Early Clinical Experience with the 1440-nm Wavelength Internal Pulsed Laser in Facial Rejuvenation
Two-Year Follow-up
Keywords
• Internal pulsed laser • Facial rejuvenation • Body rejuvenation • Collagen • Lipolysis • Noninvasive cosmetic surgery
Introduction
The recent adoption of the internal 1440-nm pulsed laser has advanced the safety, efficacy, and versatility of laser lipolysis and tissue tightening in face and body rejuvenation.1–6 The longer wavelength provides increased localized photothermal and vaporizing effects in front of the fiber on fatty tissue and collagen fibers (water), achieving 20 times more absorption in adipose tissue than the 1064-nm/1320-nm and 40 times more absorption than 924-nm/980-nm wavelengths.7–11 In recent clinical studies,4,6 use of the 1440-nm wavelength has been shown to significantly increase skin thickness and elasticity 6 to 18 months from baseline measurements. This article reports the early experience with the 1440-nm wavelength, using a specially designed side-firing fiber in a four-step approach primarily to the lower third of the midface and neck.
Laser device system
The laser workstation delivered the 1440-nm wavelength pulsed laser from a 800-μm side-firing fiber that was enclosed and protruded 2 mm from the tip of a temperature-sensing 1.2-mm cannula. The side-firing fiber distributed about half of its energy perpendicular to the fiber axis and the other half transmitted along the fiber axis (Fig. 1). Chevron markings on the handpiece designated the direction of the laser emission perpendicular to the fiber axis. This emission design permitted a more targeted delivery of laser energy to the structures of interest, which included in turn fat, fibrofascial layer of the muscle, and the skin-dermis. During treatment of the fatty tissue and deeper fibrofascial muscle layer, the temperature-sensing cannula acted as a thermal switch by sensing temperatures set between 45°C and 47°C at the immediate laser-delivery point. When the recommended target temperature was attained, an audible signal was triggered. If the surrounding tissue temperatures exceeded the preset threshold level, a beeping audible signal warned the operator to move to another area to reduce excessive thermal injury. Maintenance of this thermal window distributed laser heat energy evenly to melt fatty tissue and denature collagen in a safe manner. When the laser fiber treated shallow subdermal tissue (5 mm below the dermis), the temperature-sensing cannula was programmed at the same 45°C to 47°C temperature range, which in turn produced a superficial skin temperature between 38°C and 42°C, which was optimal for collagen denaturation and later delayed tissue tightening. An infrared thermal camera (Therma View EHS, FLIR Systems Inc., Niceville, FL) obtained continuous skin temperatures between 38°C and 42°C and ensured a uniform, real-time deliverance of heat by depiction of a confluent orange-red coloration within each treatment site. A hand-held infrared noncontact thermometer (MiniTemp MT6; Raytek Corporation, Santa Cruz, CA) was used simultaneously with the thermal camera to measure rapidly surface skin temperatures by spot-checking to ensure skin safety.
Procedure
In the first step, the fiber was inserted through one of the access incisions in the down position (Fig. 3). Once in place, the activated cannula-fiber was moved back and forth in a fanlike pattern in the mid-to-deep levels of the subcutaneous fat in each sector. Two sectors on one-half of the neck were treated at a time to optimize laser delivery. The power and pulse frequency settings were set between 6 and 10 W and 25 H, respectively. The endpoints of treatment were determined when the total number of joules delivered ranged between 500 and 700 J/sector, and limited or no tissue resistance to the fiber’s passes while the temperature-sensitive cannula maintained a 45°C to 47°C threshold. External skin temperature monitoring was performed with the thermal infrared imaging camera and the hand-held infrared noncontact thermometer.
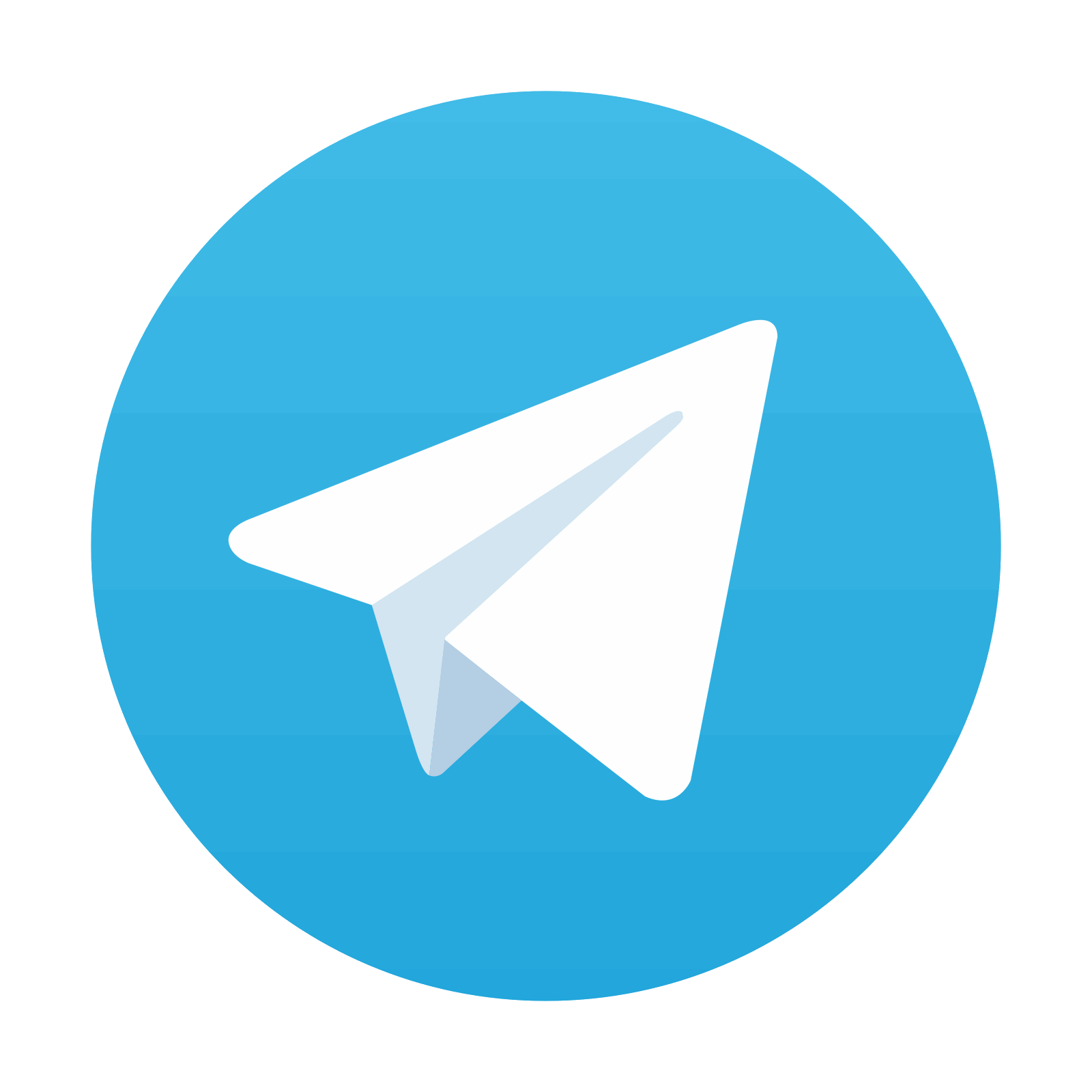
Stay updated, free articles. Join our Telegram channel

Full access? Get Clinical Tree
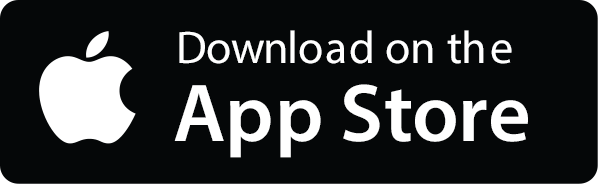
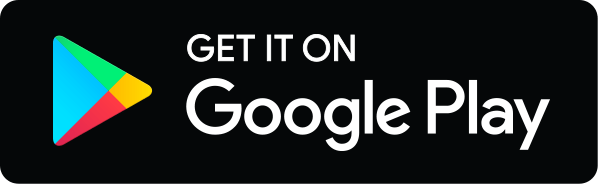