Vaccine
Name
Manufacturer
Type
Route
Anthrax
BioThrax
BioPort
Inactivated bacterial
SC
DTaP
Daptacel
Sanofi
Inactivated bacterial
IM
Infanrix
GSK
Inactivated bacterial
IM
Tripedia
Sanofi
Inactivated bacterial
IM
DT
Generic
Sanofi
Inactivated bacterial toxoids
IM
DTaP/HiB
TriHIBit
Sanofi
Inactivated bacterial
IM
DTaP/IPV/HiB
Pediarix
GSK
Inactivated bacterial and viral
IM
Haemophilus influenzae type b (Hib)
HibTITER
Wyeth
Inactivated bacterial
IM
PedvaxHIB
Merck
Inactivated bacterial
IM
ActHIB
Sanofi
Inactivated bacterial
IM
Hepatitis A
Havrix
GSK
Inactivated viral
IM
Vaqta
Merck
Inactivated viral
IM
Hepatitis B
Engerix-B
GSK
Inactivated viral (recombinant)
IM
Recombivax HB
Merck
Inactivated viral (recombinant)
IM
HepA/HepB
Twinrix
GSK
Inactivated viral
IM
HPV
Gardasil
Merck
Inactivated viral (recombinant)
IM
HepB/Hib
Comvax
Merck
Inactivated bacterial and viral
IM
Influenza
Fluarix
GSK
Inactivated viral
IM
Fluvirin
Chiron
Inactivated viral
IM
Fluzone
Sanofi
Inactivated viral
IM
FluMist
Medimmune
Live attenuated viral
Intranasal
Japanese encephalitis
JE-Vax
Sanofi
Inactivated viral
SC
MMR
M-M-R II
Merck
Live attenuated viral
SC
MMRV
ProQuad
Merck
Live attenuated viral
SC
Measles
Attenuvax
Merck
Live attenuated viral
SC
Mumps
Mumpsvax
Merck
Live attenuated viral
SC
Rubella
Meruvax II
Merck
Live attenuated viral
SC
Meningococcal
Menomune
Sanofi
Inactivated bacterial
SC
Menactra
Sanofi
Inactivated bacterial
IM
Pneumococcal
Pneumovax 23
Merck
Inactivated bacterial
SC or IM
Prevnar
Wyeth
Inactivated bacterial
IM
Polio
Ipol
Sanofi
Inactivated viral
SC or IM
Rabies
BioRab
BioPort
Inactivated Viral
IM
Imovax Rabies
Sanofi
Inactivated Viral
IM
RabAvert
Chiron
Inactivated Viral
IM
Rotavirus
RotaTeq
Merck
Live viral
Oral
Td (tetanus & diphtheria)
Decavac
Sanofi
Inactivated bacterial toxoids
IM
(Generic)
Massachusetts Biological Labs
Inactivated bacterial toxoids
IM
Tdap (tetanus & diphtheria toxoids/pertussis vaccine
Boostrix
GSK
Inactivated bacterial
IM
Adacel
Sanofi
Inactivated bacterial
IM
TT (tetanus toxoid)
(Generic)
Sanofi
Inactivated bacterial toxoid
IM
Typhoid
Typhim Vi
Sanofi
Inactivated bacterial
IM
Vivotif Berna
Sanofi
Live bacterial
IM
Varicella
Varivax
Merck
Live viral
SC
Vaccinia (smallpox)
Dryvax
Wyeth
Live viral
Percutaneous
Yellow fever
YF-Vax
Sanofi
Live viral
SC
Zoster (shingles)
Zostavax
Merck
Live viral
SC
Control of Infection
Extracellular Infection
Cytokines secreted by CD4+ T-helper-1 (Th1) T cells aid in activating phagocytic cells such as macrophages and thus help in the destruction of the foreign agent in this fashion or complexed with an antibody.
Intracellular Infection
With intracellular infections with viruses, cytotoxic T lymphocytes (CTLs) play a dominant role in controlling and clearing the infection. In addition to its active role in killing infected cells, cytotoxic T cells secrete potent cytokines with antiviral and macrophage-activating capabilities, such as interferon-γ (IFN-γ) and tumor necrosis factor-α (TNF-α) [1]. With intracellular bacterial infections, the immune response is not as straightforward. Both CD4+ Th1 and CD8+ T cells have an important role in reducing, controlling, and clearing the infection [4–7].
Regional Immunity
The mucosal surfaces of the human body are far greater in area in comparison to the skin and are well endowed with draining lymphoid tissues, with the exception of the vagina. The main routes of infection are the gut, the rectum, the genitourinary tract, the respiratory tract, and the eye. There is a common mucosal system, so that immunization at one site can result in protection at that and other mucosal areas. For instance, the adenovirus vaccine is administered orally but offers protection against a respiratory infection [8]. In addition, since the female genital tract is devoid of lymph tissue, systemic immunization is necessary to confer protection. Specifically, immunization through the respiratory tract was shown to be superior to other routes in inducing protection in the female genital tract [7, 9–11].
The formation and secretion of secretory immunoglobulin A (sIgA), is the first line of defense in the mucosal immune system; sIgA binds to antigens and their toxins and subsequently aids in prevention of attachment and penetration of the mucosal surface. Cytotoxic T cells have also been shown in the cytobrush specimens from the cervix of HIV-infected women [12]. Thus, protection in mucosal immunity is likely mediated through humoral and cell-mediated mechanisms.
Immunologic Memory
Most organisms are detected and destroyed within hours by defense mechanisms that are not antigen-specific and do not require any prolonged period of induction. This illustrates the mechanism of innate immunity. Only when a pathogen or antigen is able to break this early line of defense will an adaptive immune response ensue, including the production of antigen-specific effector cells, secretion of antibodies by B cells, direct cytotoxic activity (CTLs), or via the secretion of immunologic mediators and effector molecules such as cytokines and chemokines. Most of the effector cells will die within 10–14 days after infection, but some cells survive as highly reactive plasma cells (B cells) or memory cells (B and T cells) to combat subsequent infection. The goal in preventative vaccination is to stimulate the adaptive response in the body with formation of long-lasting antibodies against a specific pathogen and induction of memory cells. The mechanism of how it should be achieved through immunization is less certain, but significant information has been elucidated about immunologic memory thus far [13–15].
B-Cell Memory
A memory B-cell is one that has undergone immunoglobulin isotype switching and somatic hypermutation [16]. This differentiation process starts late in the primary immune response and takes place in the germinal centers of lymph nodes. Upon reencountering the same pathogen (or antigen in a booster vaccine), the memory cells start dividing at a high rate and differentiate into antibody-secreting plasma cells. Further, memory cells produce antibody of higher average affinity than unprimed B cells; the affinity of that antibody persists to increase during the ongoing secondary and subsequent antibody responses.
T-Cell Memory
After immunization, the number of T cells reactive to a given antigen increases markedly as the majority become effector T cells; then the number falls back to continue at a level significantly (100- to 1000-fold) above the initial frequency. These cells carry cell-surface proteins more characteristic of effector cells than of naive T cells. Both CD4+ and CD8+ T cells can differentiate into two types of memory cells. One type is called effector memory cells because they can quickly mature into effector T cells and secrete large amounts of cytokines such as IFN-γ, interleukin-4 (IL-4), and IL-5 early after re-stimulation. They probably develop from effector T cells and are relatively short-lived [17]. The other type is called central memory cells, and they are long-lived in the absence of antigen and are able to deal with systemic pathogenic infections. Recently, a new type of T cell, the T memory stem cell, has been recognized in the CD8+ which has the ability to reproduce itself as well as give rise to effector memory and central memory cells. These cells have been shown to play a significant role in immune homeostasis and have been a target in recent therapies targeting intracellular pathogens and cancer [18].
Routes of Vaccination
Most vaccines are currently given by injection, which has two main disadvantages. The first is that injections are painful and expensive, requiring needles, syringes, and a trained injector. Mass vaccination can be arduous through this route. The disadvantage from an immunologic standpoint is that the injection may not be the most effective way of stimulating an appropriate immune response since it is not imitating the route of the majority of the pathogens.
Although the presentation of soluble protein antigens by the oral route often results in tolerance, which is necessary with the large load of food-borne and airborne antigens presented to the gut and respiratory tract, many pathogens that cause significant morbidity and mortality across the world infect mucosal surfaces or enter the body through mucous surfaces. For this reason, several vaccines targeting the mucosal immune response have been and are currently being studied. For instance, there is the Sabin trivalent oral poliovirus vaccine (OPV). Unfortunately due to one case of paralysis, the Centers for Disease Control (CDC) and the American Academy of Pediatrics have recommended that only inactivated polio vaccine (IPV) be used after January 1, 2000 [19]. Oral polio vaccines are still being used in areas outside the United States where polio is endemic [20]. In addition, a safe and effective intranasal influenza vaccine has been available for several years. Buccal, sublingual, vaginal, and eye mucosa methods of vaccination have recently been studied in preclinical investigations and have shown promising results [21, 22].
Due to the significant advances in the technology of immunization, other routes of delivery are being considered. For instance, viral and bacterial antigens have been produced in transgenic plants [23, 24]. Plant-based vaccines offer a means to deliver large quantities of a designated antigen in an encapsulated form. This encapsulation appears to protect against rapid and complete degradation of orally administered recombinant proteins. Thus, there is the potential for antigen to be gradually released into the gastrointestinal tract as host tissue is digested. This should theoretically permit an increased proportion of orally administered antigens to reach the effector sites, such as Peyer’s patches lining the gastrointestinal tract [25]. A few human vaccine candidates have entered or completed phase I clinical trials. These include diarrheal vaccine candidates targeting enterotoxigenic Escherichia coli [26, 27] and Norwalk virus [28] and candidates against hepatitis B, rabies, smallpox, RSV, diphtheria, influenza, and anthrax [29–31].
Another route being considered is epicutaneous immunization. In the past few years, it has been elucidated that application of antigen onto bare skin induces potent systemic and mucosal immunity in an antigen-specific manner via Langerhans and dendritic cells [32–34]. Coadministration of nonspecific adjuvants, such as cholera toxin, is necessary to induce good immune responses. It has recently been demonstrated that a natural adjuvant effect can be achieved by disrupting the stratum corneum prior to topical antigen application; this act stimulates the Langerhans cells [32]. Epicutaneous immunization also generates active antigen-specific immunity in the gut and particularly augments Th2 responses subsequent to oral antigen [35] and inhalation of antigen [36]. The search for methods of vaccine delivery not requiring a needle and syringe has been influenced by concerns of pandemic disease, bioterrorism, and disease eradication campaigns [37]. Early testing of skin-patch tests is beginning, and past data with epicutaneous influenza vaccine showed that the vaccine was well tolerated by human volunteers [38].
Vaccine Development
Live-Attenuated Vaccines
Viruses
Live-attenuated viral vaccines are composed of viruses that are traditionally grown in cultured cells. Viruses are typically selected for preferential growth in nonhuman cells, and, in the course of selection, become less capable of growth in human cells. Since these attenuated strains do not replicate well in human hosts, they induce immunity but not disease when given to individuals. There may be a slight possibility that the pathogenic virus can reemerge by a further series of mutations even though attenuated virus strains contain multiple mutations in their genome. Further, attenuated viral vaccines can cause significant damage by inducing viral opportunistic infections in those that are immunodeficient [39]. Live-attenuated viral vaccines are currently in use for smallpox, measles, mumps, rubella, varicella, herpes zoster, polio, influenza (intranasal), yellow fever, and rotavirus. The vaccines targeting diseases that cause cutaneous manifestations are discussed in this section and throughout the chapter.
Smallpox
Edward Jenner, in 1796, was the first to demonstrate that inoculation of cowpox virus into human skin could lead to protection from subsequent smallpox infection [40]. Jenner named the inoculation substance vaccine, based on the Latin word vacca, meaning “cow.” The vaccines used for smallpox vaccination are derived from vaccinia virus, a species similar to cowpox. The virus that causes smallpox is the variola virus, which belongs to the Poxviridae family and Orthopoxvirus genus, which also includes the vaccinia, cowpox, and monkeypox viruses. The smallpox vaccine, consisting of several types of strains of the live attenuated vaccinia virus, has served as the prototype of success of a viral vaccine; it was employed in the eradication of the disease. Prior to immunization, smallpox infection killed hundreds of millions of people unremittingly. The eradication of smallpox has been viewed as one of the greatest achievements in medicine.
Approximately two decades ago, after the complete elimination of smallpox, vaccine production ceased [41]. However, due to the recent concerns about biologic warfare and the use of smallpox as an agent, the danger of this disease has not been eliminated. Thus, renewed interest has been developed in production of the smallpox vaccines. ACAM2000 (Acambis) is the currently licensed vaccine used for immunization against smallpox in public health, health-care-response teams, and laboratory workers involved with vaccinia virus research. It is derived from the formerly used vaccine Dryvax, with the main difference being vaccine preparation; ACAM2000 is prepared in cell culture rather than calf lymph, which gives it a lesser risk of causing rare but serious complications [42].
Contraindications to the use of this vaccine in the absence of circulating smallpox include allergies to polymyxin B sulfate, streptomycin sulfate, chlortetracycline hydrochloride, and neomycin sulfate. Patients in an immunocompromised state or women who are pregnant or intend to become pregnant or who are breastfeeding should also not receive the vaccine. The vaccine should be administered concurrently with antihistamines or glucocorticoids to individuals who have allergic symptoms to the above compounds and have contact with individuals with smallpox. Further, the use of the smallpox vaccine is cautioned in persons with a history of eczema or atopic dermatitis; in persons who have acute, chronic, or exfoliative skin conditions; in persons who are using topical ocular steroid medications; and in persons who are younger than 18 years of age [43].
Measles, Mumps, and Rubella
Vaccination for the three classic childhood diseases of measles, mumps, and rubella (MMR) includes live attenuated viruses, and was introduced in the 1960s. The annual reported cases of these infections have declined by more than 98 % in the United States and Europe [44]. This decrease in incidence is largely due to the recommendation that all states require a two-dose MMR vaccination prior to children entering school. The two-dose MMR vaccine is advocated to induce immunity in the small percentage of individuals who do not respond to one or more components of the first dose. The most updated recommendations from the CDC are vaccination with the first MMR dose at 12–15 months and the second dose at 4–6 years of age [45]. Table 49.2 summarizes the childhood immunization schedule for MMR, as well as other vaccines described in this chapter [46]. Immunization evokes a mild subclinical infection that is noncommunicable.
Table 49.2
Childhood immunization schedule for diseases with cutaneous manifestations
Dose Vaccine | Dose 1 | Dose 2 | Dose 3 | Dose 4 | Dose 5 | Boostert |
---|---|---|---|---|---|---|
Hepatitis B | Birth | 1–2 months of age | 6–18 months | X | X | X |
Hib | 2 months | 4 months | 12–15 months | X | X | X |
MMR | 12–15 months | 4–6 years | X | X | X | X |
Pneumococcal (PCV) | 2 months | 4 months | 6 months | 12–15 months | 24–59 monthsa | X |
Varicella | 12–15 months | 4–6 years | X | X | X | X |
Meningococcal | Single doseb | X | X | X | X | X |
Hepatitis A | 2 doses between 12 and 23 monthsc | X | X | X | X | |
Human papillomavirus | Total of 3 dosesd |
Since the vaccines are composed of live attenuated viruses, they are not recommended for pregnant women or those planning to become pregnant within the next 3 months. The primary concern with pregnant women has been the risk of eliciting the congenital rubella syndrome (CRS). However, a study of 321 women who received the vaccine 3 months prior to or after conception demonstrated no congenital malformations compatible with congenital rubella infection [47]. Another contraindication is a history of anaphylactic hypersensitivity to neomycin. Persons with asymptomatic HIV infection or with mild immunosuppression can get vaccinated with MMR. Further, healthy persons with minor illnesses with or without fever, and those with an allergy to eggs can receive the vaccine as well. The risk of severe anaphylactic shock reaction is exceedingly low in individuals with a history of allergy to eggs [45].
The recommendation is to observe these patients for up to 90 minutes after immunization [48]. Also, despite suggestions that the MMR vaccine has a reported association to autism, studies have proved strong evidence against that hypothesis [49–53].
Measles
The measles virus is very efficient in transmission, and has been noted to be the most infectious disease of humankind in regard to the minimal number of virions required to elicit infection [54]. Since humans are the only reservoirs for the measles virus, global eradication is a realistic goal. In the U.S., measles is no longer considered an indigenous disease due to universal childhood immunization programs [55], and the reported incidence of measles has decreased by >99 % since the measles vaccine has become available until a recent surge in reported cases since 2013 owing largely to travelers from abroad and outbreaks among children whose parents refused immunization. Data from the CDC regarding 2014 demonstrated 644 confirmed cases, which exceeded the previous highest annual total number of cases since 2000 [56]. Unfortunately, however, the current rapid spread of measles among unvaccinated persons in the U.S. resulted in another large outbreak in 2015.
Subsequent to receiving two doses of the vaccine, 95–99 % of individuals develop serologic evidence of immunity to measles [57, 58]. Immunity is viewed as lifelong, and comparable to an acquired infection with the wild-type virus [59]. Rare cases of measles infection have been reported in patients with previously documented postimmunization seroconversion [60, 61].
Adverse effects after measles vaccination are usually mild, and include fever (5–15 %) [62], transient viral exanthems [44], and less commonly encephalitis or encephalopathy (less than one per one million vaccines) [63]. Further, a small number of cases have been described of the occurrence of subacute sclerosing panencephalitis (SSPE) in individuals with a history of vaccination but no known history of infection [64–66]. A more detailed review of these cases demonstrated that some cases had unrecognized natural measles infection prior to vaccination, and the SSPE was directly correlated to the infection [45]. Widespread measles immunization has practically eliminated SSPE in the U.S., and the live measles vaccine does not increase the risk of this adverse event [45].
Mumps
A live attenuated mumps vaccine (Jeryl-Lynn strain) was introduced in 1967 and is prepared in a chick embryo cell culture. Immunization evokes a mild subclinical infection that is noncommunicable. Early clinical efficacy studies have shown that 97 % of children and 93 % of adults develop serologic evidence of immunity after vaccination [67–69] and serologic and epidemiologic evidence suggests that immunity persists for at least 30 years after immunization [70–73].
Rubella
The RA 27/3 (rubella abortus 27, explant 3) rubella vaccine is grown in human diploid fibroblast cell culture. It induces a response in more than 97 % of recipients [54, 68]. Immunity in vaccinated individuals is believed to be lifelong and has been demonstrated to persist for at least 16 years [76, 77].
Adverse events following rubella vaccination include fever, lymphadenopathy, and viral exanthemas, usually between 5 and 12 days after vaccination [74, 78]. Arthralgias and arthritis occur more frequently with adult vaccinees, especially women, ranging from 25 to 40 % in this population [79–81].Although no longer common, failure to develop 50–60 % of immunity to rubella by vaccination leaves women of childbearing age susceptible to developing rubella infection during pregnancy. This causes congenital rubella in children born of mothers who contract rubella during early pregnancy.
Varicella-Zoster Virus
Prior to the prevalent use of varicella vaccine, annual U.S. figures for varicella infection included an estimate of 4 million cases, 11,000 hospitalizations, and 100 deaths [82]. The annual incidence of varicella has decreased significantly since the use of this vaccine. The vaccine, Varivax, developed by Takahashi in 1974 and approved in 1995, is a live attenuated Oka strain vaccine that has been shown to be very safe and effective [83–85]. In May 2006 the Food and Drug Administration (FDA) approved a vaccine, Zostavax (Merck) which is 14 times more concentrated than the varicella vaccine, against herpes zoster in individuals 60 years age and older [86]. After further studies showed its efficacy in a younger cohort, the vaccine was subsequently approved by the FDA for use in individuals aged 50–59 in 2011. Cell-mediated immunity is increased following vaccination in immunocompetent older adults, and the incidence and severity of herpes zoster [87–91] is reduced. The vaccine lessened the overall incidence of herpes zoster by 51.3 % and notably reduced the pain and discomfort by 66.5 % among subjects in whom herpes zoster developed. The zoster vaccine had low rates of serious adverse events, which were similar to the placebo vaccine.
Long-term follow-up data of the varicella vaccine depicted protection against chickenpox for at least 17–19 years, and, furthermore, all of the subjects vaccinated continue to have persistent antibodies and delayed-type skin reaction to the varicella-zoster antigen [92]. In a double-blind, placebo-controlled study of the Oka vaccine in 914
U.S. children, the varicella vaccine demonstrated an efficacy of 100 % at 9 months [93, 94]. Following 7 years of vaccination, 95 % of the subjects remained without chickenpox clinically [81]. Additional studies in the U.S. have demonstrated that the Oka vaccine induces humoral and cell-mediated immunity in healthy children [94–97], with protection for at least 8 years, while other data have suggested effectiveness decreases markedly after 1 year postvaccination [98]. Further, case series depict that vaccinated individuals have less severe varicella (<50 lesions, no fever, and shorter duration of illness) than those who are unvaccinated [99, 100].
The vaccine is recommended as part of the childhood immunization schedule, and all susceptible children from 12 months of age to 18 years of age should receive the varicella vaccine. The first dose of the Oka vaccine should be administered at 12–15 months and the second dose should be given at 4–6 years of age [86]. Those over the age of 13 years should receive two doses, 4–8 weeks apart, to generate seroconversion rates and antibody responses comparable to those attained in healthy children [83–85]. In the US, childhood vaccination with a single dose continues to provide up to 95 % protection 10 years after immunization; children with two doses can see up to 98 % protection [101]. The vaccine is also recommended for susceptible adults, notably those in high-risk situations (e.g., health care personnel); children who have no prior history of chickenpox and are required to be present in school; and immunosuppressed subjects, particularly those with acute lymphocytic leukemia (ALL) [102–104]. The varicella-zoster virus (VZV) vaccination is safe for all patients with lymphocyte counts >700/mm [3]. Further, recent data suggest that varicella vaccine is >95 % effective for prevention of disease and 100 % for prevention of moderate or severe disease in susceptible contacts when given within 36 h of exposure [105].
A combination vaccine with the live attenuated viruses of MMR and VARIVAX is also available (ProQuad; Merck) and it is administered to individuals 12 months to 12 years of age. Post-licensing studies have shown the combination vaccine to be as effective as separate injections of MMR and Varivax [106].
Varicella transmission is approximately one- fourth the rate of natural varicella (20–25 % vs. 87 %) [107] if a rash develops in the immunized individual. In children, the incidence of primary varicella is between 18 and 77 per 1 million person years of follow-up [108]. Herpes zoster can later develop from this vaccine type virus or from natural wild-type varicella-zoster virus [109, 110]. Although there are reports of herpes zoster in healthy children vaccinees, the incidence is less than that seen in children with prior chickenpox, suggesting that vaccinated children may have a decreased risk for herpes zoster [111].
The most common side effects from the vaccine include mild tenderness, erythema, induration at the injection site (19.3–24.4 %), fever (10.2–14.7 %), and a localized or generalized varicella- like rash (3.8–5.5 %). The transmission rate of the varicella vaccine virus from a healthy vaccinated individual is low, but may be more likely if a rash appears subsequent to vaccination, particularly in those who are immunocompromised. Vaccinated individuals should avoid close contact with susceptible high-risk individuals for up to 6 weeks. The vaccine is contraindicated in pregnant women or any woman planning to become pregnant within 3 months since the vaccine uses a live attenuated virus and natural varicella can cause fetal harm [111].
A separate adjuvant subunit vaccine has recently been shown in Phase 3 clinical studies to be advantageous in reducing the risk of herpes zoster in adults over 50 years of age by up to 97 % [112]. The efficacy of this vaccine was shown to be similar across age group stratifications, making it more effective than Zostavax which has waning efficacy as patients increase in age. While still currently under investigation, this new adjuvant subunit vaccine represents a significant improvement in providing protection to those at highest risk for developing herpes zoster.
Yellow Fever Virus
A live attenuated vaccine produced from the 17D strain of yellow fever virus is available for subcutaneous administration. It is recommended for travelers to areas where yellow fever is endemic or enzootic, such as tropical parts of South America and Africa. Booster immunizations are required every 10 years. This vaccine is contraindicated in persons allergic to eggs, in pregnant women, in immunocompromised persons, and in children younger than 4 months of age.
Bacteria
The only currently used live-attenuated bacterial vaccine in the U.S. is against the causative agent of typhoid fever, Salmonella typhi. This vaccine, given orally, was first developed through chemical mutagenesis in vitro. Protection is conferred by mucosal and serum antibodies as well as cell-mediated immunity [113]. The induction of mucosal antibodies offers protection against infection and disease [114].
Inactivated Vaccines
Both inactivated viral and bacterial vaccines consist of viruses and bacteria, respectively, treated so that they are unable to replicate. They are less potent in the effector response than the live-attenuated vaccines, and consist of vaccines currently in use against hepatitis A, Haemophilus influenzae type b (Hib), influenza (intramuscular), Japanese encephalitis, meningococcus, pneumococcus, polio (subcutaneous or intramuscular), rabies, tetanus/diphtheria toxoids, and typhoid fever (intramuscular). Again, in this section, the focus is on the vaccines targeting diseases that cause cutaneous manifestations.
Viruses
Hepatitis A
Both the inactivated and attenuated forms of hepatitis A vaccines have been developed and studied, although only the inactivated vaccine is licensed and available in the U.S. (Havrix and Vaqta). These vaccines are propagated in human diploid fibroblast culture and inactivated by formalin. Immunization typically involves two doses given 6 –12 months apart in adults and in children aged 1 year and older. Studies of both available vaccines depict excellent safety profiles in addition to comparable immunogenicity and efficacy rates. Overall, 97–99 % of those vaccinated develop protective levels of antibodies 1 month after the first dose, and 99–100 % are protected 1 month after second dose [115–120]. Long-term data are limited, but one recent study showed that protection following primary hepatitis A vaccination persists for more than 10 years [121].
The hepatitis A vaccine is recommended for individuals at least 1 year of age living in or traveling to high endemic areas for hepatitis A. Further, individuals with chronic liver disease due to causes other than hepatitis A, persons engaging in high-risk sexual activity, residents of a community experiencing an outbreak of hepatitis A, and users of illicit injectable drugs are strongly advised to receive hepatitis A vaccination. In addition, in some states and regions, the hepatitis A vaccine is recommended for routine pediatric use.
Adverse effects with hepatitis A vaccination are typically mild, and no serious adverse side effects have been credited to the vaccine in clinical trials [122]. Side effects include soreness at the injection site, headache (14 %) and malaise (7 %) in adults, and feeding problems (8 %) and headache (4 %) in children.
Bacteria
Anthrax
Pasteur in 1881 demonstrated the protective efficacy of the first anthrax vaccine when he injected sheep with heat-attenuated Bacillus anthracis. In the 1930s, wide use of vaccine composed of attenuated strains markedly decreased the incidence of anthrax in domesticated animals in industrialized countries. The licensed human vaccine, anthrax vaccine adsorbed (AVA), also known as BioThrax since 2002, is a culture supernatant of a toxigenic, avirulent, nonencapsulated B. anthracis strain, V770–NP1–R, derived from the Sterne strain [123]. A vaccine similar to AVA is used in the United Kingdom, produced from culture supernatant-derived human vaccine (PL 1511/0058) [124].
Anthrax vaccinations are recommended for individuals working in the production of B. anthracis cultures, those engaged in activities with a high risk of aerosol exposure, individuals in the military, and other groups of persons with a calculable risk [125]. Preexposure vaccinations are currently not recommended for emergency first responders, medical practitioners, and civilians. However, due to the bioterrorist attacks in 2001 and limited supply of anthrax vaccine, supplemental recommendations of the Advisory Committee on Immunization Practices endorse the anthrax vaccine in combination with antimicrobial postexposure prophylaxis (PEP) for unvaccinated persons at risk for inhalational anthrax [126].
Anthrax vaccine adsorbed has numerous limitations despite being relatively efficacious and safe [127]. The schedule of AVA administration, which consists of subcutaneous injections at 0, 2, and 4 weeks and boosters at 6, 12, and 18 months (with recommended annual boosters to maintain immunity), is probably suboptimal. The enduring protective efficacy in humans is unknown. AVA contains other cellular elements that aid in the fairly high rate of local and systemic adverse reactions [127], including injection-site hypersensitivity, edema, pain, headache, arthralgia, asthenia, and pruritus. Approximately 20 % of vaccinees develop mild cutaneous reactions such as erythema, edema, and induration. In <1 % of recipients, systemic side effects of fever, chills, nausea, and body aches occurred [128]. Due to these limitations, newer anthrax vaccines that involve a purified form of the anthrax toxin are currently being developed, some of which have passed Phase 1 trials [129].
Conjugate Vaccines (Fig. 49.1) [1, 130]

Fig. 49.1
Antibody responses to polysaccharide antigens and polysaccharide–protein conjugates. (a) A polysaccharide antigen binds to an immunoglobulin M (IgM) receptor on the surface of a B cell in lymphoid tissues. Once B cells are activated, they produce and then secrete IgM antibody molecules. The individual Fab segments of the IgM molecule have only a moderate affinity, but because there are 10 such segments, an IgM molecule has a high avidity. (b) In contrast, some polysaccharide–protein conjugates are taken up by dendritic cells, which present peptides from the protein portion of the conjugate to type 2 helper T (Th2) cells. Other conjugate molecules bind to B cells that have IgM receptors specific for the carbohydrate moiety and will undergo endocytosis and be processed by the B cell; the resulting peptides will be expressed with major histocompatibility complex (MHC) class II molecules on the surface of the B cell
Many bacteria, including Neisseria meningitidis and Haemophilus species, have an outer capsule composed of polysaccharides that are species and type specific for particular strains of the bacterium. The goal of vaccination is to generate antibodies against the polysaccharide capsule of the bacteria since the most effective defense against these organisms is opsonization of the polysaccharide coat with antibody. However, children under 2 years of age do not mount a good response against capsular polysaccharide, which is a T-cell–independent antigen. An effective way to overcome this problem is to conjugate bacterial polysaccharide (chemically) to protein carriers, which offer peptides that can be recognized by antigen-specific T cells. Thus, a T-independent response is converted into a T-cell–dependent antipolysaccharide response [1, 39].
Haemophilus Influenzae
In the second generation of Hib vaccines, the polyribosylribitol phosphate (PRP) vaccine has been covalently conjugated with various protein molecules to form the Hib conjugate vaccine. This has resulted in a vaccine that is able to stimulate the immunologic system to produce a T-dependent response, for use in infants [131]. Four different types of the Hib conjugate vaccine have been licensed for use: PRP conjugated to the diphtheria toxoid (PRP-D), PRP conjugated to the outer membrane protein of Neisseria meningitidis group B (PRP- OMP), PRP conjugated to tetanus toxoid (PRP-T), and PRP oligosaccharides conjugated to mutant diphtheria toxin CRM (HbOC) [132]. Recommended immunization involves three doses of the Hib vaccine given at 2, 4, and 12 months of age [46].
Neisseria Meningitidis
Bacterial meningitis remains a severe threat to global health, with an estimated 500,000 cases worldwide with at least 50,000 deaths and as many cases of neurological damage [133]. Neisseria meningitidis is responsible for 60–65 % of cases of bacterial meningitis, and it is the only bacterium that is able to generate epidemics of meningitis [134].
It is also associated with a petechial eruption or more severe hemorrhagic lesions on the trunk and lower extremities.
The significant determinant of virulence in N. meningitidis is the polysaccharide capsule. Among the 13 distinct N. meningitidis serogroups that have been defined on the immunohistochemistry of their polysaccharide group, groups A, B, C, W135, and Y account for over 90 % of severe meningitis and septicemia. Two meningococcal vaccines, each containing antigens to serogroups A, C, Y, and W-135, are licensed in the U.S. In 2015 the FDA approved the first vaccine that provides immunity against serogroup B, which accounts for an estimated one third of cases of meningococcal disease. This recombinant serogroup B vaccine, Bexero (Novartis) has been licensed for use in Europe, Canada, and Australia; before being licensed for use in the US, it was used to control two separate outbreaks of meningococcal disease within the US and had been allowed by the FDA to treat certain outbreak situations [135, 136].
Over 30 years ago, the first meningococcal polysaccharide vaccine (MPSV4 or Menomune, Sanofi Pasteur), was developed against serogroups A and C among U.S. military recruits [137–139]. It is approved for all ages in which meningococcal vaccine is currently recommended. Further, the vaccine is safe and bestows protection to 90–95 % of people. However, there are limitations to the vaccine. First, the vaccine offers a short duration of immunity: 1–3 years in children younger than 5 years of age [134, 140] and 3–5 years in adolescents and adults. Second, like other polysaccharide vaccines, this vaccine could not elicit memory T cells, and even after repeated injections, booster responses are low [141, 142]. In addition, like other polysaccharide vaccines, this vaccine does not prevent mucosal colonization and hence does not offer herd immunity by breaking up the transmission of N. meningitidis [143, 144]. This vaccine is a logical option for individuals requiring protection for a limited amount of time.
The second vaccine, meningococcal conjugate vaccine A, C, Y, and W135 (MCV4 or Menactra, Sanofi Pasteur), was approved in January 2005 for use in individuals 11–55 years of age. This conjugate vaccine contains the same antigen as that found in the meningococcal polysaccharide vaccine, but it is conjugated to 48 μg of diphtheria toxoid [145]. What is different regarding this vaccine is that immunity is more durable, and revaccination can generate a rise in antibody level. Although not yet proved, it is suggested that, like other conjugate vaccines, it is likely that this vaccine will offer more durable protection than the polysaccharide vaccine alone and will further reduce nasopharyngeal carriage, allowing herd immunity to occur.
The serogroups A and C vaccines have demonstrated estimated clinical efficacies of >85 % among school-aged children and adults and are useful in controlling outbreaks. Serogroups Y and W-135 polysaccharides are safe and immunogenic among adults and children aged >2 years. The advantages of the meningococcal conjugate vaccine have led the Advisory Committee on Immunization Practices (ACIP) of the CDC to extensively widen the recommendations for immunization, with routine vaccination of all persons aged 11 through 18 years old, composed of a single dose at age 11–12 years and a booster dose at age 16 [146].
Both vaccines are currently recommended for use in control of outbreaks as a result of N. meningitidis serogroups A, C, Y, and W-135 [145]. However, due to its enhanced booster effect, the conjugate vaccine is favored when revaccination is indicated for individuals who have received the meningococcal polysaccharide vaccine before and who remain at higher risk. Long-term follow-up studies are still needed for those that have been immunized with the meningococcal conjugate vaccine to establish if boosters are needed.
Adjuvants
Purified antigens are not typically immunogenic on their own, and thus most acellular vaccines require adjuvants to enhance their immunogenicity. Alum, the adjuvant most often used, delays the release of an antigen and enhances the generation of antibodies. Most adjuvants are thought to act on antigen-presenting cells, particularly on dendritic cells. Adjuvants manipulate the immune system into reacting as though there were an active infection, and just as different classes of infectious agents elicit different types of immune response, different adjuvants may stimulate different types of response.
Recombinant Subunit Vaccines
Another route to vaccine development is the identification of the T-cell peptide epitopes that stimulate protective T-cell–mediated immunity. T cells recognize their target antigens as small protein fragments or peptides presented by major histocompatibility complex (MHC) molecules at the cell surface, and thus these peptide epitopes, once identified, can then be tried as vaccines. Using peptides has many advantages and some drawbacks [1]. Advantages include that the peptide is chemically defined, stable, and safe and contains only important B-cell and T-cell epitopes. Drawbacks include the complexity in mimicking the conformation of antigen polymers found with many viruses, that B-cell epitopes recognized by neutralizing antibodies are sometimes discontinuous sequences, and the vulnerability of peptides to proteolysis. Several administrations, likely with an adjuvant, may be required. Since 1990, over 100 chemically synthesized short peptide vaccines have been initiated into phase I clinical trials, less than 20 have advanced into phase II, and none has entered phase III clinical trials [147].
Subunit vaccines have often made use of recombinant-DNA technology. Immunogenicity can be improved and the immune response aimed at induction of both cell-mediated and humoral responses through the formation of aggregates such as immunostimulating complexes, virus-like particles, antigen-coated beads, or lipid-encapsulated antigens under many conditions. Linked peptides are also being tested in the field of subunit vaccines; for instance, aggregates of linked peptides from group A streptococcus as a vaccine against rheumatic fever have demonstrated promising results in animal models [148, 149].
Viruses
Human Papillomavirus
According to the WHO, cervical cancer is the fourth most common cause of death in women globally from cancer, and it is the cause of death of approximately 265,000 women per year [150]. Almost half a million new cases of invasive cervical cancer develop each year, and in the U.S. it was estimated to afflict over 11,800 women in 2010 [151]. Epidemiologic studies have proved that persistent HPV infection is the main cause of almost all cervical carcinogenesis [152–154].
Approximately 40 of the 100 HPV genotypes have an affinity for infecting the mucosal epithelium and subsequently causing genital infection. The major types associated with malignancy (16, 18, 31, 33, 45, 52, and 58) and condyloma (6 and 11) are relatively few in number, which has allowed for more focused strategies for immunization against these specific types [111].
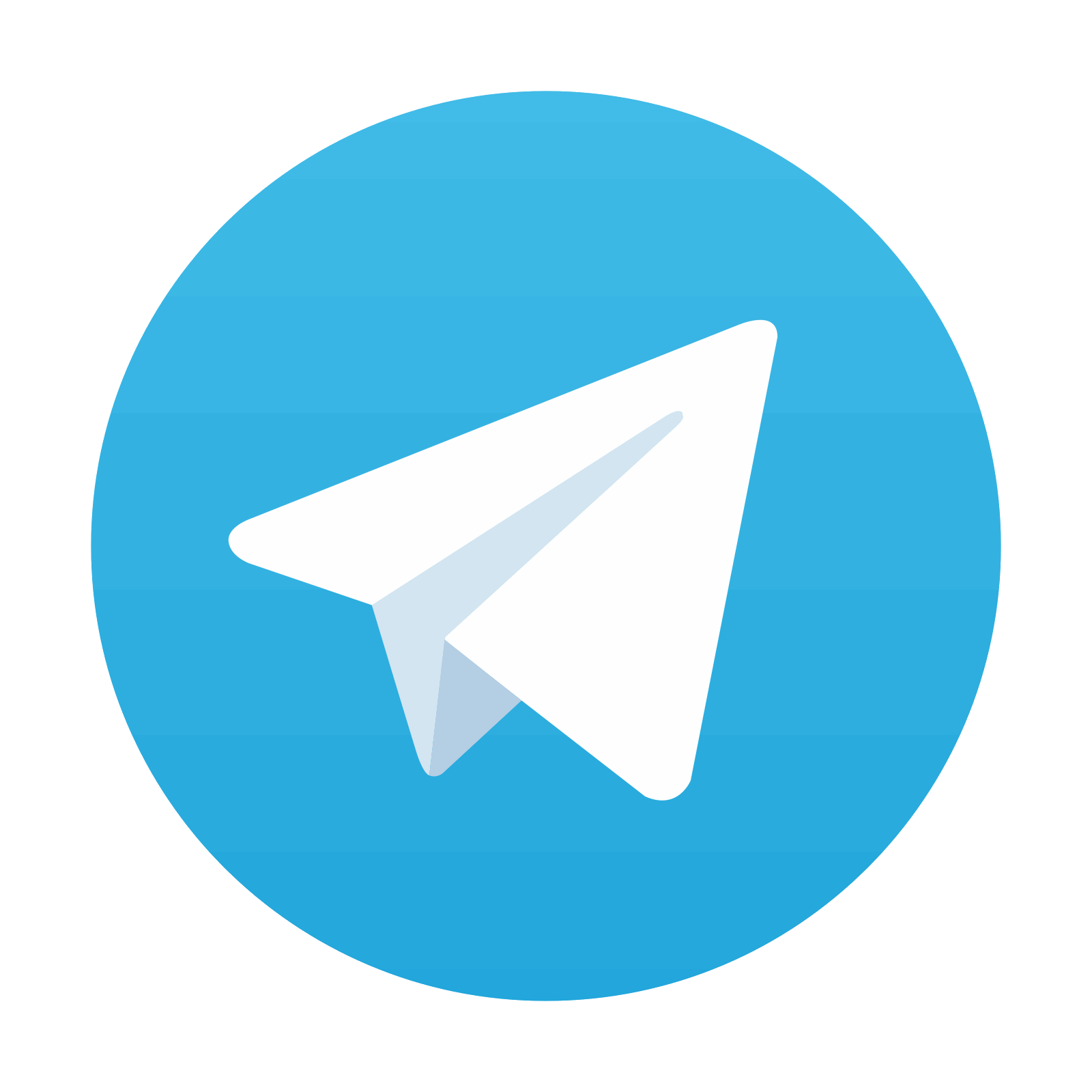
Stay updated, free articles. Join our Telegram channel

Full access? Get Clinical Tree
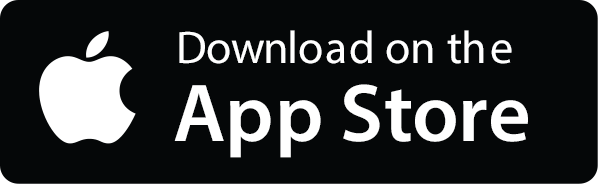
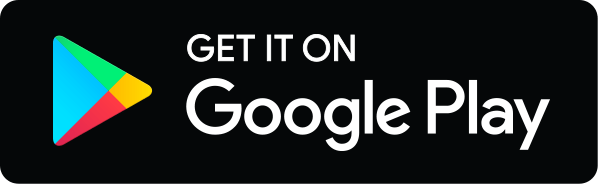
