Jacob Beer1, Soraya Azzawi2, Kenneth R. Beer2,3, and Aigen Alyx Rosen2 1 Department of Dermatology, University of Pennsylvania, Pennsylvania, PA, USA 2 Dr. Phillip Frost Department of Dermatology and Cutaneous Surgery, University of Miami Miller School of Medicine, Miami, FL, USA 3 Surgical and Aesthetic Dermatology, West Palm Beach, FL, USA Nonsurgical cosmetic procedures are increasingly becoming a staple of dermatologic and plastic surgery practices around the country. From 2010 to 2019, the number of nonsurgical cosmetic procedures performed in the United States has skyrocketed by 40.5%, representing a total of over 16 million procedures in the year 2019 alone [1, 2]. One of the most common among such treatments is polylactic acid (PLLA) fillers. These injectable soft‐tissue augmentation agents were initially approved in 2004 [3] to address lipodystrophy due to adverse effects of HIV antiretroviral therapy. Since then, the utility of PLLA in rejuvenation of face and body contours has been increasingly recognized, revolutionizing the fields of cosmetic dermatology and reconstructive surgery. PLLA affords patients two significant advantages compared with other options: it is a gradual transition to increased volume and it stimulates the body to produce its own collagen and elastin fibers which many patients find preferable. Given the sharp rise in demand, it behooves physicians to become familiar with the utility, administration techniques and complications associated with PLLA fillers. Aging is an elaborate process interweaving environmental factors, including stress, smoking and sun exposure (extrinsic aging), with the natural degeneration of senescent cutaneous, connective, and osseous tissue (intrinsic aging) [4–8]. The biomechanical properties of the skin are derived from the epidermal architecture. This architecture includes the dermal fibrillar collagen matrix and elastin network, as well as a host of subcutaneous structures [7, 9]. Chronologic aging induces damage to these tissues that is similar to impairment triggered by sun exposure [7, 10, 11]. These two mechanisms act synergistically to promote disorganization of dermal fibrillar collagen, the key protein involved in cutaneous structural support and organization [12]. Upregulation of matrix metalloproteinases in the skin leads to collagen degeneration [13, 14], and by extension, dermal atrophy. In addition to destruction of the collagen matrix, the ability to synthesize new collagen is compromised. Fibroblasts, the primary cells responsible for collagen production, are severely reduced in damaged skin [7,14–17]. Furthermore, progressive weakening of the collagen matrix through either chronologic or photoaging renders it incapable of providing the requisite mechanical tension to sustain fibroblast neocollagenesis [16]. Another target of natural and photoaging include the dermal elastin network. Deposition of aberrant elastin undermines the skin’s capacity for elastic recoil in response to mechanical deformation [12]. In combination, these processes are reflected as cutaneous laxity and coarse wrinkles [4]. Chronologic aging leads to prominent changes in both fat distribution and volume [18, 19]. Fat pad compartments are found in the superficial layer, beneath the dermis and above the fascia superficialis, and a deeper layer beneath the musculature [20]. These fat compartments work in concert with musculature and ligaments to generate natural facial contours. During the aging process, the perioral, buccal, periorbital, temporal, and forehead areas undergo fat atrophy along with laxity of the tendons [21]; By contrast, hypertrophy of the fat pads occurs in the jowl, lateral nasolabial fold, lateral labiomental crease, and lateral malar areas [21]. In addition to the arrangement of subcutaneous fat, retromuscular convexity also plays a key role in the perception of a healthy, youthful appearance. Unique from every other muscle group in the human body, youthful facial muscles demonstrate a broad convex conformation. When subjected to chronological aging, deep fat is displaced inferiorly, leading the convex contour of the overlying muscle to transform increasingly rectilinear [22]. This evolution of fat distribution and muscle contouring unmasks bony protuberances [23], distorts natural boundaries between anatomic areas, and dramatically alters the perception of the face. Osseous resorption and remodeling profoundly alter facial dimensions in chronologic aging [24, 25]. At approximately age 40 in both men and women, the compact bone diminution commences at a rate of 3% every 10 years [26], resulting in loss of craniofacial support to soft tissue. According to Lambros’s theory, the conceptual foundation informing modern aesthetic surgical approaches, age‐related changes of the periorbital and midfacial bone are characterized as a clockwise rotation of the midface relative to the cranial base [27]. This remodeling involves an increase in the orbital aperture [28, 29], and decreased glabellar, pyriform, and maxillary angles [24, 28]. Loss of the maxilla accentuates the nasolabial fold [30]. Increase in mandibular angle erodes jawline definition, resulting in a blunted, ovoid contour of the lower face [31]. Reduced mandibular volume both compromises structural support for the lower face, leading to perioral wrinkling [5], as well as increases laxity of the soft tissue in the neck [31]. Changes in the maxilla and mandible work in tandem to reduce facial height [5]. The dynamic process of chronologic aging therefore combines craniofacial volume loss and remodeling, fat redistribution and changes in skin biomechanical properties, all of which alter facial topography. Reflecting on the aforementioned changes, patients often seek correction of skin laxity, fat redistribution, and reduced elasticity. Comprehensive evaluation of all underlying factors is critical to providing optimal, natural‐looking cosmesis and selecting appropriate therapeutic options. To date, there is a dearth of empirical data to guide clinicians and researchers in assessing the severity of skin damage related to aging and sun exposure [32]. Although three‐dimensional imaging and digital photographs allow for surveillance of surface shape and volume measurements, there remains no consensus regarding classification of structural changes. Several approaches can enable a more objective assessment of facial aging, including numerous grading scales [33–36]. The Fitzpatrick–Goldman’s nine‐point classification [33], Glogau’s four‐grade classification [37], Lemperle’s six‐grade classification [36], and Rao–Goldman facial wrinkling scale [38] provide standardized methods of analyzing generalized facial change. Grading systems tailor‐made to certain areas of interest have also been validated, including the forehead [39], nasolabial folds [40–42], and periorbital [43] regions. The wrinkle severity rating scale (WSRS) is a validated method for quantifying the severity of nasolabial folds with excellent intra‐ and inter‐observer reliability [40]. Fewer resources exist for objective analysis of deep wrinkles of the body. The Fabi–Bolton five‐point chest wrinkle scale is one of the few such instruments for analysis of chest fold wrinkles [44]. To evaluate upper extremity contour deformities, the Brachial Ptosis scale examines volume of adipose tissue deposition and extent of associated ptosis [45]. The commercial formulation of PLLA (Sculptra, Galderma (Dallas, TX)) is a biocompatible synthetic polymer of lactic acid. Injectable PLLA is a lyophilized powder consisting of microparticles mixed with the excipients sodium carboxymethyl cellulose and mannitol. This formulation requires reconstitution with sterile water to form a suspension [46–49]. Through its affinity for water, sodium carboxymethyl cellulose promotes uniform distribution of PLLA microparticles [47, 50]. As a bulk agent, mannitol acts to improve solubility upon reconstitution [47, 50]. The sodium carboxymethyl cellulose and mannitol act to stabilize the PLLA and have no biologic effect on the volume stimulation. Each microparticle has a diameter between 40 and 63 μm, a deliberate range that is meticulously selected [49, 51, 52]. At this size, PLLA microparticles are massive enough to evade macrophage‐mediated phagocytosis and diffusion through vessel walls, enabling the microparticles to exert their effects locally [49, 51, 52]. A unique property of PLLA in comparison to other injectable soft‐tissue augmentation agents is its biostimulatory nature [52–55]. While collagen‐based and hyaluronic acid (HA) injectable agents for the most part act as transient, space‐occupying fillers, PLLA stimulates neocollagenesis, leading to gradual volume correction over time. By addressing the mechanisms fundamental to chronologic aging, biostimulatory products like PLLA thereby offer a more enduring solution to volume loss [56, 57]. When injected into the dermal or subcutaneous planes, PLLA microparticles directly stimulate a subclinical local inflammatory reaction. This foreign body response encapsulates PLLA microparticles and induces secondary fibroplasia [53, 58, 59]. Fibroblasts then produce autologous type I collagen deposition, reinforcing the extracellular matrix and eventually restoring facial volume loss [46, 49, 54, 60]. The histologic sequence of events following subcutaneous PLLA injection has been examined extensively in murine models [54]. PLLA was found to be biocompatible, with no abscess formation, acute inflammation, or tissue necrosis noted in surrounding tissues. In the first month after injection, a mixed inflammatory infiltrate of lymphocytes, mast cells, and mononuclear macrophages encircle the PLLA particles. Collagen deposition is observed at approximately 3 months postinjection. Neocollagenesis continues through 6 months postinjection, along with a gradual reduction in the inflammatory infiltrate. In human histologic studies, endogenous collagen deposition has been observed to develop over a time span of 8–30 months [49, 53, 61]. Over 9 to 24 months, PLLA is degraded through nonenzymatic hydrolysis into water and carbon hydroxide and eliminated from the body [61–64]. At 9 months postinjection into murine models, no residual polymer or remnant cicatricial fibrosis was observed histologically [60]. Despite degradation of PLLA microparticles, the newly synthesized collagen and augmented volume can be sustained for up to 2–3 years [65–72]. PLLA’s unique biochemical properties set it apart from other surgical and nonsurgical treatments for rejuvenation. While surgical options may improve cutaneous laxity, they do not restore lost volume and results therefore often lack natural proportions. Permanent implants augment volume but are unable to adapt to dynamic processes of chronologic aging [73]. In contrast to these alternatives, PLLA acts as a semi‐permanent filler. The US Food and Drug Administration has currently approved PLLA for two major indications: correction of lipoatrophy in patients with human immunodeficiency virus and correction of age‐related facial wrinkles and contour deficiencies [74]. Injectable PLLA is contraindicated in patients with any history of hypersensitivity to PLLA or its components. Patients with a predisposition for hypertrophic or keloid scarring should also avoid PLLA injections. The ultimate clinical results achieved by PLLA injections are contingent on numerous factors, many of which precede the injection itself. Candidate selection, dilution ratios, and reconstitution time must be carefully accounted for. Given that treatment response tends to wane with increasing age, optimal outcomes with PLLA injections have been observed with patients under 60 years of age. Although the amount of PLLA in a vial of Sculptra is fixed at 367.5 mg [74], the methods of reconstitution and thus the volumes used for injections are variable. The ability to add different amounts of water, lidocaine, and epinephrine is one opportunity for physicians to vary the use of this material and may contribute to differing reports of success and complications. Unlike other filling agents, the product injected is not standardized and the package insert does not reflect the way that the product is presently used. Initial reports of product use in Europe utilized dilutions of 2 mL of sterile water per vial [61]; this led to unacceptably high incidences of complications. In these preliminary investigations, PLLA injections were complicated by papulonodule formation at rates of 6–44% [75]. These rates notably dropped in the seminal PLLA randomized study, when the reconstitution volume used was increased to 5 mL [66]. Postinjection papules occurred at a rate of 8.6%, while the incidence of nodules was 6.9% [66]. The majority of these complications are self‐resolved. This reduced rate of complications was also appreciated at 25 months. Practitioners are increasingly appreciating the utility of dilution volumes in diminishing rates of adverse events related to PLLA injections. As people have gained more experience, many have adapted more dilute injections. These are typically in the 9 mL diluents/bottle for face but may be as high as 15 mL per bottle when working on areas other than the face. There is very little data on what the optimal dilution and timing for injections is and it is hoped that these trials will provide better insights for both patient and physician. In addition to sterile water, some practitioners elect to also dilute PLLA with an injectable anesthetic such as lidocaine either with or without epinephrine. To optimize efficacy of the epinephrine, the anesthetic should be introduced near the time of injection [3]. Volumes of anesthetic added to the reconstituted material vary based on the location of each injection and the experience of the injector. At the present time, most injectors recommend reconstituting with dilution volumes of 8–12 mL water for at least 24 hours, and then adding 1–2 mL of 1% lidocaine with 1:100,000 epinephrine immediately before injection [3]. When injecting the dorsal hands, for example, a common practice is utilizing a total reconstitution volume of 15 mL; of this volume, 14 mL consists of water mixed with an additional 1 mL 1% lidocaine with 1:100,000 epinephrine. By contrast, many practitioners opt for dilutions exceeding 10 mL per vial when treating the chest, arms, and knees. With regards to reconstitution time, the protocol delineated in the PLLA trial recommended adding 5 mL of sterile water to the PLLA freeze‐dried powder, permitting 2 hours for hydration and then agitating the vial prior to use [66, 76]. However, a recent study exploring immediate use of PLLA after hydration found no difference in physiochemical properties between 0, 2, 24, and 72 hours of standing time, suggesting that the PLLA product can be used without prolonged reconstitution time [47]. Despite these early suggestion algorithms, many injectors add 9 mL of water and allow the product to imbibe for at least 24 hours and typically at least 48 hours. Prior to injection, many injectors prefer to allow the product to return to room temperature. Given that dilution ratios and reconstitution times are under active investigation, there are currently no evidence‐based guidelines that conclusively determine ideal parameters. Appropriate injection technique involves intimate knowledge of anatomy and danger zones, depth of injection [77], administration technique, conservative amount of product, treatment intervals of at least 4 weeks, and consistent reassessment. The most common problem encountered is clogging of material in the needle, resulting in sporadic injections of product under high pressure and placement of the product at the wrong plane. Rapid injection is the best technique for injecting PLLA. This avoids needle clogs thus reducing high pressure injections and areas of high and low concentration of product placement. Injection needles should be either 25 or 26 gauge and 0.5–1 inch in length. Smaller bore and longer needles may result in difficulty extruding the product into the tissue. However, the advantages of longer needles are that they can get to a wide area with fewer injection sites and less theoretical trauma. Cannulas may also be used for the insertion of PLLA but should be 25 gauge to avoid clogging. One advantage of cannulas is that they enable the injector to deposit PLLA across a wide area of the face with far fewer punctures than a traditional needle. Although injections of PLLA require deep product placement to minimize papule formation and irregular texture, a review of the literature highlights the variability in practice. Some practitioners recommend needle should be angled at 30–40° and inserted at the level of the deep dermis or dermal–subcutaneous junction [73]. Alternatively, others recommend injection into the subcutaneous fat [78]. Prior to actually injecting, it is critical to draw back on the syringe to confirm that the needle has not punctured vasculature. If blood is noted, the syringe should be completely retracted, disposed of in the appropriate sharps container and a new needle reinserted [73] (Figure 48.1). Numerous PLLA injection techniques have been described, including serial puncture, linear threading, radial fanning, and cross‐hatching. Depot injections may be an attractive option for deeper areas. Serial puncture, as the name suggests, delivers product sequentially along the length of a vector; multiple punctures increases the risk of post‐procedural bruising. The linear threading approach introduces the full length of the needle or cannula into the area being targeted for rejuvenation; PLLA is then deposited while the instrument is being retracted, creating “tunnels” or “channels” that the product fills. The cross‐hatching method, excellent for treating large areas, is essentially sequential linear‐threading. After completing one round of linear threading, a second group of injection lines is then arranged perpendicularly to the first series, creating a hash appearance overall. The exact method utilized is often governed by the anatomical region being injected and the volume of product used.
CHAPTER 48
Polylactic Acid Fillers
Introduction
Biomechanical dynamics of aging
Skin
Subcutaneous fat and musculature
Bone
Assessment of candidates for polylactic acid filler therapy
Composition of polylactic acid fillers
Mechanism of action of polylactic acid fillers
Indications and contraindications
Pretreatment considerations
Injection techniques (Table 48.1)
Stay updated, free articles. Join our Telegram channel

Full access? Get Clinical Tree
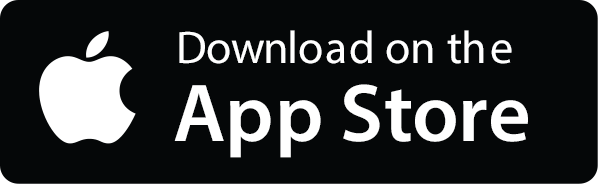
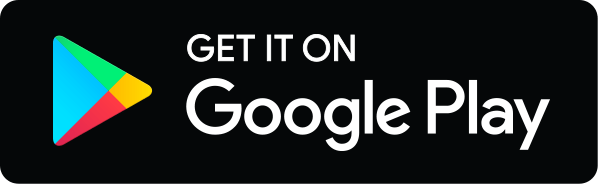