Fig. 7.1
Antibody structure and immunoglobulin locus organization. (a) Structure of an individual antibody molecule. Highlighted are the immunoglobulin light chains (IgL, blue) and heavy chains (IgH, black), the disulfide hinge (yellow), two identical antigen binding variable regions (Fab, pink) and the crystalline region (Fc, green). Cleavage sites for the generation of Fab, F(ab′)2 and Fc fragments, with papain and pepsin, respectively, are indicated. (b) The B cell receptor (BCR) signaling complex is comprised of membrane anchored immunoglobulin and a CD79A/B (Ig α/β) heterodimer. Following antigen (Ag) binding to the antigen binding variable region of the BCR, signaling is triggered via the CD79A/B immunoreceptor tyrosine-based activation motifs (ITAMs). (c) Key components of the human germline IgH locus and IgL (both k and λ) loci [11–13]. Numbers of estimated functional gene segments are indicated in parentheses following gene segment type. IgH constant (C) genes encode constant regions and dictate isotype. μ = IgM, δ = IgD, γ = IgG (IgG1, IgG2, IgG3, IgG4), α = IgA (IgA1, IgA2) and ε = IgE. (d) Antigenic (Ag) stimulation initiates class-switch recombination (CSR). Mature, antigenically-naïve B cells express both IgM and IgD; following Ag stimulation and class switch, they express IgG, IgA or IgE. (e) Secreted antibodies can exist in three forms: monomeric, dimeric (IgA only) or pentameric (IgM only) that have implications for avidity and for downstream antibody-mediated effector function
As antibodies can be raised against a wide range of antigens, they are valuable reagents in clinical and research applications. Antibody molecules can be conjugated to flurochromes, enzymes, toxins and chemical isotopes or used directly (ustekinumab, adalimumab, belimumab) to target specific antigens for the treatment of malignancies or autoimmune diseases or for flow cytometric or histologic analysis. To develop reagents that lack background binding from the Fc region, it is often useful to separate antigen-binding domains from effector domains using proteases that cleave the disulfide bonds between Fab and Fc fragments. Digestion with papain yields two identical, but separated Fab fragments (each with a single antigen-binding domain) and digestion with pepsin yields a single F(ab’)2 fragment with two antigen-binding domains (Fig. 7.1a). These fragments can also be labeled with fluorochromes and enzymes, etc., as described above. Using genetic approaches, the DNA encoding the variable region can be ligated to alternate Fc regions to create chimeric human-mouse monoclonal antibodies (infliximab, rituximab), which are less immunogenic than complete mouse antibodies, or antibodies with enhanced Fc-mediated functions (obinutuzumab, omalizumab). Additionally, Fc regions can be linked to receptors in order to target ligands of interest (etanercept, abatacept).
Diversity in the Antigen-Binding Variable Region Is Generated via Gene Segment Recombination and Use of Editing Enzymes
The enormous diversity in BCR specificity is generated via several mechanisms. Prior to antigen exposure, diversity in the BCR repertoire results from the recombination of a seemingly limited number of gene segments encoding the heavy (IgH) and light (IgL) variable regions and from variations in the junctional regions between these segments (Fig. 7.1c). This process is analogous to the generation of the TCR repertoire. The IgH locus, located on human chromosome 14, is comprised of three gene segments – Variable (V), Diversity (D) and Joining (J). IgL loci, found on chromosomes 2 (kappa) and 22 (lambda), contain only V and J gene segments. The IgH locus contains an estimated 37 VH gene segments, 23 DH gene segments and 6 JH gene segments [11–13]. There are also approximately 35 Vk gene segments, 5 Jk gene segments, 35 Vλ gene segments and 4 Jλ gene segments [12]. A rearranged IgH will contain a single V, D and J gene segment while a rearranged IgL will contain a single V and J gene segment from either the kappa or lambda locus. Thus, combinatorial diversity yields roughly 106 possible IgH and IgL pairings [12].
Recombination of IgH and IgL segments proceeds in an orderly fashion mediated by the V(D)J recombinase enzyme complex, which includes two proteins encoded by genes uniquely expressed in B cells and T cells: recombination activating gene-1 (RAG-1) and recombination activating gene-2 (RAG-2). Recombinases bind signaling sequences flanking the gene segments and then bind to each other to bring the segments together for joining. Mice deficient in the RAG proteins lack mature B cells and T cells and thus have no viable adaptive immune system. Some humans with severe combined immunodeficiency (SCID) have mutations in one or both of the RAG genes, resulting in little to no V(D)J recombination [14]. Additionally junctional diversity/generates more sequences than would be present through mere recombination of the germline genes. Junctional diversity is introduced by exonuclease, which removes nucleotides at the sites of V(D)J recombination, and terminal deoxynucleotidyl transferase (TdT), which catalyzes the addition of random nucleotides.
While the above mechanics generate diversity in the pre-immune antibody repertoire, further diversification in the BCR occurs after antigen exposure and can result in the formation of B cell clones that bind pathogens and immunogens with a much higher affinity than the original clone. This process of somatic hypermutation occurs in the germinal center reaction as a result of T-dependent B cell activation. It is catalyzed by activation-dependent (cytokine) deaminase (AID) and results in the selection of point mutations focused in hotspot hypervariable regions important for binding antigen.
The Heavy Chain Constant Region Dictates Antibody Isotype and Effector Function
The effector function of an antibody is distinct from its antigen binding specificity and is dictated by the class of its heavy chain. There are five antibody isotypes – IgM, IgD, IgA, IgG and IgE – that are each encoded by a distinct constant region (C) gene. The C gene cluster follows the J gene segments on the IgH locus. All mature naïve B cells co-express transmembrane IgM and transmembrane IgD of identical specificities. Other isotypes are expressed only when the B cell is activated by antigen, after an irreversible process known as class-switch recombination (CSR) (Fig. 7.1d). Class-switch recombination does not affect the rearranged V(D)J nor does it use the RAG enzymes. Therefore, a single variable region can elicit a number of different effector functions depending on the C gene with which it is associated.
Functional properties of the various isotypes are summarized in Table 7.1. Secreted IgM is unique amongst the isotypes in that it can pentamerize, which is advantageous in binding repetitive antigen sites, such as those found on encapsulated bacteria and gut flora, and in increasing antibody avidity (binding strength) (Fig. 7.1e). IgD, initially expressed by all naïve B cells, is poorly defined in effector function. IgG is the most abundant isotype in the serum and also has the longest half-life. In humans, there are four subclasses of IgG – IgG1 (the most abundant IgG subclass), IgG2, IgG3, and IgG4. Maternal IgG can cross the placenta, providing protection to the fetus. IgA is produced primarily at mucosal surfaces such as the respiratory and gastrointestinal tracts and, when secreted, can dimerize and cross the epithelium (Fig. 7.1e). Finally, IgE plays an important role in immunity to parasites, in type I hypersensitivity and in common allergic responses. It is unique amongst antibody isotypes in that it can be bound to its high affinity Fc receptor prior to antigen binding.
Table 7.1
Isotype-specific functions of antibodies
Isotype | Complement fixation | Major functions and unique properties |
---|---|---|
IgM | +++ | Antigen receptor of naïve B cells |
Pentameric secreted IgM | ||
Fcα/μR binding | ||
IgD | No | Antigen receptor of naïve B cells |
IgG1, IgG3 | ++/+++ | FcγR binding – all |
IgG2 | + | FcγR binding – all except FcγRI, FcγRIIB and FcγRIIIB |
IgG4 | No | FcγR binding – all except FcγRIIIB |
IgA1, IgA2 | No (Lectin but not classical pathway) | Mucosal immunity |
FcαR, Fcα/μR binding | ||
IgE | No | Helminth response |
Allergic response | ||
FcεR1, FcεRII binding |
Class-switch is heavily influenced by the cytokine milieu surrounding the B cell. Cytokines may be produced by CD4+ T cells, macrophages or dendritic cells and can promote, inhibit or augment class-switch to specific isotypes. For example, in mice, IL-4 promotes class-switch to IgG1 and IgE but blocks class-switch to IgG2a and IgG3. Conversely, IFN-γ induces class-switch to IgG3 and IgG2a, but blocks class-switch to IgG1 and IgE. Along with IL-5, IL-6 and TGF-β, IL-4 and IFN-γ play important roles in influencing B cell effector function.
Effector Functions of Antibodies
Neutralization
Some antibodies function by binding to and directly neutralizing their antigens, a role that does not require the help of other immune cells or immune cell products. Neutralizing antibodies are effective against bacterial toxins, viruses and adhesion factors expressed on bacteria that facilitate host entry. Thus, B cells (through antibodies) are capable of blocking infection before it occurs. Neutralizing antibodies are central to the protective effects of the tetanus toxoid, pneumococcal, hepatitis A and B and HPV vaccines. Development of effective vaccination strategies to induce neutralizing antibodies against human immunodeficiency virus (HIV) and influenza virus have been more challenging, in large part because these viruses have intrinsic mechanisms to mutate or mask vulnerable surface epitopes.
Complement Activation
Most antibodies do not neutralize their targets, but instead initiate a protective response by recruiting other components of the immune system, such as the innate complement system. The complement system is a collection of circulating and cell membrane proteins that play critical roles in host defense against microbes and in tissue injury. The complement system can be activated by pathogens directly or by antibodies, thus linking the adaptive and innate immune systems. Ability to activate the complement pathway varies widely amongst antibody isotypes; IgM and most IgG isotypes are robust at activating the complement cascade while IgE and IgA are not (Table 7.1).
The complement pathway is a series of controlled proteolytic cleavage reactions that ultimately lead to microbe or host cell destruction, neutrophil recruitment and inflammation. There are three complement pathways – classical, mannan-binding lectin and alternative – which all converge on a common intermediate protease, C3 convertase. This convertase is in turn cleaved with its products eliciting various effector functions.
Activation of the classical pathway is initiated by binding of the first complement component, C1q, to the Fc portion of an antibody:antigen complex, known as an immune complex. This binding triggers a series of proteolytic reactions, leading to C3 cleavage and activation. Activated C3b fragments can opsonize (coat) microbes and these fragments in turn bind to specific complement receptors on phagocytes, ultimately leading to microbe phagocytosis. When binding to microbes such as Neisseria, C3b fragments initiate formation of the membrane-attack complex, which creates pores in the pathogen membrane, leading to its lysis. Lastly, C3 activation and subsequent reactions stimulates release of inflammatory mediators, enhances vascular permeability and triggers neutrophil recruitment and activation.
There are six complement receptors (CR) that vary in cell expression and in effector function. Red blood cells (RBCs) express the most CRs, binding immune complexes for later removal in the spleen. CRs can be found on macrophages, endothelial cells, mast cells, dendritic cells, FDCs and even B cells themselves.
Complement activation can stimulate and enhance the humoral response via binding of B cell-expressed CR1/CD35 and CR2/CD21. CR2 functions as a BCR auxiliary receptor, as recognition of C3 coated pathogens by the BCR and CR2 simultaneously triggers a stronger net signal than BCR alone. Additionally, complement receptors on FDCs bind antigens coated with complement proteins, presenting them to B cells in the germinal center reaction, thus facilitating B cell activation and selection during the immune response.
The complement system is tightly regulated and dysregulation of or deficiencies within the complement cascade can lead to poor pathogen clearance and immune complex-driven disease. Complement opsonization is a primary clearance mechanism for polysaccharide encapsulated bacteria, so individuals with complement deficiencies can have serious Hemophilus, Streptococcal and Neisseria infections [15]. Binding of antigen:antibody immune complexes to complement receptors on red blood cells is critical to their clearance in the spleen and liver. Thus, in systemic lupus erythematosus (SLE), deficiencies in CR1/CD35 on red blood cells and C1q, C2 and C4 contribute to immune complex deposition and subsequent sequelae in the skin and kidney. Conversely, complement activation promotes tissue damage in many autoimmune situations, such as in bullous pemphigoid [16, 17]. Complement measurement is therefore a useful clinical disease metric in both autoimmune and infectious contexts.
Fc Receptor-Mediated Cell Activation
In addition to antigen neutralization and complement activation, antibodies activate other arms of the immune system via binding to specific Fc receptors (FcR) on the surfaces of immune cells. All known human FcRs and their expression patterns and functions are outlined in Table 7.2. Specific FcR-mediated effector functions are described below.
Table 7.2
Fc receptors and their functions
Fc Receptor | Ig Ligand (Avidity) | Cell expression pattern | Downstream effector function |
---|---|---|---|
FcγRI (CD64) | IgG1,IgG3, IgG4 (high to very high) | Macrophages | Phagocytosis |
Monocytes | Endocytosis | ||
Neutrophils | Antigen presentation | ||
Eosinophils | Respiratory burst | ||
Mast cells | |||
Dendritic cells | |||
FcγRIIA (CD32) | IgG1, IgG3 (medium to high) IgG2, IgG4 (very low) | Macrophages | Phagocytosis, degranulation |
Monocytes | |||
Neutrophils | |||
Eosinophils | |||
Basophils | |||
Mast cells | |||
Platelets | |||
Langerhans cells | |||
FcγRIIB (CD32) | IgG3 (low) IgG1, IgG4 (very low) | T cells | Phagocytosis |
B cells | Feedback inhibition of B cells and macrophages | ||
Macrophages | |||
Monocytes | |||
Neutrophils | |||
Basophils | |||
Mast cells | |||
FcγRIIC (CD32) | All IgGs? (unclear) | NK cells (not in all humans) | Antibody-dependent cell-mediated cytotoxicity (ADCC) |
FcγRIIIA (CD16) | IgG1, IgG3 (medium) IgG2, IgG4 (very low) | T cells | ADCC by NK cells |
NK cells | |||
Macrophages | |||
Monocytes | |||
Neutrophils | |||
Mast cells | |||
FcγRIIIB (CD16) | IgG1, IgG3 (low to medium) | Neutrophils | Phagocytosis |
Basophils | |||
FcRn (*not a classical Fc receptor) | All IgGs | Syncytiotrophoblasts | Transports maternal IgG across placenta |
Endothelia | Maintenance of serum IgG levels | ||
Mucosal epithelia | |||
Hepatocytes | |||
FcεR1 (CD23) | IgE (high) | Mast cells | Degranulation |
Basophils | |||
FcεRII (CD23) | IgE (low) | B cells | Regulation of antibody response |
Eosinophils | Degranulation | ||
Langerhans cells | |||
Fcα/μR (CD351) | IgM, IgA1, IgA2 (medium) | B cells Mesangial cells | Endocytosis |
Intestinal cells | |||
Macrophages | |||
FcαR1 (CD89) | IgA (low to medium) | Monocytes | Phagocytosis |
Macrophages | Degranulation | ||
Neutrophils | |||
Eosinophils |
FcR-Mediated Opsonization and Phagocytosis
In addition to recognizing antigens opsonized with complement proteins, phagocytes bind immune complexes directly via binding of phagocyte FcR to Fc portions of immune complexes. Macrophages, dendritic cells and neutrophils bind and phagocytose pathogens opsonized with antibody, then degrade the pathogens within phagolysosomes. Antibody-mediated phagocytosis is the major form of defense against encapsulated bacteria such as pneumococcus.
FcR-Mediated Antibody-Dependent Cellular Cytotoxicity
FcγIIIA is expressed on natural killer (NK) cells and binds to multiple IgG subclasses. Binding initiates a process known as classical antibody-dependent cell-mediated cytotoxicity (ADCC), in which the opsonized cell is brought into close proximity to the NK cell, which then discharges granules to lyse the cell. ADCC is one mechanism by which therapeutic antibodies deplete target cells in autoimmune disease and cancer.
FcR-Mediated Eosinophil and Mast Cell Degran-ulation
IgE:FcεRI ligation can trigger degranulation by eosinophils or basophils. Parasites such as helminthes evade phagocytosis because of their large size. When opsonized with IgE, they can bind to FcεR1 on eosinophils which then destroy them by degranulation. IgE:FcεRI-induced eosinophil and mast cell degranulation also play important roles in tissue damage in asthma, urticaria and other allergic disease.
FcR-Mediated Antibody Recycling and Transport
The neonatal FcR, FcRn, is expressed in the placenta, neonatal intestinal epithelium and adult vascular endothelial cells [18]. It binds maternal IgG in blood or milk and transports it across the placenta or intestinal lumen, providing short-term passive immunity to the fetus and neonate. It also plays a homeostatic role by recycling serum IgG and protecting it from catabolism. In a therapeutic context, it has been hypothesized that high dose intravenous immunoglobulin (pooled IgG from donors) competes with pathogenic auto-antibody for binding to FcRn, resulting in more rapid degradation of pathogenic IgG antibodies [19].
FcR-Mediated Downmodulation of the Immune Response
FcR activity is not exclusively activating. FcγRIIB binds all IgG subclasses with low affinity and this binding can mediate endocytosis of immune complexes as well as triggering of negative signaling cascades that dampen the immune response. FcγRIIB is expressed on B cells and tranduces downregulating signals via immunoreceptor tyrosine-based inhibition motifs (ITIMs). FcγRIIB influences peripheral tolerance by binding low-affinity, autoreactive IgG+ B cells and blocking clonal expansion [20] and, in the context of a productive immune response can negatively regulate B cell activation [20]. FcγRIIB, is also involved in plasma cell survival [21].
Factors That Modulate Antibody: FcR Interactions
Antibody glycosylation can affect binding to FcRs and thus can also affect antibody effector function. Additionally, FcγR polymorphisms can affect binding of the receptor to IgG and this in turn can lead to increased susceptibility to autoimmune diseases and infections. Several FcγR alleles have been associated with increased risk for developing systemic lupus erythematosus, rheumatoid arthritis and Wegener’s granulomatosis, amongst other autoimmune diseases [22]. In a murine model of bullous pemphigoid, mice deficient in activating FcγRs, but not in the inhibitory FcγRIIB, have a reduced disease phenotype [23]. Thus, dysregulated or deficient expression of FcγRs can have a variety of effects as these receptors both promote and negatively regulate the immune response.
Summary
Antibodies contain two identical antigen binding domains (Fab domains) and a single effector domain (Fc region). Antigen binding diversity in the mature naïve B cell repertoire results from somatic recombination of assorted Variable, Diversity and Junctional (V, D, and J) gene segments and editing at gene segment junctions during the recombination process. Upon activation, some B cells undergo class-switch recombination, resulting in antibodies with the identical antigen specificity but different heavy chain isotypes (IgG, IgA, IgE), each with specialized effector functions. One role of antibodies is to directly neutralize pathogens. Via complement activation or engagement of Fc receptors on a variety of immune cells, antibodies can also trigger effector processes that result in pathogen destruction. Such effector functions include phagocytosis, degranulation and antibody-dependent cell-mediated cytotoxicity and require the recruitment of arms of the innate immune system including NK cells, neutrophils, eosinophils, mast cells and macrophages. Lastly, antibodies play immunomodulatory functions. Antibody binding to Fc receptors on B cells provides feedback inhibition to activated B cells. Binding to Fc receptors on epithelia and endothelia regulates protective antibody levels in the blood and tissues.
B Cell Development and Tolerance
Introduction
In 1965, Max Cooper formally identified the cells that give rise to antibodies and the humoral response [8]. Dr. Cooper was working with chickens, where the cells are derived from the Bursa of Fabricius, and thus the term “B” cell was coined. In mammals, B cell lymphopoiesis occurs primarily in the bone marrow. B cells are continually produced throughout life, though generation slows with advanced age [24].
B cells, T cells and NK cells originate from a common lymphoid progenitor cell, which is in turn derived from a pluripotent hematopoietic stem cell (Fig. 7.2). B cell development can broadly be divided by geography – initial stages (pro-, pre- and immature) occur “centrally” in the bone marrow. The last two developmental stages (transitional and mature) occur in the periphery, which includes secondary lymphoid organs such as the spleen and lymph nodes. B cell developmental stages are defined by the expression of markers that are turned on and off and by rearrangement of the IgH and IgL loci. Developing B cells, like T cells, pass through both positive and negative selection checkpoints. The BCR must bind ligand to be positively selected; however, strong binding to self-antigen will generally trigger negative selection. The vast majority of developing B cells will die or be rendered unresponsive due to unsuitable and/or autoreactive BCRs.


Fig. 7.2
B cells develop in an ordered maturation process that includes central and peripheral tolerance checkpoints and is characterized by distinct marker expression and survival factor dependence. Development stages include the hematopoietic stem cell (HSC), common lymphoid precursor (CLP), pro-B, pre-B, immature (Imm) B cell, transitional (Tr) B cell and the mature (Mat) B cell. Timing of B cell receptor (BCR) checkpoints and tolerance mechanisms are depicted. Following antigen stimulation, mature B cells can differentiate into memory B cells (MBC) or antibody-secreting plasmablasts (short-lived) or long-lived plasma cells. MBCs and long-lived plasma cells constitute humoral memory. Therapeutically, specific developmental populations of B cells can be deleted via the monoclonal antibody, belibumab, which blocks the soluble survival factor, BLyS/BAFF or rituximab, a chimeric antibody which targets CD20+ B cells
In this section, we will review the basic steps in B cell lymphopoiesis and highlight two monoclonal antibodies that selectively deplete B cells at specific developmental stages. We will also describe B cell-intrinsic and B cell-extrinsic mechanisms – in both the bone marrow and in the periphery – that prevent the proliferation of autoreactive B cells in healthy individuals.
Developing B Cells Must Productively Rearrange Both the Immunoglobulin Heavy and Light Chain Loci to Exit the Bone Marrow
The first cell in the B cell development assembly line is known as a pro-B cell, which differentiates from the common lymphoid progenitor cell. The pro-B cell undergoes rearrangement at the IgH locus (first D to J and then V to DJ). If rearrangement on the first allele results in an in-frame V(D)J, the IgH locus on the other allele is turned off in a process known as allelic exclusion, ensuring that the B cell expresses an IgH of a single specificity. Should the first allele have a nonproductive V(D)J rearrangement (a sequence that cannot result in a properly folded, functional IgH molecule), the IgH locus on the second allele undergoes rearrangement. Nonproductive V(D)J rearrangements on both alleles results in death of the pro-B cell.
Productive IgH rearrangement allows the pro-B cell to progress to the pre-B cell stage. At this stage the IgH protein pairs with a surrogate IgL to form a pre-BCR. Meanwhile, the pre-B cell undergoes rearrangement at the IgL locus (first the kappa (k) light chain genes, then, if they fail, the lambda (λ) light chain genes). Again, the principle of allelic exclusion applies: an in-frame VJ IgL rearrangement on one allele results in the remaining IgL locus on the other allele being turned off. Without productive rearrangement on any allele, the pre-B cell dies. If productive IgH and IgL rearrangements are achieved, the pre-B cell is termed an immature B cell.
Immature B Cells Are Evaluated for Strong Self-Antigen Binding
Immature B cells express a true surface BCR of the IgM isotype and express the co-receptors required for signaling. Via this BCR complex, immature B cells sample antigens within their environment. If there is little to no antigen recognition – indicating low autoreactivity – they shut off the recombinase activating gene (RAG) enzymes, preventing further rearrangement, and exit the bone marrow. If the BCR binds antigen more tightly – indicating potential autoreactivity – they die or undergo receptor editing, described below.
B cells exit the marrow as immature cells and undergo final stages of maturation in the periphery, termed the transitional B cell stage [25, 26]. Early transitional cells that bind tightly to antigen die via apoptosis in another critical checkpoint for potential autoreactivity, and later transitional cells appear to undergo positive selection events into the mature B cell pool [27]. Transitional B cells require stimulation with B cell activating factor (BAFF) to survive, and without it die before transitioning to maturity [28–30]. The half-life of transitional B cells is only a few days and in a healthy adult most cells die without entering the mature pool.
A mature B cell expresses its BCR in both the IgM and IgD isotypes and expresses markers that distinguish it from its precursors. Mature B cells continually recirculate via the blood and lymphatics through the spleen, lymph nodes, tonsils, bone marrow, mucosa and other tissues. These “naïve” (non-antigen activated) mature B cells sample the environment for cognate antigen to bind. Mature B cells do not self-renew and turn over every 2–4 months [31, 32]. They rely on stimulation with BAFF and ligand-independent tonic non-BCR signals for survival. When a naïve mature B cell binds cognate antigen via its BCR, the cell is activated and an immune response is initiated, as detailed later.
Tolerance Mechanisms Limit the Expansion of Autoreactive Lymphocytes
Given the incredible antigen-binding diversity in the naïve B cell repertoire, it may seem surprising that autoimmune diseases are not more common. In healthy humans there are two major check points where autoreactive B cells are managed: central (during early development in the marrow) and peripheral (during later stages of maturation outside of the marrow) (Fig. 7.2). Central tolerance mechanisms help manage immature B cells. It is estimated that approximately 75 % of the human immature B cell repertoire is self-reactive [33]. If an immature B cell binds antigen strongly, it can undergo receptor editing whereby RAG genes are re-expressed and mediate further recombination of light chain genes in an attempt to generate antibody of an alternate specificity [34]. Immature B cells that continue to bind antigen strongly die by apoptosis (negative selection).
As not all self-antigens localize to the bone marrow, autoreactive lymphocytes must be managed in the periphery as well. Peripheral tolerance is a collection of mechanisms that prevent transitional or mature self-reactive B cells from becoming activated. Peripheral tolerance mechanisms include deletion by apoptosis, functional inactivation by anergy or regulatory cells and physical sequestration of self-antigen. When peripheral tolerance is broken, pathogenic autoreactive responses can be initiated.
Deletion
Autoreactive B cells in the periphery can die by apoptosis when they encounter antigen in the absence of co-stimulatory signals. This can occur because cognate helper T cells were themselves deleted thorough negative selection or rendered anergic or because the self-antigens lack innate activating signals characteristic of pathogens.
Anergy and Hyporesponsiveness
After encounter with self-antigen, some B cells become functionally unresponsive, or anergic, to further simulation. Such cells express low levels of surface BCR and have an inhibited capacity to be activated. For example, anergic B cells upregulate SHIP1 [35], a phosphatase that negatively regulates BCR signaling [36]. Additionally, self-reactive B cells may be more dependent on BAFF stimulation for survival; in competition with non-reactive cells, many self-reactive B cells are disadvantaged [37–39], although this scenario does not necessarily apply to all self-reactive B cells [40]. Generally, anergic B cells have a reduced lifespan relative to other, non-autoreactive mature B cells [41].
Regulatory Cells
Specialized regulatory cells also play a role in the maintenance of peripheral tolerance. These cells are discussed later in the section on the immune response.
Mechanisms of Targeted B Cell Depletion
Unique surface marker ‘fingerprints’ for B cells at different developmental stages can be used to develop therapeutic strategies to selectively deplete B cell subpopulations. One such marker is CD20 (Fig. 7.2), a B cell-specific transmembrane protein with no defined function or ligand to date [42]. CD20, commonly used as a B cell identification marker, is expressed on pre-, immature-, mature and memory B cells, but is downregulated on plasmablasts and long-lived plasma cells. Rituximab is an anti-human CD20 chimeric monoclonal antibody that depletes CD20-expressing B cells via a combination of antibody-dependent cellular cytotoxicity, complement-mediated cellular toxicity and directly inducing apoptosis [43]. Rituximab treatment depletes most B cell precursors, mature and memory B cells, but spares lymphoid and pro-B precursors and long-lived plasma cells in the marrow. Thus, following rituximab-mediated B cell depletion, the B cell pool will slowly reconstitute itself. Furthermore, vaccine-induced and naturally acquired standing antibody titers to pathogens are spared. Rituximab is approved by the U.S. Food and Drug Administration for the treatment of B cell lymphoma, rheumatoid arthritis and ANCA-associated vasculitis and is used off-label to treat systemic lupus erythematosus and immunobullous disorders [44].
Another approach to B cell depletion is to target soluble factors required for their survival. One successful target is B cell activating factor (BAFF; also known as BLyS). BAFF is a TNF superfamily ligand that binds BAFF-receptor (BAFF-R) on transitional and mature B cells and is required for their survival [28, 45, 46]. Memory B cell survival as a whole is independent of BAFF [47], although subsets differ in their dependence [48]. While plasma cells utilize BAFF signals to survive, they are not absolutely dependent on BAFF. APRIL, a ligand related to BAFF, can compensate for BAFF loss. BAFF is an appealing therapeutic target in B cell mediated autoimmunity because some autoreactive B cells may be more BAFF-dependent than non-autoreactive populations [49, 50]. Belimumab is a human monoclonal antibody that targets BAFF, thus inhibiting receptor binding. Belimumab treatment depletes immature and mature B cells but spares plasma cells and the majority of memory B cells. As with CD20-targeted depletion, the B cell pool can reconstitute as precursor populations in the bone marrow are spared. In 2011, the U.S. Food and Drug Administration approved belimumab for the treatment of systemic lupus erythematosus (SLE), the first drug approved for the treatment of SLE since hydroxychloroquine in 1955 and aspirin in 1948 [51, 52]. Related drugs are in development, including ones that block BAFF via alternate mechanisms or that block BAFF and APRIL simultaneously.
Summary
B cell development is an ordered maturation process that starts from a pluripotent hematopoietic stem cell in the bone marrow and concludes with a mature B cell in the periphery. The goal of early stages of development is the formation of a B cell precursor bearing a fully functional B cell receptor of a single antigen specificity. Next, developing B cells are evaluated for autoreactivity (central tolerance). Immature B cells with strongly reactive B cell receptors can be deleted directly or can undergo receptor editing, in an attempt to generate receptor of a different specificity. Next, newly generated transitional B cells exit the bone marrow and undergo final stages of maturation and selection in the periphery. This population includes cells with weak autoreactivity, which can be managed though deletion or other mechanisms that suppress their activity (peripheral tolerance). Finally, a minority of transitional B cells are positively selected into the mature B cell pool. This mature B cell pool is comprised of B cells of diverse antigenic specificities. Mature B cells continually recirculate through the spleen, lymph nodes, marrow and peripheral tissues via the blood and lymphatics, surveilling for infection.
Breaks in central and/or peripheral B cell tolerance underlie autoimmune disorders such as systemic lupus erythematosus, rheumatoid arthritis and immunobullous skin disease. Malignant proliferation of B cell populations results in lymphoma. Targeted monoclonal antibodies that deplete B cells can be effective therapies for B cell malignancies and B cell-mediated autoimmune disease. Such drugs include rituximab and belimumab, which target CD20 or BAFF-R expressing B cell subpopulations.
B Cells in the Immune Response
Introduction
Upon binding to its cognate antigen, a B cell becomes activated. B cell receptor activation is intrinsically regulated – positively and negatively – through accessory surface proteins. Different types of antigens and the involvement of CD4+ T cells shape the B cell response. A primary goal of the B cell response is to produce antibody, which binds the pathogen or pathogen-produced toxins, mediating a variety of effector functions as described above (Antibody Structure and Function). CD4+ T cell help enables another, more temporally distant goal – that of achieving long-term immunity (memory) to the pathogen.
This section will describe B cell signaling and B cell participation in the immune response. The focus is the T cell-dependent immune response, although the T-independent B cell response is reviewed as well. Specific interactions between T cells and B cells that profoundly influence B cell fate are highlighted.
Activation of the B Cell Receptor Signaling Complex Triggers a Protein Kinase Signaling Cascade
B cell activation begins with ligation of antigen to the BCR complex which triggers an intricate and highly regulated signaling cascade [53]. The BCR does not on its own trigger downstream signaling following antigen binding, but rather, it requires invariant transmembrane proteins Igα (CD79a) and Igβ (CD79b) – which each have immunoreceptor tyrosine-based activation motifs (ITAMs) – to initiate a protein kinase signaling cascade. These proteins associate with a portion of the immunoglobulin heavy chain constant region and are phosphorylated by associated kinases. The phosphorylation cascade culminates in the activation of phospholipase C-γ (PLC-γ) and guanine-nucleotide exchange factors (GEFs). PLC-γ cleaves a membrane phospholipid, resulting in an increase in intracellular Ca++ levels. This in turn leads to the activation of two transcription factors: NF-kB and NFAT. Guanine-nucleotide exchange factors activate a MAP kinase cascade that leads to the activation of another transcription factor, AP-1. Together, these three transcription factors turn on genes important for proliferation, differentiation, antigen presentation and cytokine production.
BCR signaling is tightly regulated. At the initiation stage, crosslinking of two or more BCRs with antigen is required for triggering the downstream signaling cascade (Fig. 7.3). Subsequently signaling can be both positively and negatively modulated. The B cell coreceptor complex – CD19, CD21/CR2 and CD81 – is activated by antigens opsonized with complement and augments BCR signaling by recruiting one of the guanine-nucleotide exchange factors. BCR signaling can be negatively regulated by several B cell-intrinsic proteins, such as CD22 and FcγRIIB, that contain immunoreceptor tyrosine-based inhibitory motifs (ITIMs) in their cytoplasmic tails. ITIMs recruit the phosphatases SHIP1 and SHP1, which dephosphorylate and thus deactivate kinases involved in the BCR signaling cascade. SHIP1 and SHP1 are upregulated in germinal center B cells and may be involved in their selection by increasing the minimum threshold for BCR signaling, thus favoring higher-affinity B cells [36]. Anergic B cells – B cells that are unresponsive to antigen stimulation – have chronic activation of SHIP1 [35].


Fig. 7.3
B cell activation requires B cell receptor (BCR) cross-linking and is augmented by additional signals. B cell activation is initiated by antigen (Ag) binding induced BCR cross-linking. Additionally, the BCR co-receptor complex – CD21/CR2, CD19 and CD81 – can further enhance signaling. CD21/CR2 binds complement-coated microbes and activates CD19. Toll-like receptors (TLRs) recognize motifs unique to different classes of pathogens and further activate B cells, thus linking the innate and adaptive immune systems
In addition to recognizing antigen via the BCR, B cells engage antigen or modified antigen via surface receptors, particularly Toll-like receptors (TLRs), CD21/CR2 and FcγRIIB. The latter two proteins manipulate the BCR signaling cascade by binding complement-bound antigen or IgG-bound antigen respectively. TLR activation can exquisitely tune B cell antigen presentation, differentiation and class-switch during the B cell immune response [54].
B Cells Responses to Lipid and Polysaccharide Antigens Do Not Require T Cells
Humoral responses are the principal defense against polysaccharide and lipid antigens, and T cells are not required to mount these “T-independent” (TI) responses. In TI responses, antigen binding induces B cell activation, proliferation, expansion and differentiation into antibody-secreting plasma cells. Resultant antibodies are typically IgM and low affinity, identical to those produced by their naïve precursors. There are two forms of T independent responses, classified by antigen type. Type I responses involve pathogens that engage B cell Toll-like receptors (TLRs) as well as the BCR. Type II responses involve polysaccharide antigens that exclusively activate the BCR and induce strong cross-linking because of their repeating antigenic epitopes. T-independent type II antigens can elicit both memory B cells and a long-lasting antibody response [55–57], although antibody titers are not boosted upon repeat immunization [58]. Polysaccharide-based vaccines include Pneumovax and Menomune, which provide protection against pneumococcus bacteria and bacterial meningitis, respectively.
B Cell Responses to Protein Antigens Require Cognate B-T Interactions
An effective humoral responses against protein antigens requires both B and T cells is and is termed a “T-dependent” (TD) response. B cell responses primarily occur in secondary lymphoid organs such as the spleen or lymph node. B cell activation occurs when a naïve B cell binds cognate antigen, triggering intracellular signaling and receptor-mediated endocytosis of antigen. Antigen is processed and the resultant peptide fragments presented on MHC class II molecules for display to CD4+ T cells.
B and T cells are located in separate areas of the spleen and lymph node, so during early activation they must be brought into close proximity. Activated B cells alter expression of chemokine receptors, downregulating receptors for chemokines expressed in the B cell follicle and upregulating receptors for chemokines expressed in T cell zones. Thus, activated B cells migrate toward T cells and, similarly, dendritic cell-activated T cells migrate towards B cells. When B and T cells specific for the same antigen meet and have a cognate MHC Class II:TCR interaction, the T-dependent response is initiated. Additional direct interactions between B cells and T cells further stabilize the conjugate.
The B cell response to T-dependent antigen is highly orchestrated and occurs in overlapping stages (Fig. 7.4). The early “extrafollicular” response, so called as it occurs outside of the B cell follicle at the T cell zone – follicle border, is characterized by B cell proliferation and differentiation into short-lived plasmablasts that secrete copious amounts of low affinity antibody. This early extrafollicular response is important for gaining rapid control of a new infection, and in the case of persistent self-antigen, can contribute to autoimmunity [59, 60]. Some memory B cells are formed during this time, but are typically unmutated and of low-affinity compared with those that are generated later in the response [61, 62].


Fig. 7.4
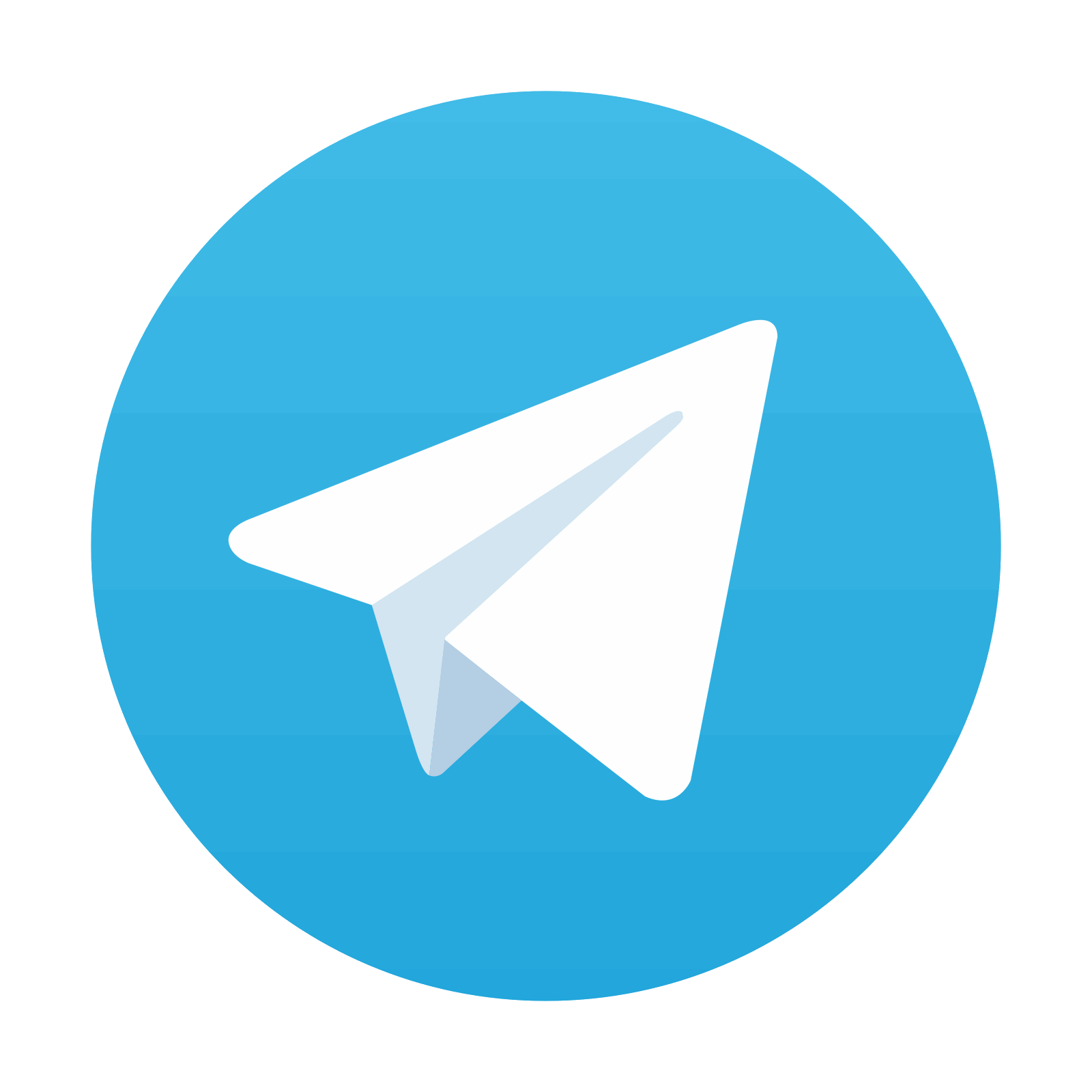
B cells in the immune response. Following binding to its cognate antigen (Ag), the naïve B cell (Nve B) presents antigen-derived peptide to an activated CD4+ T cell and is itself activated. Rapid proliferation results in the formation of an extrafollicular focus of B cells (EF B), which is characterized by rapid differentiation into short-lived plasmablasts (PB) that produce a large burst protective low-affinity IgM antibody (Ab). With time, some B and T cells can seed a germinal center (GC). Germinal center B cells (GCBC) proliferate rapidly and interact with follicular dendritic cells (FDC) and T follicular helper cells (TFH). Somatic hypermutation, competition between B cell clones and affinity maturation yield B cells with higher affinity for antigen. Class-switch recombination produces clones with switched immunoglobulin isotypes. Ultimately, the germinal center produces long-lived plasma cells (LLPC), terminally differentiated cells that exclusively produce antibody, and memory B cells (MBC). Upon antigen re-stimulation in a secondary (2°) response, MBC rapidly differentiate into plasmablasts or form new germinal centers and undergo further affinity maturation
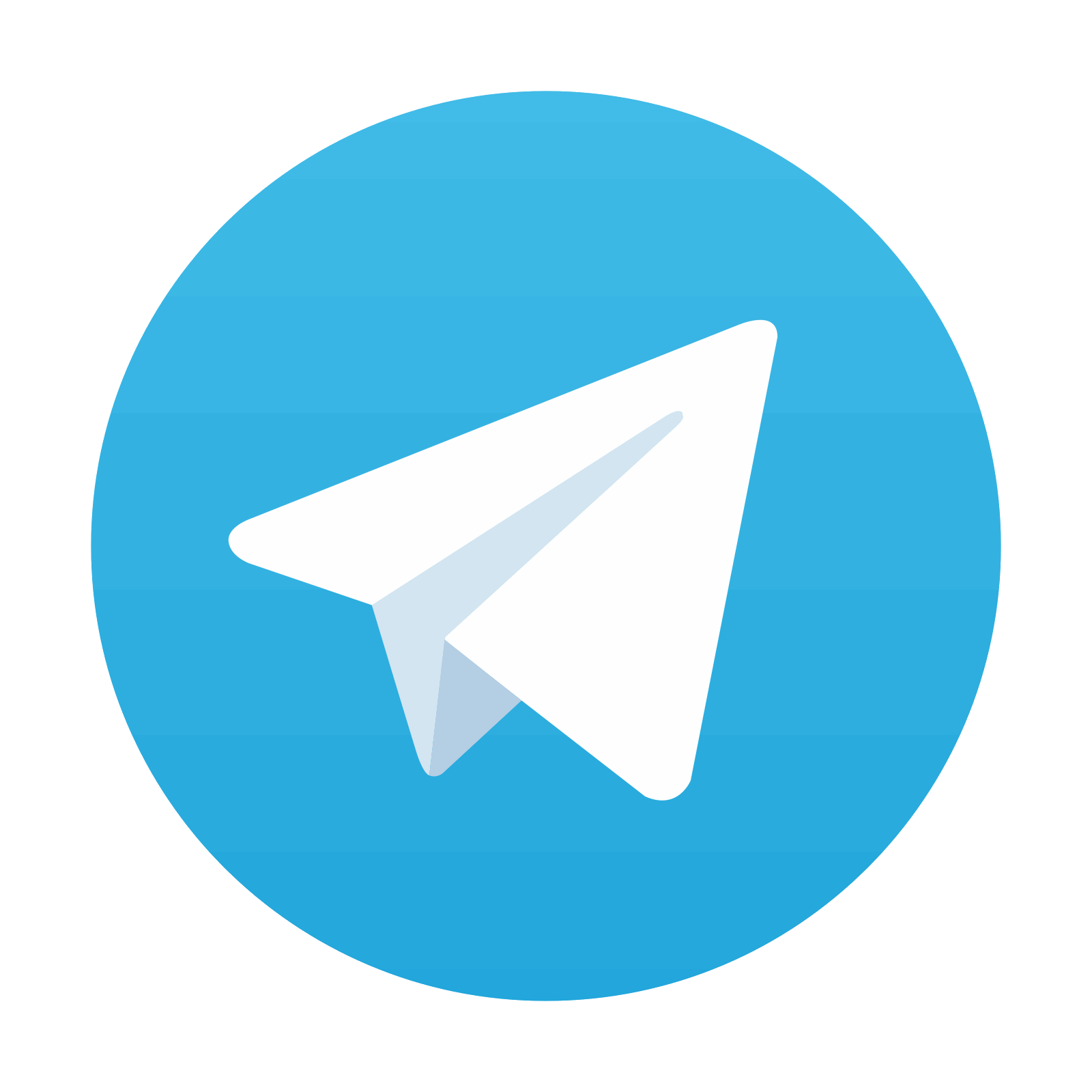
Stay updated, free articles. Join our Telegram channel

Full access? Get Clinical Tree
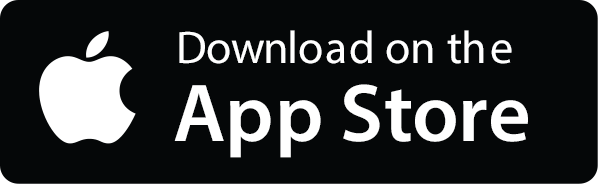
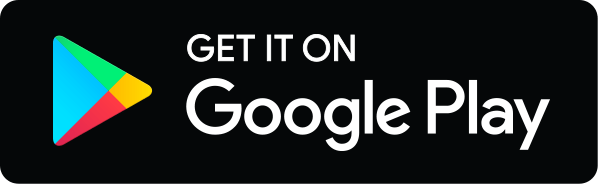
