassezia to activate the complement system, via either the alternative pathway or the classic pathway [166–168]. The extent of activation of the alternative pathway was cell concentration and time dependent, reaching a plateau after 30 min. Although the molecule responsible for triggering the alternative pathway is not well defined, ß-glucan in the cell wall may be involved [168]. The ability to activate complement has been suggested as a mechanism responsible for the inflammation associated with seborrheic dermatitis. Phagocytosis and intracellular killing of the yeast, mainly by neutrophils, is another important innate mechanism by which nonspecific effector cells play a role in host defense against Malassezia [169, 170].
The importance of phagocytosis in protection against fungal infections is highlighted by the increased susceptibility of neutropenic patients to many mycoses. In vitro, neutrophils take up Malassezia in a complement-dependent process. On the other hand, the receptors involved in phagocyte–yeast cell binding have been characterized in a human monocytic cell line as the mannose receptor, ß-glucan receptor, and complement receptor type 3 [171]. Recent studies have shown that when a monocytic cell line, THP1, was stimulated with either live or heat-killed Malassezia, the production of IL-8 was increased, while stimulation of a granulocytic cell line, HL-60, resulted in increased levels of both IL-8 and IL-1β [172]. The effects of IL-1β in host defense against cutaneous fungal organisms include the activation of lymphocytes, chemotaxis, and neutrophils and the induction of inflammation [172–174]. Interleukin-8 also induces chemotaxis and activation of neutrophils and T cells. Therefore, the interaction of Malassezia with phagocytic cells may serve to amplify the inflammatory response and encourage further recruitment of phagocytic cells.
Opsonized live Malassezia yeast cells are more stimulatory than were nonopsonized or heat-killed Malassezia yeast cells. However, the ability of neutrophils to kill Malassezia seems limited compared to efficient killing of C. albicans yeast cells and other fungal genera. The mechanism by which Malassezia may resist or prevent phagocytic killing is not completely clear. In addition to escaping phagolysosomal killing, in vitro studies suggested an immunosuppressive effect of Malassezia on activation of different innate effector cells [174, 175]. M. furfur was able to invade human keratinocytes and resist phagolysosomal fusion, which allows their survival inside host cells. In addition, culture of yeast cells of M. furfur with normal human keratinocytes did not stimulate cytokines or chemokines production.
Low levels of monocyte chemotactic protein 1 (MCP-1), TNF-α, and IL-1β (IL-1β) were found, which were associated with overproduction of immunosuppressive cytokines IL-10 and tumor growth factor-β (TGF-β) [175]. It was further postulated that the suppression of proinflammatory cytokines might allow Malassezia to survive within host cells without causing an inflammatory response. Suppression of IL-1β, IL-6, and TNF-α also has been reported when Malassezia was co-cultured with peripheral blood mononuclear cells (PBMCs), and that the suppression was IL-10 dependent [175]. This correlates with the situation seen in normal healthy skin and also with the limited inflammation seen in PV, despite the large fungal burden seen in the lesions [176, 177]. It is known that the immunosuppressive effects of Malassezia on PBMCs can be reversed by removal of the lipid-rich capsular-like layer around the organism, and it will be interesting to determine if this is also the case with keratinocytes [178].
The role of DC and NK cells in innate and acquired immune responses against Malassezia in atopic and nonatopic individuals has been extensively studied. Uptake of whole M. furfur yeast cells and various allergenic components from the yeast, including M. furfur extracts, recombinant M. furfur allergen 5 (Mal-f-5), and M. furfur mannan by immature monocyte-derived dendritic cells (MDDCs) has been demonstrated in vitro [179, 180].
The internalization of Malassezia was shown to occur via binding to the mannose receptor (other receptors may also be involved) or pinocytosis and is not influenced by IgE. The presence of M. furfur was also shown to induce maturation of immature MDDCs by upregulation of CD83 expression, and also resulted in increased expression of the co-stimulatory molecules CD80 and CD86 [179–181].
The uptake of the yeast by the DCs also induced a significant production of TNF-α, IL-1β, and IL-18, but not IL-10 or IL-12, after 46 h of co-culture. Although immature DCs are highly phagocytic, mature DCs are excellent at presenting antigen-derived peptides on MHC molecules to T cells [182]. Thus, the DCs that had been exposed to Malassezia induced proliferation of autologous T lymphocytes in a dose-dependent way [182–184].
The interaction of Malassezia-infected mature DCs with NK cells in atopic patients also has been examined by comparing the numbers of NK cells in the normal skin of healthy controls with those in the atopic skin of atopic dermatitis or AEDS patients [179, 185]. These studies showed that there were only scanty NK cells in normal skin, but that they were numerous in the atopic skin, and they were in close apposition with DCs. Dendritic cells that had been preincubated with Malassezia for 46 h were less susceptible to NK-mediated lysis, and this resistance to NK lysis was mediated by soluble factors [185–189].
This protection of DCs against NK-mediated lysis, if it were to occur in vivo, would allow the mature dendritic cells to remain in the epidermis, presenting Malassezia antigens to T cells and hence contributing to the maintenance of the inflammatory response in AEDS lesions [188, 189].
The effect of different forms of Malassezia on the responses of DCs has been examined. Similar to that described in immunity against C. albicans, the yeast phase elicits production of IL-12 and priming of Th1 cells, while the hyphal phase inhibits IL-12 and Th1 priming, and induces production of IL-4, a Th2-type cytokine [190–192]. Similar to other fungi, Th1 T-cell–mediated immunity is important in the prevention and recovery from infections. A deficiency in cell-mediated Th1 responses could therefore predispose the host to overgrowth of Malassezia. Atopic patients with specific IgE antibodies against M. furfur were shown to have increased synthesis of the Th2-related cytokines IL-4, IL-5, and IL-10 by Malassezia-stimulated PBMCs [190–192].
In regard to humoral immune responses against Malassezia, it is clear that IgG responses to Malassezia yeasts are common in both healthy individuals and patients with Malassezia-associated diseases. This probably reflects exposure of the immune system to antigens produced by commensal organisms [193]. However, enhanced IgG responses can be seen in humans with atopic dermatitis. The role of this IgG response in the pathogenesis of skin disease is currently unclear. IgG antibodies are known to be able to act as opsonins coating microorganisms and to activate phagocytes, which in turn ingest and destroy extracellular pathogens. This could in theory provide protection for the host. However, as overgrowth with Malassezia does not appear to be a self-resolving condition, it seems likely that these antibodies are not protective [194, 195].
Alternatively, IgG antibodies could activate the complement system, as has been demonstrated with Pityrosporum ovale and P. orbiculare, and exacerbate the inflammatory response [196]. A final possibility is that IgG responses to the yeast are merely an association and neither contributes to nor inhibits the ongoing disease process [195]. Further studies are therefore required to determine the precise role played by these antibodies in Malassezia-induced skin disease. Using in vitro serologic tests such as enzyme-linked immunosorbent assay (ELISA), the radioallergosorbent test (RAST), and Western immunoblotting, Malassezia-specific IgE has been detected in human atopic patients for over a decade [194, 195].
Stimulation with Malassezia extracts and IL-4 led to a dose-dependent increase in IgE synthesis from PBMCs only in RAST(+) atopic patients, indicating a Th2-type skewed response towards Malassezia in these patients. The Malassezia-specific IgE antibodies in human atopic patients could play a key role in enhancement of immune responses [196–198]. The allergen-specific IgE antibodies could bind to Langerhans cells in the skin, thus enhancing their allergen capturing and presentation capacity upon a second encounter with the allergen. In addition, IgE could mediate mast cell-mediated hypersensitivity responses to Malassezia allergens, and that may be involved in the pathogenesis, and contribute to the clinical signs, in some cases of human atopic dermatitis.
Subcutaneous Mycosis
Chromoblastomycosis
Clinical Manifestations and Pathogenesis of Chromoblastomycosis
Chromoblastomycosis is a term that designates a group of chronic cutaneous and subcutaneous mycoses caused by several species of dematiaceous (darkly pigmented) fungi. As a member of the heterogeneous group of subcutaneous mycoses, chromoblastomycosis commonly presents the following typical features: lesions beginning at the site of a transcutaneous trauma, chronic evolution associated with survival of the fungal agent, and fibrotic reaction [199–201] as seen in Fig. 19.4. In tissues, all agents form thick-walled, dark multiseptate structures—the muriform (sclerotic) cells. It is common worldwide but occurs mostly in tropical and subtropical areas of Africa, Asia, and South America. Chromoblastomycosis is considered an occupational disease in many tropical and temperate countries (Madagascar, northern Venezuela, and the Amazon region of Brazil) [202–204].


Fig. 19.4
Leg of a patient with chromoblastomycosis
The disease is caused by a large number of fungi that exist in the soil, plants, flowers, and wood. The most common agents are Fonsecaea pedrosoi and Cladophialophora carrionii. The latter is considered to be the most important etiologic agent in deserts in South Africa and Australia [202–204]. Less frequently, the disease is caused by Phialophora verrucosa, Rhinocladiella aquaspersa, as well as Wangiella or Exophlaia dermatitidis. Other etiologic agents that were discovered recently are Exophiala jeanselmei and Exophiala spinifera. Fonsecaea compacta has also been observed in lesions of chromoblastomycosis. The etiologic agents enter the host through cutaneous puncture wounds, usually on a thorn or a splinter. Other kinds of trauma such as animal-associated trauma (insect bite or sting) are identified as the portal of entry of the fungus.
A primary lesion is represented by a papule that slowly enlarges over time and can ulcerate. The primary lesion can progress to polymorphic skin lesions, including nodular and verrucous lesions. Sometimes the lesions can heal as sclerotic plaques, scars, or keloid formations [199–204]. The most frequent clinical presentation is a cauliflower-like lesion that develops at the site of inoculation, and satellite lesions gradually arise from scratching, autoinoculation and spread via the lymphatic system. Secondary lymphedema and pruritus are common findings, but the disease remains confined to the subcutaneous tissue and does not invade underlying muscles and bone, except in immunosuppressed patients such as those on high-dose corticosteroids. The lower limb is the most common site followed by the upper limb, ear pinna, and nose [199–204]. The diagnosis can be made by detection of multiform cells, referred to as sclerotic bodies, from tissue biopsies or from fungal culture. Hydrogen peroxide and hematoxylin and eosin (H&E) are recommended stains for elucidation of the sclerotic bodies in tissues [204, 205]. The hyphae and budding cells are usually seen in the surface of the lesion, while the muriform cells are often seen in the deep part of the lesion. The treatment of chromoblastomycosis is difficult due to its limited response to oral antifungal therapy [206, 207]. Medical treatment usually includes combined use of antifungal drugs such as itraconazole with terbinafine. Other treatment strategies include a combination of cryotherapy, 5-fluorocytosine, and amphotericin B. Surgical treatment is the ultimate effective therapy, which involves surgical removal of lesions, electrodesiccation, and cryosurgery.
Immunology of Chromoblastomycosis
The host defense mechanism in chromoblastomycosis has not been extensively investigated. The first line of defense utilizes HLA-DR and costimulatory molecules, like CD86, TNF-α, IL-10, and IL-12, to activate dendritic cells. Although melanin produced by F. pedrosoi has been suggested to play a role in immune evasion, other research suggests that melanin stimulates the immune response by helping to activate TLR4 leading to the production of IL-8, increasing fungal internalization and amplifying oxidative burst. Mannose in the cell wall may also contribute to this immune reaction [208].
Some studies have focused on fungus–host interactions, showing a predominantly cell-mediated immune response, with activated macrophages involved in fungus phagocytosis [209–211]. The highly organized inflammatory reaction and chronic nature of chromoblastomycosis is characterized by the presence of a granulomatous reaction in association with neutrophil-rich, purulent abscesses. This granulomatous reaction shows extensive phagocytosis of brown thick-wall fungal cells, which is considered the main factor explaining the chronic and inflammatory nature of the disease [204, 212]. However, the degree of phagocytosis and cytotoxicity depends on the fungal species causing infection. P. verrucosa and R. aquaspersa rely on complement-mediated phagocytosis and macrophages are only cytotoxic on R. aquaspersa [208].
Macrophages (referred to as epithelioid or giant cells) are highly activated as marked by higher expression of TNF-α and their enhanced in vitro antifungal activities marked by H2O2 and NO production [213, 214]. Inhibition of macrophage synthesis of NO by fungus-derived melanin has been proposed as an immune evasion mechanism that prevents the host from clearing F. pedrosoi, leading to a chronic disease [215]. CD4+ Th1 cells and type 1 response are important for host defense against causative agents of chromoblastomycosis [216]. Patients with a mild form of the disease have predominant production of IFN-γ, low levels of IL-10, and efficient T-cell proliferation, consistent with a type 1 response, while patients with the severe form of the disease have predominant production of IL-10, low levels of IFN-γ, and inefficient T-cell proliferation, consistent with an immunosuppression rather than Th2 response [216, 217] Histopathologic and immunohistochemical staining of skin tissues from patients with the verrucous form of the disease has identified CD4+ T lymphocytes at the periphery of the granulomas, with immunostaining for IL-10. These findings suggest that either a Th2 phenotype or immunosuppression is linked to severe or verrucous forms.
Furthermore, patients with chromoblastomycosis produce specific IgM, IgG, and IgA antibodies [209] and levels may correlate with disease chronicity [208]. Several cell wall and secreted immunoreactive antigens of F. pedrosoi have been identified. Antifungal antibodies specific to secreted melanin inhibit fungal development, support fungal internalization, and enhance macrophage functions as marked by greater degrees of oxidative burst [52]. Sera from infected human patients also reacted with secreted melanins, suggesting that F. pedrosoi synthesizes melanin in vivo [52]. Antibodies against melanin purified from patients’ sera also reacted with sclerotic cells from patients’ lesions as well as with sclerotic bodies cultivated in vitro—conidia, mycelium, and digested residues [218].
Taken together, these results indicate that melanin from F. pedrosoi is an immunologically active fungal structure that activates humoral and cellular responses that could help the control of chromoblastomycosis. Glucosylceramide (GlcCer) is another immunoreactive conserved lipid component in the cell wall of many fungi including dark fungi. Antifungal antibodies specific to GalCer directly inhibit fungal development, which supported the use of monoclonal antibodies to GlcCer as potential tools in antifungal immunotherapy [219, 220]. However, unlike the clear correlation between mild disease and cell- mediated type 1 response, there is inconclusive evidence to support a protective role of the humoral immune response in host defense against chromoblastomycosis.
Mycetoma
Clinical Manifestation and Pathogenesis of Mycetoma Infection
Mycetoma is a chronic granulomatous infection caused by fungi (eumycetoma) or actinomycetes (actinomycetoma). The first description of a case of mycetoma is usually attributed to Dr. John Gill, who reported “Madura foot” in a report of the Madras Medical Service of the British Army in India in 1842 [221–224]. The mycetoma lesion is characterized by a subcutaneous mass and multiple sinuses draining pus, blood, and fungal grains as seen in Fig. 19.5. The morphologic characteristics and color of the grains provide clues about the species of the agents. The mycetoma infection has a prolonged and indolent course. The mycetoma lesion might ultimately extend to deeper tissues and bones, leading to deformity of the affected site and subsequent disability for the patient. This disease has been reported in many countries including Sudan, Somalia, Senegal, Mauritania, Kenya, Niger, Nigeria, Ethiopia, Chad, Cameroon, Djibouti, India, Yemen, Mexico, Venezuela, Columbia, and Argentina [221–225].


Fig. 19.5
Clinical example of Madura foot from mycetoma infection
Mycetoma infection is not self-limited and, if untreated, leads to massive lesions, which in the end necessitate surgical amputation. Mycetoma initially presents as a slowly progressive and painless subcutaneous swelling, sometimes in combination with a history of preceding trauma [225–227]. However, the incubation time before classic signs develop is not well defined because in many cases the patients usually present at later stages of disease when most early clinical symptoms have disappeared.
The duration of the disease, the type of causative organism, the site of the infection, and the immune response of the host can all affect the clinical presentation of mycetoma. However, patients with a short disease history might present with massive lesions and severe destruction of deep tissues and even of bones. The subcutaneous swelling is usually firm and rounded but it can also be soft and lobular. It is rarely cystic and is often movable. The subcutaneous nodule increases in size, and secondary nodules might evolve as well. The nodules might suppurate and drain through multiple sinus tracts, which can close transiently after discharge during the active phase of the disease. The disease process involves opening of fresh adjacent sinuses while the old ones heal completely. The nodules are connected to the skin surface and to each other through deep sterile abscesses.
Mycetoma can affect any part of the body. Most cases are usually seen in the feet, followed by the hands, legs, and knee joints. Rarely, the chest and abdominal walls, facial bones, paranasal sinuses, eyelid, orbit, scrotum, and old surgical incisions might also be affected [224–228]. Mycetoma spreads locally or through the lymphatic system, and, rarely, through the bloodstream. Compared to actinomycetoma where secondary nodules that represent lymphatic metastasis, are common, eumycetoma is rarely associated with secondary nodules [229].
Mycetoma is usually painless, and the mycetoma lesion has been suggested to produce substances that have an anesthetic effect. At a late stage of the disease, the absence of pain might be due to nerve damage by the tense fibrous tissue reaction or endarteritis obliterans, or alternatively, poor vascularization of the nerves. In the final stages of the disease, pain might be due to invasion of the bone or to secondary bacterial infection. As the mycetoma granuloma increases in size, the skin may become smooth and shiny, and areas of hypopigmentation or hyperpigmentation can develop [226–230].
Diagnosis of mycetoma is based on the presence of subcutaneous masses, sinuses, and granular discharge in patients from an endemic area. Madurella species, the causative agents for eumycetoma, in general are slow-growing fungi that produce dark colonies composed of a dense, melanized, and mostly sterile mycelium. Madurella species are well-known agents of black-grain mycetoma. Two species are recognized, M. mycetomatis and M. grisea.
In different culture media, M. mycetomatis strains show various colonies and moderate growth rate. The colonies, which are white and woolly at first, becoming olivaceous, yellow, or brown, and produce a brownish, diffusing pigment. Colonies are mostly sterile, composed of dense melanized mycelium. No efficient sporulation has ever been seen [231, 232].
However, phialides with minute conidia in balls and collarettes may be seen. Species-differentiation of M. mycetomatis and M. grisea can be made by differences in sugar assimilation and optimal growth temperature. M. mycetomatis assimilates lactose but not sucrose, whereas M. grisea assimilates sucrose but not lactose. M. mycetomatis grows well at 37 °C, while M. grisea does not grow at 37 °C (growth is seen at 30 °C). This finding might also explain the observed difference in virulence.
Molecular tests have recently been developed and have significantly improved the diagnosis. The polymerase chain reaction (PCR) test is useful for detection and identification of M. mycetomatis in patients [233]. The test showed that a wide range of agents can actually cause eumycetoma. When different isolates of M. mycetomatis derived from patients originating from endemic areas were genetically compared, they did not show major differences, despite differences in phenotypes seen when cultured [231–233]. The diverse clinical presentations thus seem to be due to differences in host susceptibility rather than gross genetic differences among the fungal strains involved. In addition to culture, biochemical, and molecular identification of the fungal etiologic agents of mycetoma, other diagnostic tests are important in developing an appropriate plan of treatment [234–236]. Magnetic resonance imaging has been shown to be valuable in the detection and even identification of fungal grains and for the assessment of therapeutic success [236]. Furthermore, radiology helps in clinical diagnostics, especially in the follow-up of disease progression, development of a surgical strategy, and assessing the clinical cure. At the level of the fungus, direct examination of grains might be useful in determining the type of mycetoma [234, 235]. The large numbers of causative organisms and the poor in-vitro differentiation of fungi that cause mycetoma complicate the identification process. Histopathologic examination is generally not useful for differentiation of fungi, although some of the pigmented fungi may be categorized to a certain extent [237]. Fine-needle aspiration cytologic methods for mycetoma have also been described and are considered to be useful. Finally, serodiagnosis also can be helpful in identification of the etiologic agent [238–242]. The common serologic tests are immunodiffusion and ELISA; however, due to possible cross-reactivity between some species, the specificity of these assays is compromised [229, 238–242].
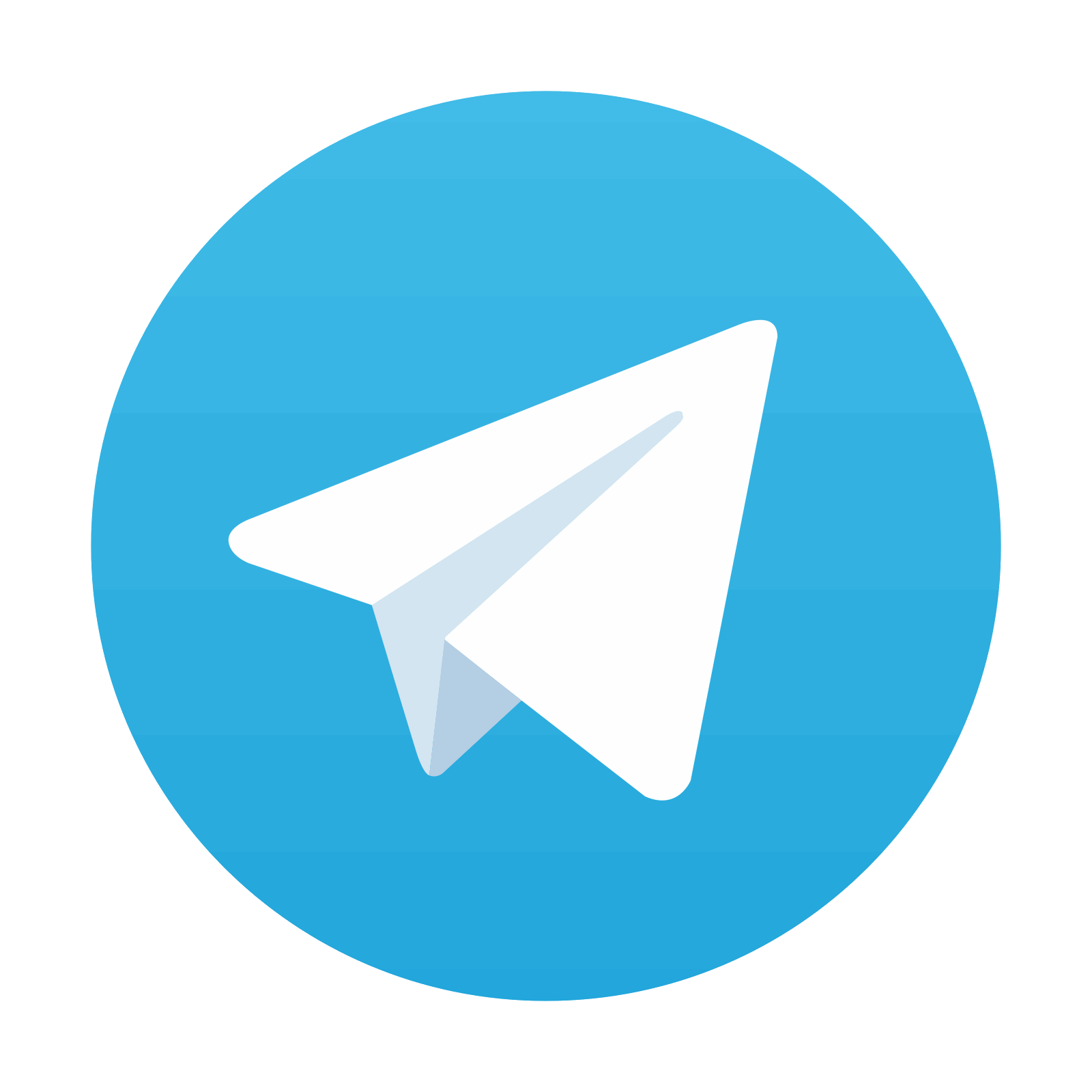
Stay updated, free articles. Join our Telegram channel

Full access? Get Clinical Tree
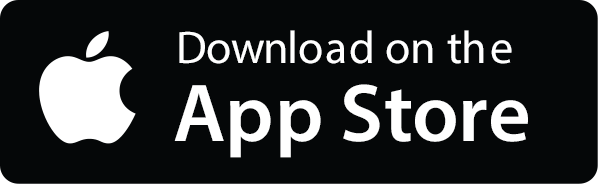
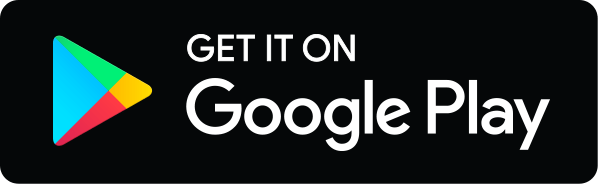