Fig. 29.1
Alopecia areata, patchy type. Nonscarring patches of alopecia on the posterior scalp of an AA patient

Fig. 29.2
Alopecia areata totalis. Alopecia involving the entire scalp in a patient with AT
Genetic Architecture of AA
Human genetic studies in AA have played a pivotal role in understanding its etiology. Initial evidence for a genetic basis to the disease arose from family studies that demonstrated increased risk of disease in first degree relatives [3, 4], twin studies [5, 6], and studies in animal models [7]. The earliest genetic studies, limited by the availability of high throughput cost-efficient technologies for genotyping or sequencing, utilized a candidate gene approach testing only a few variants in pre-selected genes, utilizing small cohorts. These initial genetic studies demonstrated associations to several immune related genes, including genes within the human leukocyte antigen (HLA) complex such as HLA-DQB1, HLA-DRB1, HLA-A, HLA-B, HLA-C, NOTCH4, MICA, as well as PTPN22 and AIRE, which are located outside the HLA. While these studies provided consistent support to the theory of an autoimmune basis for the disease, they were limited in generating new etiological insight. With the advent of commercial single nucleotide polymorphism (SNP) genotyping arrays, it became feasible to query the entire genome in large cohorts of patients and controls, enabling unbiased testing in well-powered studies. The first genome-wide association study (GWAS) conducted for AA identified eight regions in the genome with statistically significant evidence for association [8]. Of these, only the HLA had previously been implicated in AA. A subsequent replication study utilizing this GWAS data and genetic data in an independent cohort identified two additional loci in the genome [9]. Most recently, the first meta-analysis GWAS was performed in AA in a cohort of 10,000 samples. The substantial increase in sample size increased the power to detect association, and four additional loci were at or near genome-wide statistical significance [10].
GWAS loci typically contain multiple genes, but the technique does not allow individual genes within loci to be prioritized for etiological significance. In order to gain mechanistic insight from GWAS evidence, pathway analysis is used to identify sets of functionally related genes. In AA, the 14 GWAS loci contain 226 protein coding genes and pathway analysis demonstrates enrichment of genes involved with a small number of biological processes (Table 29.1). For instance, pathways that implicate specific immune perturbances include T-cell activation/differentiation, JAK-STAT signaling, and viral defense response. There are additional pathways implicated by GWAS that suggest disease mechanisms that could be operating in either cells of the immune system, or alternatively, within the hair follicle itself, including oxidation-reduction, apoptosis, and steroid hormone signaling.
Table 29.1
GWAS loci in AA implicate biological processes perturbed in disease
Pathway, GWAS locus (pathway gene) |
---|
T cell activation, co-stimulation, and differentiation 1p13.2 (PTPN22), 2q33.2 (CD28, CTLA4), 4q27 (IL2, IL21), 5q31.1 (IL13, IL4, IL5, IRF1), 6p21.32 (HLA-DMA, HLA-DOA), 10p15.1 (IL2RA, PRKCQ), 11q13.1 (BAD), 12q13 (IL23A, IKZF4 (Eos)), 16p13.13 (CIITA) |
JAK-STAT signaling 4q27 (IL2, IL21), 5q31.1 (IL13, IL4, IL5), 10p15.1 (IL2RA, IL15RA), 12q13 (IL23A, STAT2), 16p13.13 (SOCS1) |
Viral defense response 5q31.1 (IRF1, IL4), 6q25.1 (ULPB3, ULBP6 (RAET1L)), 10p15.1 (IL2RA), 11q13.1 (BAD) |
Steroid Hormone Signaling 6p21.32 (RXRB), 9q31.1 (NR4A3), 11q13.1 (ESRRA) |
Oxidation Reduction 2q13 (ACOXL), 5q31.1 (P4HA2, UQCRQ), 6p21.32 (HSD17B8), 6q25.1 (IYD), 11q13.1 (PRDX5), 12q13 (SUOX, RDH5, GLS2), 12q24.12 (ALDH2, ACAD10) |
Apoptosis 10p15.1 (IL2RA), 11q13.1 (MEN1, PRDX5, BAD), 12q13 (ERBB3, GLS2), 16p13.13 (LITAF), 1p13.2 (HIPK1, MAGI3, BCL2L15), 2q13 (BCL2L11), 2q33.2 (CD28), 4q27 (IL2, FGF2), 5q31.1 (IL4) |
Of clinical relevance, some of the etiological pathways that came to light as a result of these genetic studies are targeted by therapeutics already in use to treat other autoimmune diseases, but had not previously been considered within the context of AA treatment. For example, the costimulatory pathway is targeted by abatacept, and JAK-STAT signaling is therapeutically targeted by ruxolitinib and tofacitinib, discussed further below. Trials to test these interventions in the treatment of AA have yielded promising early results [30]. Thus GWAS in AA have rapidly realized the initial promise of the human genome project, to improve the care of patients.
Hair Follicle Immune Privilege
An ‘immune privileged’ (IP) site was initially defined as a body site to which foreign grafts could be placed without being rejected [11]. This concept was first described by Peter Medawar, after conducting experiments in rabbits demonstrating relative resistance to rejection of allogeneic skin grafted into the anterior chamber of the eye [12]. IP was extended to describe not only sites or organs but also tissues, which were so defined by their relative resistance to rejection if transplanted onto conventional, non-immune privileged sites [13, 14]. Immune privileged sites include the eye, brain, gametes, pregnant uterus, as well as the hair follicle. Indeed, early experiments in which black skin from guinea pigs was grafted onto immunologically nonreceptive white skin of a different guinea pig strain showed rejection of donor skin but prolonged retention of the donor hair follicles [13, 15, 16]. More recently, hair follicle cells from a human male donor were implanted into the skin of an unrelated female, without evidence of rejection [17].
Many factors contribute to IP in the hair follicle. HLA expression is minimal in the hair follicle [18, 19] below the level of the isthmus, preventing recognition of cell-associated antigens by T cells. The hair follicle also employs various methods to avoid or minimize recognition and attack by natural killer (NK) cells, which may be expected due to low HLA expression, including the expression of macrophage migratory inhibitory factor and relative absence of ligands of NK cell stimulatory receptors [20, 21]. Evidence exists that the local environment is comprised of other immunoregulatory proteins, such as TGF-β1 [22], α-MSH [23, 24], and cell-associated PDL1 [25] that may be serving to inhibit immune responses to hair follicle-associated antigens [26]. Lastly, there is an alteration and overall diminution in immune cells that may be contributing to inflammation. Langerhans cells, an immune component of the skin epidermis, are relatively deceased in numbers below the level of the isthmus [19, 27], as are T cells [19]. The contribution of these cells to pathologic states where IP is altered is currently an active area of research.
AA is associated with a collapse of the immune privileged status of the hair follicle [26]. Hair follicles within and adjacent to alopecic patches in AA patients exhibit marked expression of HLA molecules [28], and infiltration of the hair follicle with CD4 and CD8 T cells is observed in AA lesions (Fig. 29.3), in a pattern termed the “swarm of bees” [1, 29]. Furthermore, the hair follicle begins expressing ligands for NK cell stimulatory receptors, including MICA [20] and several ULBP molecules [8]. The cytokine milieu is also skewed toward a proinflammatory profile, exemplified by the marked increase in the expression of interferon (IFN)-response genes as well as common γ-chain (γc) receptor cytokines in AA lesions [30]. This loss of IP in the hair follicle is likely a necessary step for antigenic responses by the immune system and clinical disease in AA.


Fig. 29.3
Peribulbar CD3 infiltrate in AA. CD3 immunohistochemical staining of hair follicles in a normal patient (left panel) and AA patient (right panel). The peribulbar infiltrate has been likened to a “swarm of bees”
Cellular Immune Effectors in AA
Much of the work to identify the cellular pathogenic mediators of AA has been conducted in animal models. The C3H/HeJ mouse is a murine model of spontaneous AA development, wherein approximately 10–20 % of mice develop nonscarring alopecia that replicates many features of the human disease [31]. Subcutaneous injection of unmanipulated lymph nodes cells from alopecic C3H/HeJ mice to unaffected mice resulted in hair loss after 5 weeks, implicating the presence of an immune effector mediating the disease [32]. Another model of alopecia, in which human punch biopsy samples from normal patients are grafted onto immunodeficient recipient mice, requires the subcutaneous injection of peripheral blood mononuclear cells after culture in IL-2, corroborating the necessity of a cellular immune effector [33]. More recently, spurred by multiple lines of evidence including (1) the recognition of increased expression of NKG2D ligands in AA skin biopsies [8, 20], (2) our identification of a genetic association between AA and NKG2D ligands [8], and (3) the presence of CD8+NKG2D+ cells surrounding the hair follicle in patients with AA, adoptive transfer studies were performed demonstrating that CD8+NKG2D+ cells from skin draining lymph nodes of alopecic C3H/HeJ mice were necessary and sufficient to induce systemic disease in previously unaffected C3H/HeJ mice [30]. Several lines of evidence support the NKG2D-NKG2D ligand interaction as a critical component of disease, including the upregulation of NKG2D ligands in the hair follicle of AA patients [8] and affected C3H/HeJ mice [30] as well as the known capacity of NKG2D to potentiate antigen-induced T cell responses [34, 35]. These identify CD8+NKG2D+ T cells as critical players in AA pathogenesis.
Other T cell populations are also likely influencing the development of AA. CD4 T cells comprise part of the peribulbar infiltrate seen in lesions from AA patients [20], and the strongest AA genetic associations have been with HLA Class II molecules [8], which present peptides to CD4 T cells. Adoptive transfer studies in the C3H/HeJ model indicate that CD4 T cells from the lymph nodes of affected mice were sufficient to induce the disease in unaffected, AA-prone mice [36]. Experiments in which the CD4 T cell population have been segregated based on CD25 expression, putatively separating regulatory T cells and conventional CD4 T cells, indicate that the CD4+CD25–, or conventional T cell, population is the more potent subgroup of this population, whereas CD4+CD25+, or Treg, cells have a protective role and act to prevent the development of AA [36]. In humans, analyses of serum cytokines in AA patients show reduced levels of circulating TGF-β1 and elevated levels of IL-2, IFN-γ, IL-13 and IL-17, supporting a reduction in the ratio of Treg/conventional T cell activity [37].
The role of NK cells is currently less clear. Although the expectation may be that NK cells would be playing a pro-inflammatory role in AA pathogenesis, data exists supporting an immunoregulatory role for NK cells [38]. The mechanisms of this immunoregulation have not yet been elucidated. It has become challenging to interpret prior studies interrogating the role of NK cells in AA, and the recent discovery of a CD8 T cell population that co-expresses NK markers in AA invites a re-evaluation of prior studies examining NK cells.
Mast cells have also been described in increased numbers around the hair follicle and in the perifollicular dermis in patients with AA [39]. However, studies of mast cells in AA have been limited, although mast cells are able to act as professional antigen presenting cells [40, 41] and elaborate T cell chemotactic molecules [42]. While the mast cells in AA lesions exhibit a pro-inflammatory profile skewing [39], their contribution to AA pathogenesis is unclear.
Macrophages also make up part of the peribulbar infiltrate in AA. In the normal murine hair cycle, the transition from telogen to anagen is marked by a decrease in the number of macrophages, and experimental depletion of macrophages hastened the transition from telogen to anagen [43]. The heightened presence of these cells in AA may indicate that macrophages may be playing in a role in regulating the hair cycle in AA, although this has yet to be substantiated.
Cytokines in AA
IFNs have been implicated in the induction of AA in human disease and in mouse models. Scalp lesions in AA exhibit a strong IFN signature [30], with both type I and II IFNs likely contributing. In particular, IFN-γ, the sole member of the type II IFN family, appears to strongly influence the immune status of hair follicles. Injection of IFN-γ into C3H/HeJ mice reverses the IP status of the hair follicle, demonstrated by increased MHC Class I and II expression, T cell infiltration, and hair loss [44], although some studies of this effect have shown contrasting results [45]. Genetic ablation of IFN-γ prevented the development of spontaneous AA in C3H/HeJ mice [46]. Furthermore, in a modified murine AA model in which alopecic C3H/HeJ skin has been grafted onto previously unaffected C3H/HeJ recipients, which induces hair loss in 90–100 % of graft recipients, neutralizing antibodies to IFN-γ prevented the development of AA [30].
Evidence that type I IFNs, including IFN-α and IFN-β, contribute to the development of AA can be inferred from studies in which patients were treated with IFN for other medical conditions. IFN-α is used as a treatment for chronic hepatitis C, and case reports exist exhibiting the development of AA with treatment and subsequent resolution after the treatment course is completed [47, 48]. Examinations of lesional biopsies from patients with AA demonstrate expression of MxA [49], an IFN-stimulated gene. However, more extensive studies examining the necessity and sufficiency of type I IFNs in AA pathogenesis, for example in mouse models, are lacking.
The participation of certain γc receptor cytokines, especially IL-2 and IL-15, has also been implicated in the development of AA. IL-2 and IL-15 function in many ways in the immune system, including providing survival and proliferative signals to T cells. IL-15 can also induce the expression of NKG2D on T cells and can prime NKG2D signaling [50]. IL-2 haploinsufficent C3H/HeJ graft-recipient mice were relatively protected from developing AA, compared to IL-2 sufficient control graft recipients [51]. Antibody treatment targeting IL-2 or the shared β subunit for the IL-2/IL-15 receptor prevented the development of AA in a mouse model of the disease [30]. In AA patients, IL- 15 is expressed at high levels on the hair follicle [30] and has been implicated in another autoimmune disorder, celiac disease [52–54]. Both IL-2 and IL-15 are likely crucial to the development of AA.
Putative Antigens in AA
It is currently unknown whether a shared specific antigen or set of antigens is the target of autoimmune attack for patients with AA. Several lines of evidence support the notion that an antigen-specific response is involved, including the genetic association between AA and HLA alleles [8], the congregation of T cells around hair follicles in skin samples of affected patients [1], and the ability to induce disease from one mouse to another by adoptive transfer of T cells [30, 36]. Attempts at identifying target antigens in AA have mostly focused on autoantibodies in patients with AA, and the role of antibodies in AA has not been defined. Autoantibodies against a hair follicle-associated antigen have been detected at higher levels in AA patients compared with normal controls, although the identity of this antigen is not known. Trichohyalin has been identified as an autoantibody target in patients with AA [55], although whether autoantibody formation to this or other putative antigens is critical to the pathogenesis of AA or a marker of hair follicle damage remains an open question.
Immunointerventions in AA
There are currently no US FDA approved drugs for the treatment of AA. A Cochrane systematic review conducted in 2008 concluded that there is no convincing trial evidence that any treatments provide long-term benefit to patients with any form of AA [56]. Interventions for AA have, for the most part, consisted of local or systemic immunosuppressive regimens that are relatively nonspecific in their effects and have included topical or intralesional glucocorticoids or steroid-sparing agents.
Glucocorticoids are likely the most commonly used treatment for AA. Glucorticoids inhibit the production of pro-inflammatory cytokines and chemokines and induce the apoptosis of dendritic cells and lymphocytes. Localized treatments are often performed using topical or intralesional delivery methods, and systemic treatment is usually performed only in the context of severe disease. No double blind, randomized controlled trials exist of these treatments, and it is unknown whether these treatments alter the long-term disease course.
Prolonged treatment with systemic steroids has the potential to cause many adverse effects. Steroid-sparing immunosuppressive agents are usually used in the context of severe disease and in cases where the need for a prolonged treatment course are suspected. These treatments include methotrexate, cyclosporine, azathioprine, and sulfasalazine. Only case reports exist of the use of these treatments for AA [57].
Topical immunomodulation is another treatment strategy used for AA. The rationale for these treatments is that the end-organ inflammatory milieu may be redirected to a different, non-hair follicle associated target or can be changed in such a way that it is no longer permissive for continued attack of the hair follicle by the immune system. Anthralin has been cited as a topical immunomodulator, likely due to its proinflammatory effects causing a dermatitis to applied areas. The time that anthralin is allowed to remain on the skin is often titrated to a maximum of 1 h or until a dermatitis is achieved. The mechanism by which AA is inhibited by this drug, however, is unknown.
Topical sensitization to either diphenylcyclopropenone or squaric acid dibutylester is another form of topical immunomodulation that is used for severe cases of AA. These treatments consist of a sensitization phase, in which an allergic response is generated to the drug, and a treatment phase, in which the drug is applied to the affected area with the intent of causing a low-level dermatitis and, secondarily, allowing hair regrowth. Studies in which half the head had been treated with the topical immunomodulator while the contralateral side was left untreated demonstrated some efficacy for these treatments in severe forms of AA, although the hair regrowth is often not sustained long-term [58]. The proposed mechanisms by which these drugs effectively treat AA include antigenic competition, in which an immune response to a separate antigen is elicited with accompanying regulatory/suppressor cells that quell responses to competing antigens [59]. Other studies have shown that DPCP also increases the number of infiltrating CD8 T cells in perifollicular and epidermal areas [60] and decreases the CD4:CD8 ratio [61], but it is unclear how this contributes to ameliorating hair loss in AA since CD8 T cells are thought to be the primary cellular effectors of disease.
Targeted treatments, specifically identified for AA or for immune pathways active in AA, have been lacking. The identification of candidate therapeutic targets for drug development have been recently spurred by results from a GWAS for AA in 2010 as well as gene expression profiling of affected skin from human AA patients and the C3H/HeJ mouse model [62]. Many of these treatments have been tested in the C3H/HeJ model, with a few beginning to be assessed in human AA patients. Antibodies to IFN-γ, IL-2, and the IL-2/15R β chain have shown their utility in the prevention of AA onset in the C3H/HeJ murine model. The efficacy of blocking these cytokine pathways in the murine model led to investigation into whether inhibiting the signaling pathways activated by these cytokines is also an effective treatment strategy.
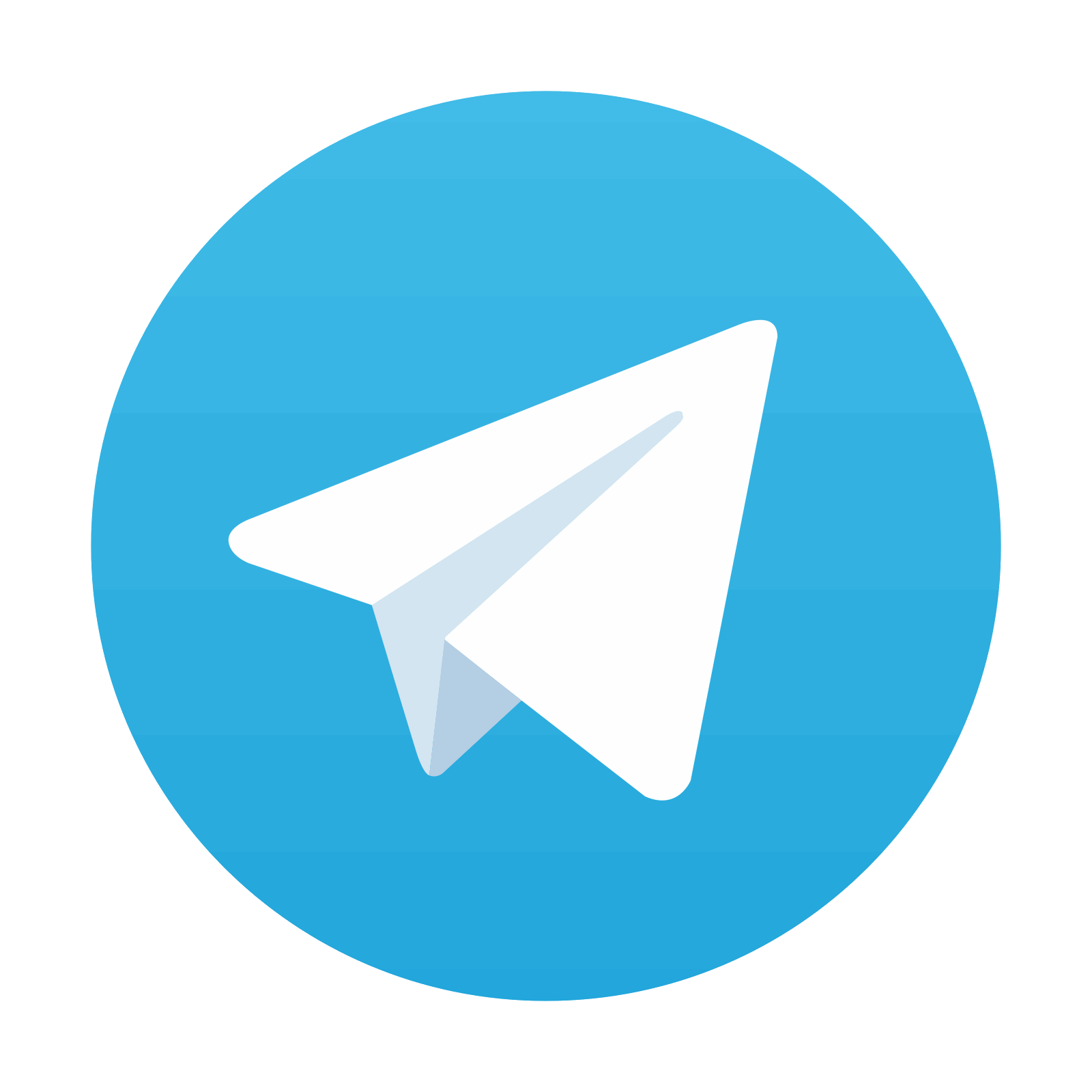
Stay updated, free articles. Join our Telegram channel

Full access? Get Clinical Tree
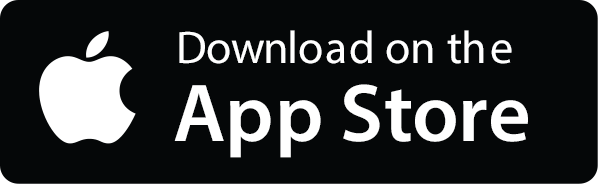
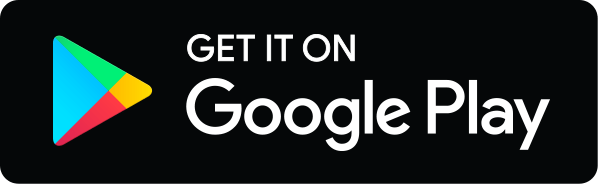