Sreekumar Pillai, Surabhi Singh, Christian Oresajo, and Nada Baalbaki L’Oréal Research and Innovation, Clark, NJ, USA Recent developments in new technologies combined with new knowledge in skin biology have advanced innovations in skin availability of actives and novel methods of substance delivery. The goal of this chapter is to review new advances in delivery of actives to the skin and the effects of penetration enhancers (PEs). An understanding of the structure of the skin is very important in managing active delivery. The goal of percutaneous delivery is to provide an effective amount of an active to the skin target site and thereby optimize efficacy while minimizing side effects. This can be achieved by an understanding of the skin’s complex structure and by relying on physical and chemical parameters of vehicles applied to the skin. There are defined compartments and biologic structures within the skin that provide opportunities to deliver actives (Figure 9.1). Within these compartments, there are many chemical and biologic processes at work that may alter a given active or the physiology of skin target. The main barrier of active permeation through the skin is the stratum corneum. This structure is located at the outermost layer of the epidermis [1]. In some cases, like with hydrating ingredients or ceramides, the stratum corneum is the target site for delivery. In other cases, the active must cross this skin barrier to reach the epidermis to be delivered to the target site. The transepidermal route can be further subdivided into transcellular and intercellular routes [2]. Delivery of hydrophilic substances can be achieved through sweat gland route; however, this is minimal in total volume. The penetration through sweat or sebaceous glands can be moderated by the secretion activity of the appendages. Therefore, the principal pathway for skin penetration of actives is the transepidermal route (route 1 in Figure 9.1). One of the first steps in understanding the phenomenon of active delivery is to completely characterize the active that is intended for delivery to the skin. There are well‐known physical and chemical parameters that are specific to all chemical compounds. The essentials for characterization of actives are typically described in the literature or can be measured in the laboratory. This includes the active’s molecular weight, dissociation constant (pK), solubility, and octanol/water [O/W] partition coefficient (log P). These parameters, along with a thorough understanding of the net ionic charge (cationic, anionic, and amphoteric) of the active, will help in understanding its penetration profile. As general rule, molecules with a molecular weight of less than 500 Da penetrate the skin better than molecules with a larger molecular weight. It is also known that the net charge of a molecule is important in enhancing penetration. An un‐ionized molecule penetrates the skin better than an ionized molecule. A thorough understanding of the relationship between the dissociation constant and formulation pH is critical. In many cases, it is advantageous to keep the pH of a formulation near the pK of the active molecule in an attempt to enhance penetration. When looking at the partition coefficient, molecules showing intermediate partition coefficients (log P O/W of 1–3) have adequate solubility within the lipid domains of the stratum corneum to permit diffusion through this domain while still having sufficient hydrophilic nature to allow partitioning into the viable tissues of the epidermis [3]. Figure 9.1 Possible pathways for a penetrant to cross the skin barrier. (1) Across the intact horny layer; (2) through the hair follicles with the associated sebaceous glands; or (3) via the sweat glands. (Source: Daniels R. Strategies for skin penetration enhancement. Skin Care Forum37, www. scf‐online.com.) The permeation of active across the stratum corneum is a passive process, which can be approximated by Fick’s first law: This defines the relationship between steady‐state flux (J) and the diffusion coefficient (D) of the active in the stratum corneum over a diffusional path length or membrane thickness (L), the partition coefficient (K) between the stratum corneum and the vehicle, and the applied drug concentration (C) which is assumed to be constant. Novel formulation strategies allow for manipulation of the partition coefficient (K) and concentration (C). Skin penetration can be enhanced by the following strategies: Equation (9.1) aids in identifying the ideal parameters for the diffusion of the active across the skin. The influence of solubility and partition coefficient on diffusion across the stratum corneum has been extensively studied in the literature [5]. The key for evaluating the vehicle effect is to understand the dynamics between the vehicle and the active. There are specific formulation strategies based on the physical and chemical nature of the active that can be designed to enhance its delivery. The primary vector for topical delivery of actives is a semi‐solid ointment or emulsion base. The main reason for selection of this dosage form is convenience and cosmetic elegance. Emulsions are typically composed of two immiscible phases (hydrophilic and hydrophobic) and an emulsifying agent. One phase, the internal phase, is dispersed as small droplets throughout the other, external phase. The most common emulsions, also referred to as a classical emulsion, are oil‐in‐water (o/w) emulsions. The consistency of an emulsion varies from thin liquids to soft, semi‐solid creams, and depends upon: The bi‐phasic nature of emulsions allows for placement of actives based on solubility and stability. This enables the formulator to bring both lipophilic and hydrophilic actives into the dosage form while maintaining the optimized stability profile. The effect of the type of this type of vehicle on skin penetration has been well described in the literature [6]. In addition to the typical bi‐phase emulsions, there are various other emulsion forms including nano‐emulsions and multiple emulsions. Nano‐emulsions are characterized by very fine dispersions of the internal phase (<100 nm). Multiple emulsions include both oil‐in‐water (o/w) and water‐in‐oil (w/o) emulsions within the same system; in a water‐in‐oil‐in‐water (w/o/w) emulsion, small water droplets are entrapped within oil droplets that are dispersed within the continuous water phase. This creates a reservoir effect, helping to protect actives from degradation and/or releasing ingredients at a controlled rate. There are several studies which show the impact of the type of emulsion, both typical emulsions and unique systems, on active delivery and release. An example of a unique type of emulsion used to control release is multivesicular emulsion (MVE) technology. MVE technology relies on a cationic quaternary amine salt emulsifier, such as behentrimonium methosulfate, to form oil‐in‐water emulsion systems with multiple concentrate spheres of oil and water within one another. Hydrophobic and hydrophilic ingredients are trapped within their compatible layers and released over time upon application to the skin [7]. Table 9.1 Formulation components. A basic formulation has many components. Table 9.1 provides an overview of these formula components and also provides a brief summary of the anticipated effect on active delivery. Some of these chemical functions are more clearly defined below in discussion on chemical PEs. The ability of vehicles to deliver actives is tied to an understanding of diffusion of actives through various skin compartments (epidermal and dermal). Diffusion of actives across the skin is a passive process. Compounds with low solubility and affinity for the hydrophilic and lipophilic components of the stratum corneum would theoretically partition at a slow rate. These difficulties may be overcome by adding a chemical adjunct to the delivery system that would promote partitioning into the stratum corneum. Partitioning of actives from the dosage form is highly dependent on the relative solubility of the active in the components of the delivery system and in the stratum corneum. Thus, the formulation of the vehicle may markedly influence the degree of penetration of the active. Percutaneous absorption involves the following sequences: One of the most effective formulation techniques to boost active penetration is supersaturation. This chemical process happens when an active’s maximum concentration in solution is exceeded by the use of solvents or cosolvents. This type of solution state can happen during the evaporation of an emulsion on the skin. As water evaporates from a cream rubbed on the skin a superconcentrate depot of active forms on the skin. This creates a diffusional concentration gradient across the stratum corneum. One can attempt to boost this effect even further in the formulation by slightly exceeding the maximum solubility of the active in the formula using cosolvents. Supersaturation is an effective technique but the disadvantage is that active recrystallization can take place in this highly concentrated solution state. There are crystallization inhibitors that can be added to supersaturated solution but many experimental data need to be collected on this type of formulation strategy. Eutectic blends are formulation techniques that can enhance penetration of actives. The melting point of an active influences solubility and hence skin penetration. According to solution theory, the lower the melting point, the greater the solubility of a material in a given solvent, including skin lipids. The melting point can be lowered by formation of a eutectic mixture. This mixture of two components which, at a certain ratio, inhibits the crystalline process of each other such that the melting point of the two components in the mixture is less than that of each component alone. In all cases, the melting point of the active is depressed to around or below skin temperature thereby enhancing solubility. This technique has been used to enhance the penetration of ibuprofen through the skin [9]. Manipulation of the vehicle skin partition coefficient of a formulation can be used as an overall formulation strategy to boost penetration of actives. This can be done by altering the solubility of the active in the vehicle via selection of different excipients. This change in the solubility parameter (δ) of the excipients can be tuned so that the active is more soluble in the stratum corneum than in the vehicle. Hence the diffusional gradient is altered toward the skin and thereby enhancing penetration. It has been shown that a solvent capable of shifting the solubility parameter (δ) of the skin closer to that of the activate will active flux rate [10]. Another strategy is to add a PE that alters the membrane permeability of the skin. This strategy is discussed in more detail below. Skin occlusion can increase stratum corneum hydration, and hence influence percutaneous absorption by altering partitioning between the surface chemical and the skin because of the increasing presence of water, swelling corneocytes, and possibly altering the intercellular lipid phase organization, also by increasing the skin surface temperature, and increasing blood flow [11]. Different substances penetrate different depth levels in the skin for best results. An effective cosmetic is designed so that active substances get inside the appropriate skin cells, affecting their metabolism in a way that improves their health, yet not damaging overall skin integrity.[12] The ultimate goal of penetration enhancement is to target the active in the stratum corneum and/or epidermis without allowing for systemic absorption. This remains the biggest challenge for active penetration enhancement and it is one of the keys for targeted active delivery. In this section, the influence of PEs on the diffusion coefficient and solubility of the active in the stratum corneum is evaluated. The use of topically applied chemical agents (surfactants, solvents, emollients) is a well‐known technique to modify the stratum corneum and also modify the chemical potential of selected actives. Collectively, these materials can be referred to as PEs. Based on the chemical structure, PEs can be categorized into several groups such as fatty acids, fatty alcohols, terpene fatty acid esters, and pyrrolidone derivatives [13]. PEs commonly used in skin care products have well‐known safety profiles but their ability to enhance penetration of an active is challenging because of the manifold ingredients used in many formulations. They are also known as absorption promoters and accelerants which are “pharmacological inert, nontoxic, nonirritating, nonallergic, rapid onset of action, and suitable duration of action, inexpensive and cosmetically acceptable [14].” A number of solvents (e.g. ethanol, propylene glycol, Transcutol® [Gattefossé, Saint‐Priest, France], and N‐methyl pyrrolidone) increase permeant partitioning into and solubility within the stratum corneum, hence increasing KP in Fick’s equation (equation 9.1). Ethanol was the first PE cosolvent incorporated into transdermal systems [15]. Synergistic effects between enhancers (e.g. Azone® [PI Chemicals, Shanghai, China], fatty acids) and more polar cosolvents (e.g. ethanol, propylene glycol) have also been reported suggesting that the latter facilitates the solubilization of the former within the stratum corneum, thus amplifying the lipid‐modulating effect. Similarly, solvents such as Transcutol are proposed to act by improving solubility within the membrane rather than by increasing diffusion. Another solvent, dimethylsulfoxide (DMSO), by contrast, is relatively aggressive and induces significant structural perturbations such as keratin denaturation and the solubilization of membrane components [16]. Table 9.2 is a list of the more commonly utilized chemical PEs. In addition to the chemical PEs discussed above, there is another class of PEs known as physical PEs. These materials stand between chemical enhancers and PE devices. This unique classification is because in most cases the materials are particles of chemical origin (polyethylene, salt, sugar, and aluminum oxide) but require physical energy to exert an action on the skin. These materials are used to physically débride or excoriate the stratum corneum by abrasive action. This is typically done by rubbing the particles by hand on the skin. New high‐tech devices are now available that propel an abrasive against the skin thereby stripping away the stratum corneum. There are customized carriers (vectors) for delivery of actives to the skin. These vectors are a type of vehicle that allow for enhanced penetration via their small size and unique physical–chemical composition. These vectors are known as submicron delivery systems (SDSs). Discussion focuses on liposomes, niosomes, lipid particles, and nanocapsules. Liposomes are colloidal particles formed as concentric biomolecular layers that are capable of encapsulating actives. The lipid bilayer structure of liposomes mimics the barrier properties of biomembranes, and therefore they offer the potential of examining the behavior of membranes of a known composition. Thus, by altering the lipid composition of the bilayer or the material incorporated, it is possible to establish differences in membrane properties. Liposomes store water‐soluble substances inside like biologic cells. The phospholipids forming these liposomes enhance the penetration of the encapsulated active agents into the stratum corneum [19]. There is debate on liposome formulations and their mode of action regarding penetration enhancement. Variation in performance may be caused by the variation in formulation and method of manufacture used to prepare this delivery form. Several factors such as size, lamellarity (unilamellar vs. multilamellar), lipid composition, charge on the liposomal surface, mode of application, and total lipid concentration have been proven to influence deposition into the deeper skin layers. It is reported by several authors that the high elasticity of liposome vesicles could result in enhanced transport across the skin as compared to vesicles with rigid membranes. Liposomes have a heterogeneous lipid composition with several coexisting domains exhibiting different fluidity characteristics in the bi‐layers. This property can be used to enhance the penetration of entrapped actives into the skin. It is supposed that once in contact with skin, some budding of liposomal membrane might occur. This could cause a mixing of the liposome bi‐layer with intracellular lipids in the stratum corneum which may change the hydration conditions and thereby the structure of lipid lamellae. This may enhance the permeation of the lipophilic active into the stratum corneum and ease the diffusion of hydrophilic actives into the interlamellar spaces [20]. Niosomes are formed by blending nonionic surfactants of the alkyl or dialkyl polyglycerol ether class and cholesterol with subsequent hydration in aqueous media. These vesicles can be prepared using a number of manufacturing processes: ether injection, membrane extrusion, microfluidization, and sonication. Niosomes have an infrastructure consisting of hydrophilic, amphiphilic, and lipophilic moieties together and as a result can accommodate active molecules with a wide range of solubilities. They can be expected to target the active to its desired site of action and/or to control its release [21]. Niosomes are similar to liposomes in that they both have a bi‐layer structure and their final form depends on the method of manufacture. There are structural similarities between niosomes and liposomes but niosomes do not contain phospholipids. This provides niosomes with a better stability profile because of improved oxidative stability. Table 9.2 Commonly utilized chemical penetration enhancers. Solid lipid nanoparticles (SLNP) were developed at the beginning of the 1990s as an alternative carrier system to emulsions, liposomes, and polymeric nanoparticles. SLNP have the advantage of requiring no solvents for production processing and of relatively low cost for the excipients. SLNP represents a particle system that can be produced with an established technique of high‐pressure homogenization allowing production on an industrial scale. This method also protects the incorporated drug against chemical degradation as there is little or no access for water to enter the inner area core of the lipid particle [22]. Lipid particles can be used as PEs of encapsulated actives through the skin because of their excellent occlusive and hydrating properties. SLNP have recently been investigated as carriers for enhanced skin delivery of sunscreens, vitamins A and E, triptolide, and glucocorticoids [23]. Nanocapsules are a type of SDS. This technology can segregate and protect sensitive materials and also control the release of actives. The more obvious opportunity for penetration enhancement of actives is because of their small size (20–1000 nm in diameter). Nanocapsules can be formed by preparing a lipophilic core surrounded by a thin wall of a polymeric material prepared by anionic polymerization of an alkylcyanoacrylate monomer. These very safe types of system have been proposed as vesicular colloidal polymeric drug carriers. Nanocapsules have the ability to enhance penetration but they can also control delivery of actives to the skin. In a recent study, indomethacin was nano‐encapsulated for topical use. This study compared cumulative release of indomethacin dispersed in gel base with indomethacin nano‐encapsulated and indomethacin nano‐encapsulated in a gel. The highest delivery was achieved with the nanoencapsulated indomethacin (Figure 9.2). Devices for enhancing skin penetration of actives are at the leading edge of skincare technology. When utilizing devices for enhanced penetration of actives it is imperative to look into the regulatory classification of these instruments. The FDA has several guidelines and requirements for medical devices (510 K). The 510 K regulatory classification is important for safety and efficacy of any consumer device product and an understanding of the regulatory landscape in this area is essential. Four device technologies are reviewed. They range from moderately invasive to mildly invasive in terms of effect on the skin. In all cases, the goal is to reversibly alter the skin barrier function by physical techniques or electro‐energetic means. Box 9.1 is a list of some methods and tools [18]; and further discussion on the more common skin‐enhancing topics. Ultrasound waves are sound waves that are above the audible limit (>20 kH). During ultrasound treatment, the skin is exposed to mechanical and thermal energy which can alter the skin barrier property. Thermal and nonthermal characteristics of high‐frequency sound waves can enhance the diffusion of topically applied actives. Heating from ultrasound increases the kinetic energy of the molecules in the active and in the cell membrane. These physiologic changes enhance the opportunity for active molecules to diffuse through the stratum corneum to the capillary network in the papillary dermis. The mechanical characteristics of the sound wave also enhance active diffusion by oscillating the cells at high speed, changing the resting potential of the cell membrane, and potentially disrupting the cell membrane of some of the cells in the area [24]. Figure 9.2 Cumulative amount of indomethacin (initial loading 0.5% w/v) per unit area, permeating through excised rat skin when released from PNBCA nanocapsule dispersion in pH 7.4 phosphate buffer, PNBCA nanocapsule dispersion in Pluronic F‐127 gel, and 25% w/w Pluronic F‐127 gel. Each value is the mean ± SE of four determinations. (Source: Miyazaki et al., 2003 [J Pharm Pharmaceut Sci6, 238–45]. Creative Common license (Attribution‐ShareAlike) License.) A recent study on the use of ultrasound and topical skin lightening agents showed the effect of high‐frequency ultrasound together with a gel containing skin‐lightening agents (ascorbyl glucoside and niacinamide) on facial hyperpigmentation in vivo in Japanese women [25]. Delivery patches have been available for some time. One of the first applications of patch technology was in a transdermal motion sickness (scopolamine) patch. There are commercial products that provide actives in a patch formula. They utilize adhesive technology or a rate‐limiting porous membrane to target and localize the actives. Some common patch applications are directed toward reduction of age spots or dark circles under the eye. The key delivery enhancement for patches is a combination of localized delivery and occlusion.
CHAPTER 9
Percutaneous Delivery of Cosmetic Actives to the Skin
Introduction
The basics
Skin physiology
Active composition
Fick’s law
Vehicle effect
Delivery of actives from emulsions
Ingredient
Chemical function
Effect on delivery
Water
Carrier/solvent
Hydration
Alcohol
Carrier/solvent
Fluidizes stratum corneum, alters permeability of stratum cornuem
Propylene glycol
Cosolvent/humectant
Alter permeability of stratum corneum
Alter vehicle stratum corneum partition coefficient
Surfactant
Emulsifier/stabilizer
Emulsion particle size reduction, active solubilizer
Emollient
Skin conditioner, active carrier
Alter stratum corneum permeability
Alter vehicle stratum corneum partition coefficient
Delivery system
Protect/target actives
Targeted/enhanced active penetration
Formulation strategies
Penetration enhancers
Chemical enhancers
Physical enhancers
Penetration enhancement vectors
Liposomes
Niosomes
Chemical penetration enhancer
Description/function
Pyrrolidones
Permeation enhancer for numerous molecules (hydrophilic and lipophilic). They partition well into human stratum corneum within tissue, act by altering the solvent nature of the membrane, and generate reservoirs which offer potential release of a permeant from the stratum corneum over extended time periods [17]
Oxazolidinones
New class of chemical agents that have the ability to localize coadministered active (i.e. retinoic acid) in skin layers, due to their structural features being closely related to sphingosine and ceramide lipids found in the upper skin layers, resulting in low systemic permeation [18]
Urea
Mechanism may be a consequence of both hydrophilic activity and lipid disruption from these biodegradable and nontoxic molecules consisting of a polar parent moiety and a long chain alkyl‐ester group [18]
Azone
Partitions into a bilayer to disrupt their packing arrangement. This nonhomogeneous integration of molecules may be dispersed within the barrier lipoid or separate domains within the bilayer [18]
Fatty acid
Oleic acids are mono‐saturated fatty acids that increase the permeation of lipophilic actives through the skin and buccal mucosa by transdermal cellular pathway [18]
Alcohol, glycol, and glycerides
Low molecular weight alkanols solvents enhance the solubility of the active into the matrix of stratum corneum by disrupting its integrity and contributing to enhancing the mass transfer of biochemicals through this tissue [17]
Alkyl‐n, n‐disubstituted amino acetates
Insoluble in water, but soluble in organic solvents and water–alcohol mixtures with low skin‐irritating potential. Skin penetration is increased by the interaction with stratum corneum keratin which results in increasing the hydrating efficiency [18]
Surfactants
Solubilize lipophilic active ingredients and lipids within the stratum corneum. Anionic and cationic surfactants swell the stratum corneum and interact with intercellular keratin. They have the potential to damage human skin as powerful irritants, increasing transepidermal water loss of human skin [18]
Essential oil, terpenes, and terpenoids
Operational mechanism modifies the solvent nature of the stratum corneum thereby improving the active partitioning into the tissue. Terpenes and terpenoids are constituents of volatile oils that are considered to be less toxic with low irritancy when compared to surfactants and other synthetic skin penetration enhancers
Cineole
Natural organic compound (eucalyptol) that promotes the percutaneous absorption of lipophilic actives [18]
Eugenol
Enhances the permeability coefficient of the active with lipid extraction and improvement in the partitioning of the active to the stratum corneum. Slightly soluble in water and soluble in organic solvents. An allylbenzene class of chemical compound (extract of clove oil, nutmeg, cinnamon, and bay leaf) that reduces ability to feel and react to painful stimulation
Farnesol
Sesquiterpene alcohol (citronella, neroli, lemon grass, tuberose, balsam, and tolu) enhances the permeation of diclofenac sodium with its use in perfumery products
Menthol
Highly effective penetration enhancer used for transdermal delivery of variety of actives and shown to increase the effect when paired with iontophoresis [17]. Used in medical and cosmetic preparations enhancing absorption of desirable molecules to the internal layers of the skin while also functioning as an effective counter‐irritant [12]
Solid lipid nanoparticles
Nanocapsules
Devices for penetration enhancement
Ultrasound waves
Patches
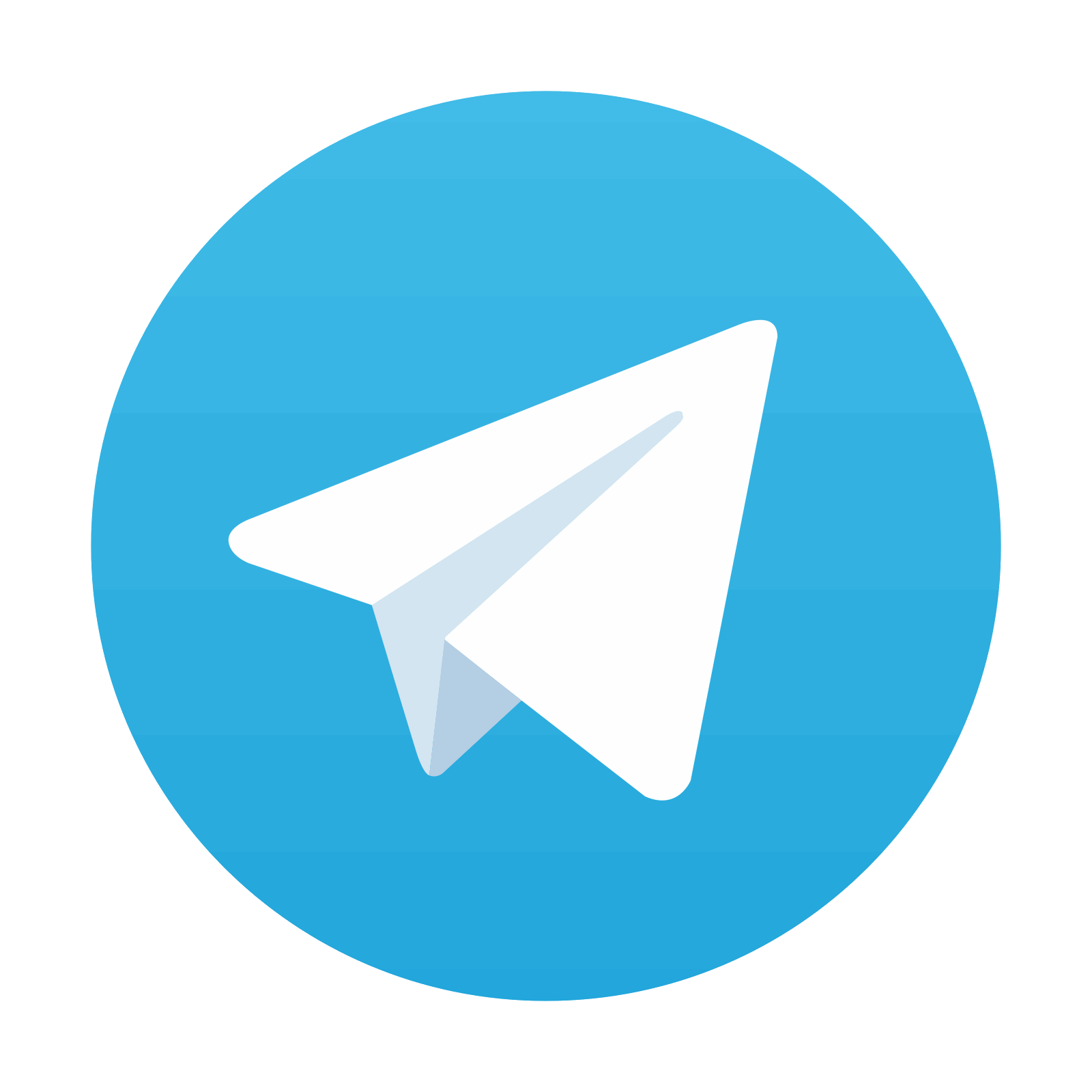
Stay updated, free articles. Join our Telegram channel

Full access? Get Clinical Tree
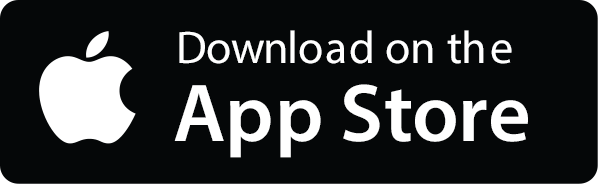
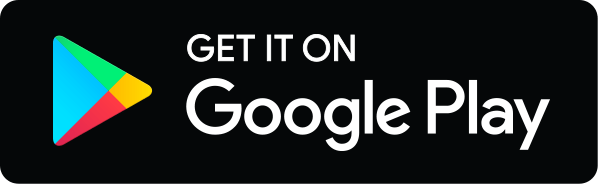