1 Understanding lasers, lights, and tissue interactions
Summary and Key Features
• Lasers and flashlamps can destroy histological targets using the concept of selective photothermolysis (SP)
• Ablative lasers vaporize tissue; non-ablative lasers heat tissue without vaporization
• Selective histological damage requires heat confinement to desirable target structures. Selective photothermolysis combines appropriate wavelength (‘color’ of light), fluence (‘dose’ of light), pulse duration, and protective skin cooling for the treatment of a variety of diseases
• Understanding the optical and thermal properties of skin and its histological targets allows safe and optimal treatments using light sources
Light
Lasers that vaporize a thin layer or column of tissue have also been developed. The concept of fractional photothermolysis (FP), reported by Manstein and colleagues in 2004, recently launched another era of lasers in dermatology, in which patterns of very small non-selective thermal damage zones are used to stimulate skin remodeling without scarring. Laser-stimulated remodeling is a complex process that mimics large wound healing in some aspects, with epidermal regeneration, induction of metalloproteinases, and formation of new dermal matrix including elastin fibrils and collagen types I and III. Compared with gross wound healing, there is minimal inflammation and no scarring. A ‘cookbook’ approach should be avoided when choosing among these devices for various applications. When treating a particular patient with a particular device, a combination of fundamental understanding, careful observation of the appropriate clinical end points, dexterity, and clinical experience is far better than a set of instructions (Box 1.1).
Box 1.1
How to choose a light source?
2. Choose correct light wavelength (nm) based on histological target chromophore
3. Observe whether continuous wave (CW) or pulsed source is required
4. Choose right pulse width if necessary (seconds)
5. Choose pulse frequency (Hz), if necessary
6. Set skin cooling parameters
7. Choose appropriate light dose
8. Test laser to check whether it is working properly
9. Trigger single pulse on target skin and observe clinical end point
10. Adjust dosimetry if necessary
11. If no unwanted sign is observed, continue with treatment
a. Anesthetize area if necessary (e.g. tattoos, ablative lasers)
b. Wear appropriate personal protection (e.g. wavelength specific eye goggles / glasses, fume-resistant mask, gloves)
c. Turn smoke evacuator on if performing ablative procedures
d. Dress the area with petrolatum ointment and protect from sun exposure until treated area is healed
Light interactions with skin
Laser dosimetry is extremely important for safe and effective results. In order to remove tissue, ablative lasers must raise local tissue temperature beyond the boiling point of 100oC, plus add much more energy needed for changing water into steam. The fundamental unit of energy is a joule (J). It takes 4.2 J to heat 1 cm3 of water by 1oC. In order to vaporize the same 1 cm3 of water, more than 2000 J are required. An ablative laser must deliver about 2500 J of energy per cm3 of vaporized tissue. Not only is a lot of energy required to ablate skin tissue – the energy must be delivered quickly to remove the hot tissue before heat is conducted deeply into the skin, causing a burn. The standard ablative lasers in dermatology are erbium (2940 nm) and CO2 (10 600 nm). The desired interaction of these ablative lasers is to precisely remove a thin layer for resurfacing or narrow column for fractional treatment of skin, leaving behind minimal residual thermal damage. A thin residual thermal damage layer, typically about 0.1 mm, is useful in practice for hemostasis. Minimum residual thermal injury is achieved with ablative lasers by a combination of wavelength, pulse duration, and power density (W/cm2) at the skin surface. A common mistake made by beginning laser users is to ‘turn down’ the power of a surgical CO2 laser in a misguided attempt to exercise caution. Unfortunately, turning down the power can cause burns because the process turns from rapid, precise vaporization with minimal thermal damage to bulk heating of the skin from unwanted residual heat. Fortunately, many of the ablative lasers made specifically for dermatology are designed to stay within a range of dosimetry for rapid tissue ablation, making this scenario less likely. The safest erbium and CO2 lasers are those emitting high power, high energy, and short (less than a few ms) pulses, designed specifically for dermatologic use with minimal residual thermal damage. Despite whatever safeguards an ablative laser may offer, the most reliable safeguard is an ability to recognize the desired and undesired immediate response end points. For example, immediate contraction of the skin is always a sign that substantial thermal injury of the dermis has occurred (Fig. 1.1).
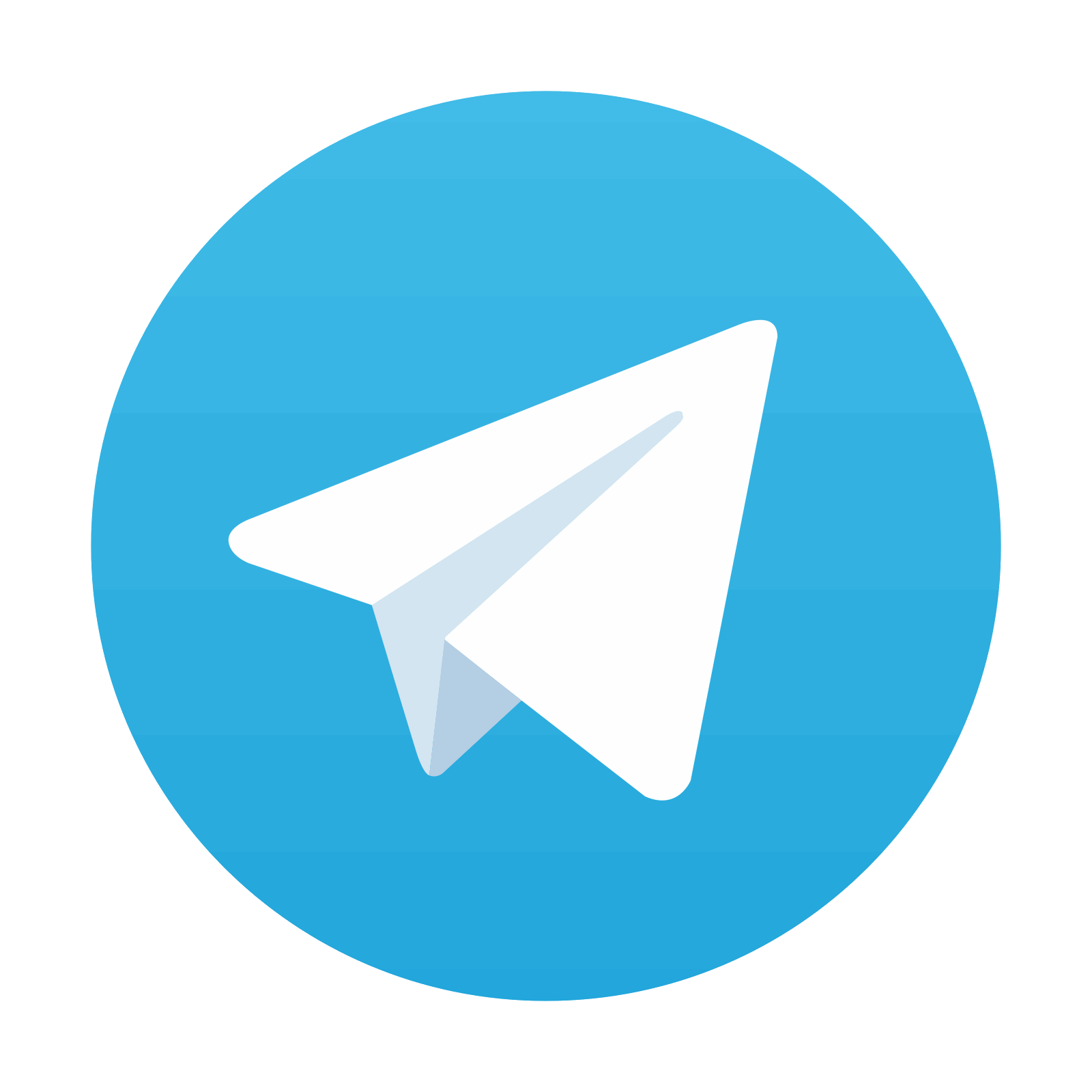
Stay updated, free articles. Join our Telegram channel

Full access? Get Clinical Tree
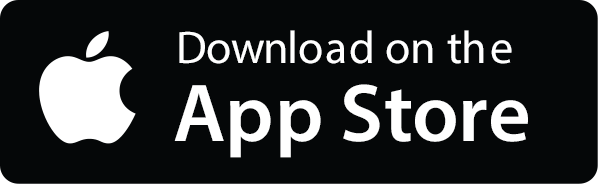
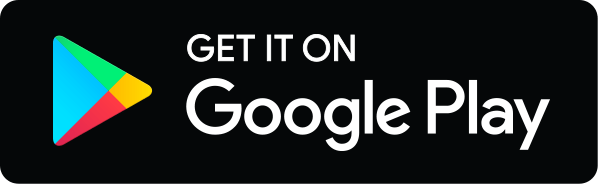