Fig. 3.1
Multiple wartlike lesions on dorsum of the hands
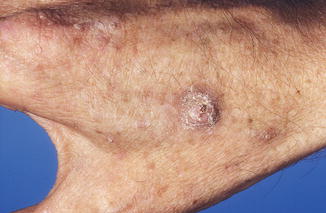
Fig. 3.2
Squamous cell carcinoma on dorsum of the hand
3.2 Human Papillomaviruses
3.2.1 HPV Genome and Taxonomy
Papillomaviruses (PV) are nonenveloped circular double-stranded DNA viruses belonging to the family papillomaviridae. The genome is approximately 8 kb and slightly varies in size between types. More than 150 types have been identified today, and the number is still increasing (Fig. 3.3). The genome is subdivided in an early (E) coding region, a late (L) coding region, and a long control region (LCR). The early region generally encodes for six nonstructural viral regulatory proteins (E1, E2, E4, E5, E6, and E7 in most PV types and an additional E8 in some PV types) involved in several functions including transformation, transcription, and viral adaptation to different cellular milieus [23]. The late region encodes for two structural proteins, namely, L1 and L2 [24]. Whereas all PV appear to have an E1, E2, L1, and L2 open reading frame (ORF), the other ORFs are not consistently present in every PV [13]. Beta-PV have E1, E2, E4, E6, E7, L1, and L2 ORFs, but lack E5.
The L1 ORF encoding for the major capsid protein L1 is relatively well conserved between HPV types. The L1 protein is the basis for currently registered prophylactic vaccines against HPV types 6, 11, 16, and 18 infections that cause genital warts and cervical cancer. The current papillomavirus classification system is based on DNA sequence homology of the L1 ORF and comprises a division in genus, species, type, subtype, and variants. The phylogenetic tree is shown in Fig. 3.3 [22].
As stated before, HPV can be divided into alpha, beta, gamma, mu, and nu genera. Species of the same genera share at least 60 % homology of the L1 ORF. A new type is defined as one in which the complete nucleotide sequence ORF of the L1 gene differs by more than 10 % from the most closely related known PV type. Table 3.1 shows all the HPV types according to the genus, based on the Papillomavirus Episteme database (http://pave.niaid.nih.gov/#home). The beta, gamma, mu, and nu genera are cutaneous types. The alpha genus contains all the mucosal types but also some cutaneous types (HPV2, 3, 10, 27, 28, 29, 57, 77, 94) and mucocutaneous types (HPV7, 40, 43, 91) [22].
Table 3.1
HPV types sorted by genus
Genus | HPV type |
---|---|
Alpha | 2, 3, 6, 7, 10, 11, 13, 16,18, 26, 27, 28, 29, 30, 31, 32, 33, 34, 35, 39, 40, 42, 43, 44, 45, 51, 52, 53, 54, 55, 56, 57, 58, 59, 61, 62, 66, 67, 68, 69, 70, 71, 72, 73, 74, 77, 78, 81, 82, 83, 84, 85, 86, 87, 89, 90, 91, 94, 97, 102, 106, 114, 117, 125, 160 |
Beta | 5, 8, 9, 12, 14, 15, 17, 19, 20, 21, 22, 23, 24, 25, 36, 37, 38, 47, 49, 75, 76, 80, 92, 93, 96, 98, 99, 100, 104, 105, 107, 110, 111, 113, 115, 118, 120, 122, 124, 143, 145, 150, 151, 159 |
Gamma | 4, 48, 50, 60, 65, 88, 95, 101, 103, 108, 109, 112, 116, 119, 121, 123, 126, 127, 128, 129, 130, 131, 132, 133, 134, 135, 136, 137, 138, 139, 140, 141, 142, 144, 146, 147, 148, 149, 153, 154, 155, 156, 161, 162, 163, 164, 165, 166, 169, 170 |
Mu | 1, 63 |
Nu | 41 |
3.2.2 HPV Life Cycle
HPV infection occurs when the virus enters the basal layer of the epithelium, supposedly achieved by small abrasions of the epithelium. However, the body-wide distribution of especially Beta-PV infections suggests a direct route of infection, bypassing the requirement of epithelial injury or abrasion [25–29]. Life cycle studies have been mainly performed for the HPV types causing cervical cancer, but the life cycle of several HPV types with a cutaneous tropism appears to be similar [30].
E6 and E7 are mainly expressed in the (supra)basal layer and the granular layer of the epithelium and are associated with PV genome maintenance and cellular proliferation. E1 and E2 are involved in viral DNA replication and the regulation of E6 and E7 transcription. E4 of some cutaneous HPV types appears to be expressed throughout the epithelium except in the basal cell layer [31] and presumably promotes viral DNA replication [32]. There is some variability in viral life cycle between PV types. However, the order of expression of viral genes throughout the differentiating epithelia is similar, although the localization of E4 and L1 expression is variable and starts either in the lower part of the epithelium (HPV1 and 2) or in the upper part (HPV63 and 65) [33].
3.2.3 Beta-PV Detection Methods
There are different methods for Beta-PV detection, based on measurement of viral protein, viral DNA, or serum antibody responses. Tissue specimens can be collected via several sampling methods like skin swabs, plucked hairs, biopsies, and blood [34]. Several PCR-based methods targeting the HPV L1, E1, or E7 ORF have been developed to detect Beta-PV types in skin biopsies, plucked hairs, and skin swabs [25, 26, 35–45].
These PCR methods can be divided into (I) type-specific PCRs, using HPV type-specific primers for the detection of a single HPV type; (II) multiplex type-specific PCR methods, in which multiple type-specific primer sets are combined in a single PCR reaction; (III) broad-spectrum PCR methods using consensus primers, which permit simultaneous amplification of multiple types; and (IV) quantitative PCR methods that allow viral DNA quantification.
Multiplex type-specific PCR and broad-spectrum PCR–based methods amplify multiple HPV types in a single reaction. Subsequent genotyping in the case of amplification systems that target multiple HPV types is generally performed by sequence analysis, either directly or preceded by cloning of the amplimer. Sequencing, however, does not easily permit the identification of HPV types present in frequently occurring multiple infections [39, 46]. Therefore, the more recently developed PCRs are followed by reverse hybridization technologies or APEX for the simultaneous identification of multiple HPV types [39, 41, 45, 47].
With viruslike particle (VLP) enzyme-linked immunoassay (ELISA) or multiplex technology (Luminex), antibodies against Beta-PV viral proteins can be detected, to determine a person’s Beta-PV serological status. Serologic responses are usually measured against the major capsid protein L1 and the nonstructural protein E6 using HPV viruslike particle (VLP) or GST-HPV fusion proteins in ELISA [48, 49] or with multiplex serology using GST-L1 fusion proteins, respectively [50]. The latter method (Luminex) is based on fluorescent bead technology that allows simultaneous detection of antibodies against up to 100 different in situ affinity-purified recombinant HPV proteins [51]. However, not all HPV infections induce an antibody response to HPV, and cross-reactive antibodies between different HPV types may be produced [52]. Nevertheless, serologic antibody responses provide a useful epidemiologic tool to explore HPV infections in certain populations.
Detection of papillomavirus proteins and DNA in paraffin-embedded tissue sections is possible by combining in situ hybridization and fluorescence detection methods (FISH = Fluorescent DNA in situ hybridization) [53]. Also laser capture microdissection can be used to specifically test a particular part of a histological specimen for HPV presence and viral load [54].
3.2.4 Beta-PV Replication, Cell Transformation, and Carcinogenesis
After infection, Beta-PV will start to replicate in the epidermal keratinocytes. As for mucosal HPV, keratinocyte differentiation is probably also crucial for Beta-PV to fulfill their life cycle [55, 56]. It is expected that in the basal epidermal layers a low level of replication of Beta-PV DNA is maintained to ensure viral episome distribution among daughter cells, and especially the early (E) genes will be expressed [57].
Transformation of human cells has been studied in great detail for the HPV types causing cervical cancer (e.g., HPV16 and HPV18). In general, transformation requires long-term and deregulated expression of viral oncogenes E6 and E7. This process is facilitated by integration of the viral episome into the cellular DNA, disturbing the E2 ORF, thereby causing a lack of control of E6 and E7 expression [32]. In general, Beta-PV are found episomal, also in actinic keratoses and SCC, although (integrated) HPV has been occasionally isolated from an SCC metastatic lesion in an OTR [43, 58].
Since papillomaviruses depend on (unscheduled) host cell DNA replication in order to produce progeny, the virus tends to keep its host cell in a replicative state (S-phase) as long as possible. This is particularly relevant as terminal keratinocyte differentiation is a dead-end road resembling programmed cell death (apoptosis) [59, 60]. For high-risk mucosal HPV types, it is known that the E7 early gene product drives cells into S phase by binding and degrading the tumor suppressor protein pRb [61]. For HPV38, a Beta-PV type that has been associated with SCC in some studies, this has been shown as well, but it does not seem to be a mechanism exploited by all Beta-PV types. For the high-risk mucosal HPVs, such as HPV16 and HPV18, this mechanism acts through ubiquitination and subsequent degradation of the tumor suppressors p53 and pRb. Presumably, persistent infections are needed to accumulate sufficient mutations in the host cellular genome to generate a malignant cell. The proliferative phenotype of these malignant cells remains dependent on E6/E7 expression [62].
HPV38 E7 was shown to bind and degrade the tumor suppressor pRb similar as HPV16 E7 [63, 64]. Furthermore, HPV38 E6 and E7 are sufficient to deregulate the cell cycle and senescence programs in primary human keratinocytes, thereby increasing the lifespan of human skin keratinocytes [63, 65, 66]. HPV38 E6, possibly in combination with E7, induces telomerase activity, which plays a key role in transformation of human keratinocytes [67].
Another mechanism behind the lack of cell cycle arrest in Beta-PV expressing cells might be the upregulation of delta-Np73 as a result of p53 accumulation [68, 69]. This upregulation prevents p53 to induce the transcription of genes involved in apoptosis and growth suppression, altering the regulation of cell cycle checkpoints that are normally activated by UV radiation [69].
Several studies investigating the effect of Beta-PV E6 and E7 in organotypic (raft) cultures showed that Beta-PV could delay cell differentiation and disturb keratinocyte outgrowth [66, 70–72].
As the vast majority of SCC occurs on sun-exposed sites, it is generally believed that Beta-PV, if involved in skin cancer development, somehow cooperates with UV radiation in transforming cutaneous epithelial keratinocytes. Beta-PV could confer its possible carcinogenic effect via inhibition of DNA repair and apoptosis in UV-damaged cells which is illustrated in Fig. 3.4 [73].
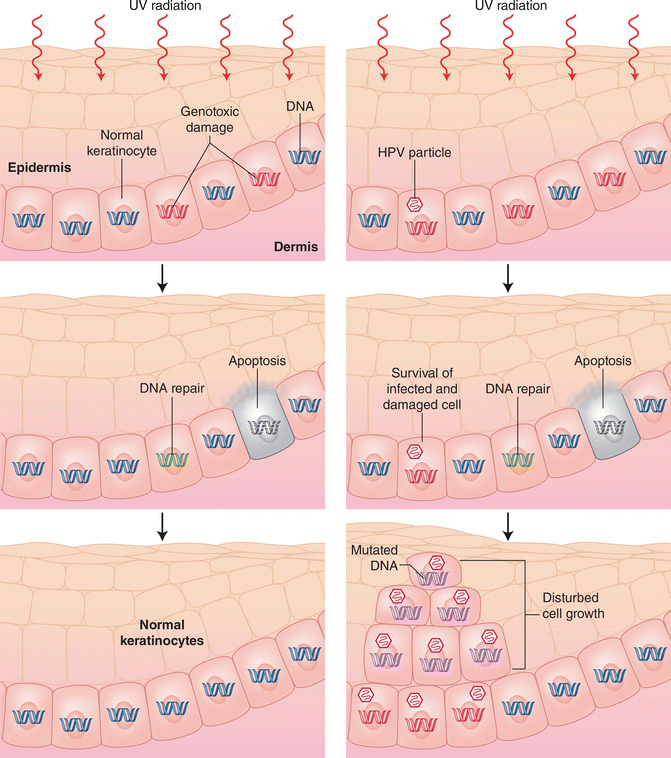
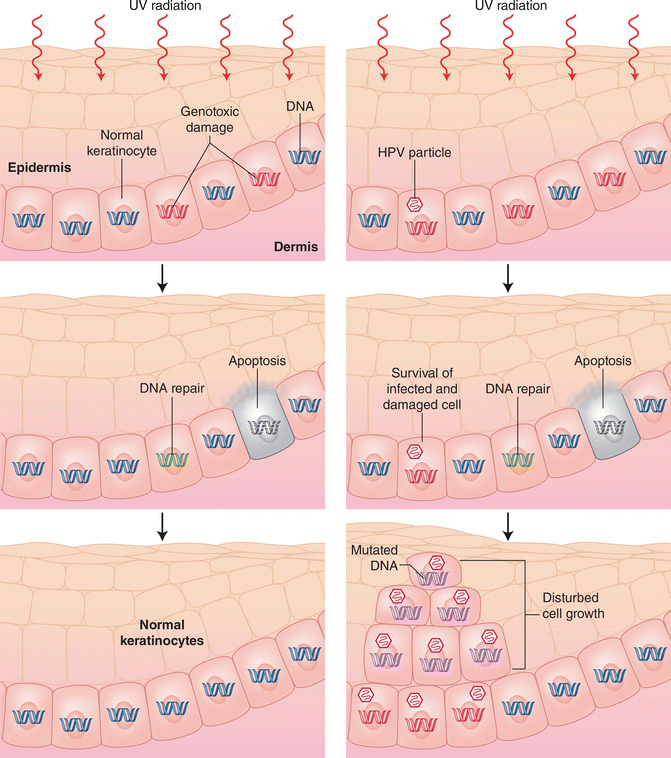
Fig. 3.4
Ultraviolet radiation causes genotoxic damage in the keratinocytes in the basal layer of the epidermis. Panels on the left illustrate DNA repair in the cells or dying due to apoptosis. The panels on the right show the situation when a keratinocyte is infected with HPV. The infected keratinocyte has low ability for DNA repair and is less sensitive to apoptosis. The DNA mutated cell can proliferate and multiply and lead to dysplastic keratinocytes. Currently it is believed that not every mutated cell carries a virus episome (Permission for reprint accepted by NEJM (rights JNBB))
HPV5 E6 appeared to exert this effect via the degradation of Bak, a protein involved in induction of apoptosis [74–76]. The E6 of HPV8, 20, 22, 38, 76, 92, and 96 can protect UV-treated keratinocytes from apoptosis [77, 78]. The oncoproteins E6 and E7 from Beta-PV38 significantly contribute to SCC development in the skin rendering keratinocytes more susceptible to UV-induced carcinogenesis in mice [79, 80]. Interference with UV-induced DNA repair and apoptosis by Beta-PV might occur, and epidemiological studies seem suggestive of a joint effect of UV radiation and Beta-PV infection as well [50, 73, 81].
Still other studies have shown that the E6 proteins of HPV5 and HPV8 inhibit the transforming growth factor beta (TGF-beta) signaling pathway by the degradation of the SMAD3 transcription factor [82]. TGF-beta-triggered pathways lead to the synthesis of inhibitors (p16, p17, p21, and p27) of the cyclin-dependent kinases that play a crucial role in the cell cycle. It can be postulated that specific degradation of SMAD3 could negatively regulate inhibitors of the cell cycle and favor cell transition from the G1 to the S phase, allowing viral DNA vegetative replication and, as a side effect, cell transformation.
Additionally, there is evidence that the association of HPV5 and HPV8 E6 proteins with MAML1 inhibits Notch signaling and that Notch signaling plays a role in both virus–host perturbations and tumor genesis [83].
In HPV-positive skin cancers, not all tumor cells contain HPV DNA, and the viral load is higher in actinic keratoses [84]. If Beta-PV are involved in the development of skin cancer, they probably play a role in tumor initiation and progression, not in maintenance of the malignant phenotype, through a “hit-and-run” mechanism of viral carcinogenesis [79].
3.3 Epidemiology of HPV Infection and Skin Cancer
3.3.1 HPV Carriage in Humans
HPV DNA can frequently be detected in skin swabs and plucked hairs in newborns, children, and adults [29, 34, 50, 85–89]. Family members may share some of the same HPV types [29, 90]. The prevalence of HPV DNA ranges between 42 and 87 %, and 84 and 91 %, respectively, using skin swab and plucked hair sampling, and varies also between geographical regions and ethnicities [25, 29, 46, 90–95].
Viral DNA is persistent in/on the skin [86, 89, 95]. Increasing age, sun exposure, sunburn, skin type, and a medical history of skin cancer are factors which are associated with an increased prevalence of HPV DNA [4, 25, 91, 96, 97]. Duration of immunosuppression and immunosuppression itself in OTR is also associated with an increased prevalence of HPV DNA in plucked hair samples compared with the immunocompetent population [46].
Seropositivity for one or more HPV types is approximately 60 % in the general population (most frequently for HPV 8 followed by HPV 15, 17, 38, and 49), but can be higher in certain subpopulations [97–99]. Serological responses to HPV are also more common in OTR and vary between 80 and 90 % to at least one HPV type. Between 45 and 56 % of these positive seroresponses are against Beta-PV types. Most commonly detected Beta-PV types are HPV 8, 15, 17, 38, and 49 [25, 100, 101].
3.3.2 Presence of HPV DNA in Skin Cancer and Precursor Lesions
Several studies have investigated the prevalence of HPV DNA in SCC and precursor lesions in both immunocompetent individuals and OTR. HPV carriage was nicely summarized by Aldabagh et al. [34]. The percentages of HPV DNA positivity vary between immunocompetent individuals and OTR and depend on the detection methods used. The prevalences of HPV DNA in SCC in the immunocompetent population range between 26 and 69 %. HPV DNA can also be found in benign lesions, actinic keratosis, and normal and sun-exposed skin from both patients with and without SCC [84, 94, 102–105]. Frequently, Beta-PV types, including HPV 5 and 8, are detected in higher rates in actinic keratoses compared to SCC [106]. Viral load of HPV infection was found to be significantly higher in actinic keratoses compared to SCC [84]. In a small study Beta-PV DNA was found to be more often present in perilesional skin than in SCC and mirror site healthy skin [107], but there are also studies reporting that Beta-PV species 2 is more likely to be identified in SCC than in adjacent healthy skin [102, 103].
Numerous studies were carried out in OTR to assess the presence of HPV DNA in keratinocyte carcinomas. De Villiers et al. found HPV DNA positivity in 91 % of SCC and in situ SCC in 25 OTR, the most prevalent types being among the Beta-PV types [38]. Berkhout et al. found similar frequencies and distribution of Beta-PV types in hyperkeratotic papillomas, actinic keratosis, and SCC, but lower in BCC, benign lesions, and normal skin [108].
Some studies have compared the prevalence of HPV DNA in SCC and precursor lesions of immunocompetent individuals with OTR. In a German study, HPV DNA was detected more frequently in SCC of OTR (75 %) than in immunocompetent patients (47 %) [109]. Similar HPV prevalences were found in cutaneous warts (91 % vs. 94 %), premalignant skin tumors (38 % vs. 36 %), and normal skin specimens (17 % vs. 16 %) for both patient populations. HPV types 5 and 8 were found more frequently in SCCs and only in the SCC of the OTR [109]. In a Dutch group of OTR with and without skin cancer, the prevalence of Beta-PV DNA in benign keratotic skin lesions was equally high, around 50 % [108–110]. A higher prevalence of Beta-PV DNA was found in lesions from sun-exposed sites with a history of skin cancer [110]. In a Scottish study no difference was detected between lesions from immunocompetent individuals and OTR with HPV 15, 24, and 38 as the most frequently detected types. In this study multiple infections were more common in tumors from immunocompetent individuals (70 %) compared with those from OTR (26 %) [111].
3.3.3 Association Between HPV DNA and Skin Cancer
Case–control studies investigating the association between the presence of Beta-PV DNA and SCC are summarized in Table 3.2. Most studies were performed with immunocompetent patients and show a statistically significant association between the presence of Beta-PV DNA and SCC or its precursor actinic keratoses, or a nonsignificant trend in the same direction (Table 3.2). However, in a large international case–control study, the presence of Beta-PV DNA in eyebrow hairs was only significantly associated with an increased risk of SCC in the Netherlands, but not in Italy and Australia [87]. Overall the Beta-PV DNA positivity was more than 90 % for all participants [87]. So far, only one large study was performed with European OTR showing a 2.4 times increased risk of SCC in OTR with Beta-PV DNA in eyebrow hairs [116].
Table 3.2
Epidemiological studies summarizing the association between Beta-PV DNA and SCC development
Author (Ref.) | Study type | Population/country | Infection marker | Method | HPV types | Cases | Controls | Adjusted odds ratio (95 % CI) | Comments |
---|---|---|---|---|---|---|---|---|---|
Boxmanet al. [36] | Nested case–control | IC/Australia | DNA in eyebrow hairs | Nested PCR | Beta | 64 KC* | 64 | 0.8 (0.3–1.8) | *BCC/SCC/intraepithelial carcinoma/KC undefined |
51 BCC | 51 | 0.6 (0.2–1.5) | |||||||
25 SCC | 25 | 2.0 (0.5–8.0) | |||||||
Boxman et al. [112] | Cross sectional | IC/Australia | DNA in eyebrow hairs | Nested PCR | Beta | 276 AK | 231 | 3.4 (1.8–6.5) (M) | Significant association between Beta-PV and AK only in men |
1.0 (0.6–1.8) (F) | |||||||||
Struijk et al. [113] | Case–control | IC/the Netherlands | DNA in eyebrow hairs | Type-specific PCR | 2, 5, 8, 15, 16, 20, 24, 38 | 155 SCC | 371 | 1.7 (1.1–2.7) | Association between Beta-PV and SCC with increasing age and male sex |
Harwood et al. [114] | Case–control | IC/UK | DNA in normal skin biopsies | Degenerate/nested PCR | Beta | 10 KC* | 29 | 6.4 (1.8–22.9) | *BCC/SCC |
Struijket al. [49] | Case–control | IC/Australia | DNA in eyebrow hairs | Type-specific PCR | 5, 8, 15, 20, 24, 38 | 126 AK | 57 | 1.6 (0.8–3.0) | |
64 SCC | 0.9 (0.4–2.0) | ||||||||
McBride et al. [115] | Prospective | IC/Australia | DNA in eyebrow hairs | Nested PCR | Beta | 71 (1–10) AK | 179 | 1.8 (0.7–4.4) | Association with having more than 10 AK. Significant associations with age over 60 years, fair skin color, high sun exposure |
41 > 10 AK | |||||||||
Bouwes Bavinck et al. [87] | Case–control | IC/Australia (AU), Italy (I), the Netherlands (NL) | DNA in eyebrow hairs | PCR–reverse hybridization assay | Beta | 689 SCC | 845 | 2.8 (1.3–5.8) NL | |
1.7 (0.79–3.6) I | |||||||||
0.91 (0.53–1.6) AU | |||||||||
Proby et al. [116] | Case–control | IS/France, Italy, the Netherlands, UK | DNA in eyebrow hairs | PCR–reverse hybridization assay | Beta | 210 SCC | 394 | 2.4 (1–5.4) | For HPV5, 9, 24, and 36 |
High viral load of Beta-PV DNA in eyebrow hairs was reported to be associated with increased risk of cutaneous SCC in immunocompetent Australian patients and in OTR, with total load seemingly more important than the load of any specific type [117].
3.3.4 Association Between HPV Serology and Skin Cancer
Most studies investigating the association between serologic antibody responses to HPV and SCC were also carried out in the immunocompetent population and show a statistically significant association between the presence of Beta-PV antibodies and SCC, or a nonsignificant trend in the same direction (Tables 3.2 and 3.3). The odds ratios are increasing when there are more positive serological responses against increasing numbers of Beta-PV types [48, 50, 121, 124, 125]. The association with SCC is exclusively found for Beta-PV types, particularly HPV 5, 8, 9, 15, 17, 20, 24, 36, 38, 49, 75, 76, and 92, but not for Alpha-, Gamma-, Mu-, or Nu-PV types [118].
Table 3.3
Epidemiological studies summarizing the association between Beta-PV seroprevalence and SCC development
Author (Ref.) | Study type | Population/country | Infection marker | Method | HPV types | Cases | Controls | Adjusted oddsratio (95 % CI) | Comments |
---|---|---|---|---|---|---|---|---|---|
Stegeret al. [119] | Case–control | IC/Germany | L1 serology | Western blot | 8 | 11 | 445 | 10.7 (2.5–63.2) | |
Starket al. [120] | Case–control | IC/Germany | L1 serology | ELISA | 8 | 14 SCC | 210 | 30.3 (7.4–142.5) | |
Bouwes Bavinck et al. [121] | Case–control | IC/the Netherlands | L1 serology | ELISA | 8 | 13 SCC | 82 | 3.1 (0.7–13.3) | |
Feltkamp et al. [48] | Case–control/Netherlands | IC/the Netherlands | L1 serology | ELISA | 5, 8, 15, 20, 24, 38 | 540 SCC | 333 | 1.4 (0.8–2.5) | |
Masini et al. [122] | Case–control | IC/Italy | L1 serology | ELISA | 8, 15, 23, 36 | 46 SCC | 84 | 3.2 (1.3–7.9) (HPV8) | |
0.4 (0.2–0.9) (HPV15) | |||||||||
1.0 (0.3–3.3) (HPV23) | |||||||||
2.8 (0.8–10.0) (HPV36) | |||||||||
Karagas et al. [50] | Case–control | IC/USA | L1 serology | Multiplex | Beta | 252 SCC | 461 | 1.5 (1.1–2.1) | |
Struijk et al. [49] | Case–control | IC/Australia | L1/E6 serology | ELISA | 5, 8, 15, 16, 20, 24, 38 | 126 AK | 57 | 2.3 (0.9–4.9)(L1) | Associations between Beta-PV L1 and E6 serology and AK/SCC |
64 SCC | 0.6 (0.3–1.3) (E6) | ||||||||
3.9 (1.4–10.7) (L1) | |||||||||
0.5 (0.2–1.1) (E6) | |||||||||
Casabonne et al. [123] | Nested case–control | IC/UK | L1 serology | Multiplex | Beta | 39 SCC | 80 | 0.5 (0.1–1.7)* | Association between 1* or 2+ ** Beta-PV type(s) and SCC |
1.0 (0.4–2.5) ** | |||||||||
Karagas et al. [124] | Case–control | IC/USA | L1 serology | Multiplex | Beta | 663 SCC |