45 Biomechanical In Vitro Motion Segment Analysis The pathophysiological mechanisms of low back pain continue to be poorly elucidated and difficult to study effectively. Whereas pain resulting from neurological compression has been traditionally treated with great success via decompressive procedures, treatment of mechanical or diskogenic-type lumbar pain has proven far more problematic. For many patients who remain refractory to conservative or less aggressive modalities, spinal fusion continues to be the mainstay of surgical treatment for the relief of axial back pain.1–7 Unfortunately, clinical outcomes have been variable and inconsistent with regard to the efficacy of spinal fusion in relieving lumbago as measured by standardized measures such as the Oswestry Disability Index, visual analog pain scale, and short form SF-36 Health Survey.1 To compound the problem, accelerated degeneration of the adjacent segment has also been observed in biomechanical laboratory investigations, on long-term radiological studies, and in numerous retrospective clinical surgical series.8–15 Although the exact incidence of this “adjacent segment disease” (ASD) remains poorly defined, it is clear that ASD is one of the most dreaded long-term clinical sequelae after successful fusion. From biomechanical investigations and clinical radiographic studies, it appears that there is an alteration of load sharing with an increase in mobility, shear, strain, and pressure at the intervertebral disk, uncovertebral joints, and facet joints of the adjacent segment (s) after rigid spinal fusion.13,15 With this in mind, many postulate that preservation of either or both motion and load sharing at the index pathological level would help to mitigate or reduce the overall incidence of ASD. In the hope of decreasing adjacent segment forces, total anterior disk replacement (TDR) devices such as the SB Charité III (DePuy Spine, Raynham, MA) and the ProDisc II (Synthes, West Chester) were developed in an attempt to preserve motion at the etiologic intervertebral disk. From the clinical Federal Food and Drug Administration (FDA) Investigational Device Exemption (IDE) study, the SB Charité III was able to provide equivalent relief of low back pain as compared with the randomized control arm of anterior fusion.2,16 However, numerous authors have cited that severe facet arthropathy, spinal stenosis, neurogenic claudication, significant canal disease, spondylolisthesis, or translational instability are all relative or absolute contraindications to placement of an anterior TDR.11,17 In a study by Huang examining the typical makeup of patients seen in a tertiary spinal clinic, there was a preponderance of such patients with dorsal disease, spinal stenosis, spondylolisthesis, and/or spinal instability. These patients were ideally suited for classical spinal decompression and, in many cases, posterior spinal fusion and were not candidates for TDR.11 As such, it is clear that motion-preserving devices that can be used for patients requiring dorsal surgical treatment are needed. When we examine the issue of posterior spinal disease and spinal stenosis, it is clear that we face not only the natural history of the disease process but also the iatrogenic instability that results from surgical decompression of these patients. Because a large majority of these patients are symptomatic from radicular or central canal compression, they require decompression of the paramedian lamina and at least the medial third or medial half of the facet complex. As a result, progressive resection for neural decompression can lead to progressive spinal instability in cases where the facet orientation is more sagittal than coronal.1 In many patients with spinal stenosis who require aggressive decompression for extensive neural foraminal narrowing, spinal fusion is often necessitated after facet resection.5,18 In Resnick et al. Fischgrund et al’s analysis, patients with spondylolisthesis and stenosis overall did better with regard to their low back pain scores when they had a primary fusion in addition to decompression as opposed to those who had decompression alone.6,7 However, it is also clear that many patients with either or both stenosis and stenosis with spondylolisthesis do well without fusion and do not go on to have gross or glacial spinal instability after decompressive surgery. As such, a motion-preserving technology that can be placed via a standard posterior approach can help to avoid fusion in the many stenotic patients who are either preoperatively only mildly unstable or made unstable after surgical decompressive destabilization of the facet complex. With this in mind, the question remains as to the ideal nature of such a posterior motion-preserving stabilizer of the spine. Whereas numerous theories regarding the etiology of low back pain exist, perhaps the most developed of these is the concept of the biomechanical neutral zone as postulated by Panjabi.19,20 In this useful heuristic system, a motion segment functions to share load and to move and impinge within a given set of mechanical parameters. During biomechanical testing, any given spinal motion segment will thus move a given amount per quantum of applied load as determined by the viscoelastic properties of the surrounding structures that bind the two vertebrae such as the intervertebral nucleus and annulus, facet joint and capsule, interspinous ligaments, spinal longitudinal ligaments, attached paraspinal muscles, and truncal musculature. Plotted in any of three dimensions, this leads to a classic load-displacement plot of the “neutral zone” (Fig. 45–1). Degeneration, acute injury, or other pathology thereby alters the biomechanical limiters of the system thereby leading to laxity, altered load sharing, and a widening of the load-displacement curves and altering of the neutral zone itself. As movement of the spinal segment begins to exceed its initial “setpoints,” joint, nociceptive, and stretch receptors begin to activate and signal pain and injury in the area, which may lead to progressive pain and inflammation in that area. As such, induced injury of the ligaments or facets or disk complex will lead to a widened neutral zone as seen on load-displacement curves during biomechanical cadaveric testing (Fig. 45–1B). This model thus provides a useful point of reference as to the cause of mechanical back pain in patients. Whereas decompression will help to relieve radicular pain, surgical restoration of proper load sharing and normalization of the neutral zone may help to decrease pain and inflammatory stimuli in the treated spinal segment (s). As such, the efficacy of rigid spinal fusion may ultimately result from its ability to radically correct the load-displacement characteristics of the motion segment to near-zero movement for any applied load after rigid fusion and instrumentation. With this in mind, a proper motion-preserving stabilizer device must also be able to correct the load-displacement curves back to an anatomically natural neutral zone while still preserving some degree of native spinal mobility above that of rigid fusion. Additionally, by preserving load sharing of the treated segment, it must also decrease the “stress-riser” effect on adjacent untreated levels to potentially minimize the incidence of ASD. Finally, the motion-preserving dorsal device must also be secured to the spine in such a way that the device–bone interface remains stable over several million cycles. For example, devices that are to be implanted through the vertebral pedicles must thereby minimize the stress at the screw–bone to prevent screw pullout. As such, the motion-preserving device must not only be able to provide motion but must also do so in a way that does not load the screws in any significant way. Figure 45–1 Mechanical properties of the intact and injured spine. ROM, range of motion. TOPS is a unitary device (Fig. 45–2) composed of a titanium “sandwich” with an interlocking polycarbonate urethane (PcU) articulating construct. The flexible PcU elements within the construct allow relative movement between the titanium plates so as to create axial rotation, lateral bending, extension, and flexion. The internal construct mechanically restricts motion to 1.5 degrees of axial rotation, 5 degrees of lateral bending, 2 degrees of extension, and 8 degrees of flexion. The implant also blocks excessive posterior and anterior sagittal translation. The TOPS system uses four standard hydroxyapatite (HA)-coated polyaxial pedicle screws for fixation to the vertebrae (Fig. 45–3). Because the internal configuration of the PcU bumpers ultimately acts as the limiter of motion, the TOPS device has an inherent dampening property, which serves to dissipate energy that is passed through it during standard load sharing of the moving spinal motion segment. Furthermore, because the PcU elements also have some “shock-absorption” properties in the vertical axis, vertical load transmitted through the cross-bars through the centroid of the device is also somewhat dampened as well. These features not only serve to allow for near full spinal motion but also to decrease stresses at the adjacent levels and also at the screw–bone interface. Figure 45–2 TOPS is a unitary device composed of a titanium “sandwich” with an interlocking polycarbonate urethane articulating construct. TOPS provides patients suffering from degeneration/hypertrophy of the facet joint, grade I degenerative spondylolisthesis, and spinal stenosis with three major advantages. The surgeon can perform a wide decompression to eliminate the pain generators. The procedure stabilizes the posterior spine. The procedure allows a controlled range of motion. The implanted device, made of flexible materials and titanium, allows for constrained bending, straightening, and twisting movements in the affected segment postsurgery. As such, the TOPS device serves to achieve the goals already detailed for restoring the physiological neutral zone, maintaining a degree of spinal motion over rigid fusion, decreasing abnormal load sharing at the adjacent levels, and minimizing screw–bone interface stresses through the dampeners of the PcU elements contained therein. As part of the overall development program on early designs of the TOPS device, a finite element analysis (FEA) was performed on the implant using ANSYS computational software (Ansys, Inc., Canonsburg, PA). The original model developed for this theoretical stress analysis was a half-section representation of the device. The model was chosen because the device itself and the loading conditions on it were found to be symmetric about the central plane. This hemimodel analysis allowed for faster computation without loss of precision. The results of this assessment show the principal stresses acting on the model as a result of the applied loading. The principal stress generated in the device during maximum anticipated loading is well below the yield stress for the titanium alloy from which it is fabricated21 (Fig. 45–4).
TOPS—Total Posterior Facet Replacement
and Dynamic Motion Segment
Stabilization System
Rationale for Posterior Facet Replacement and Arthroplasty
Surgical Technique for TOPS Posterior Facet Replacement System Implantation
Clinical Data and Outcomes of TOPS Facet Replacement
Rationale for Posterior Facet Replacement and Arthroplasty
Total Posterior Facet Replacement System (TOPS) Implant Characteristics
Design Parameters
Finite Element Analysis
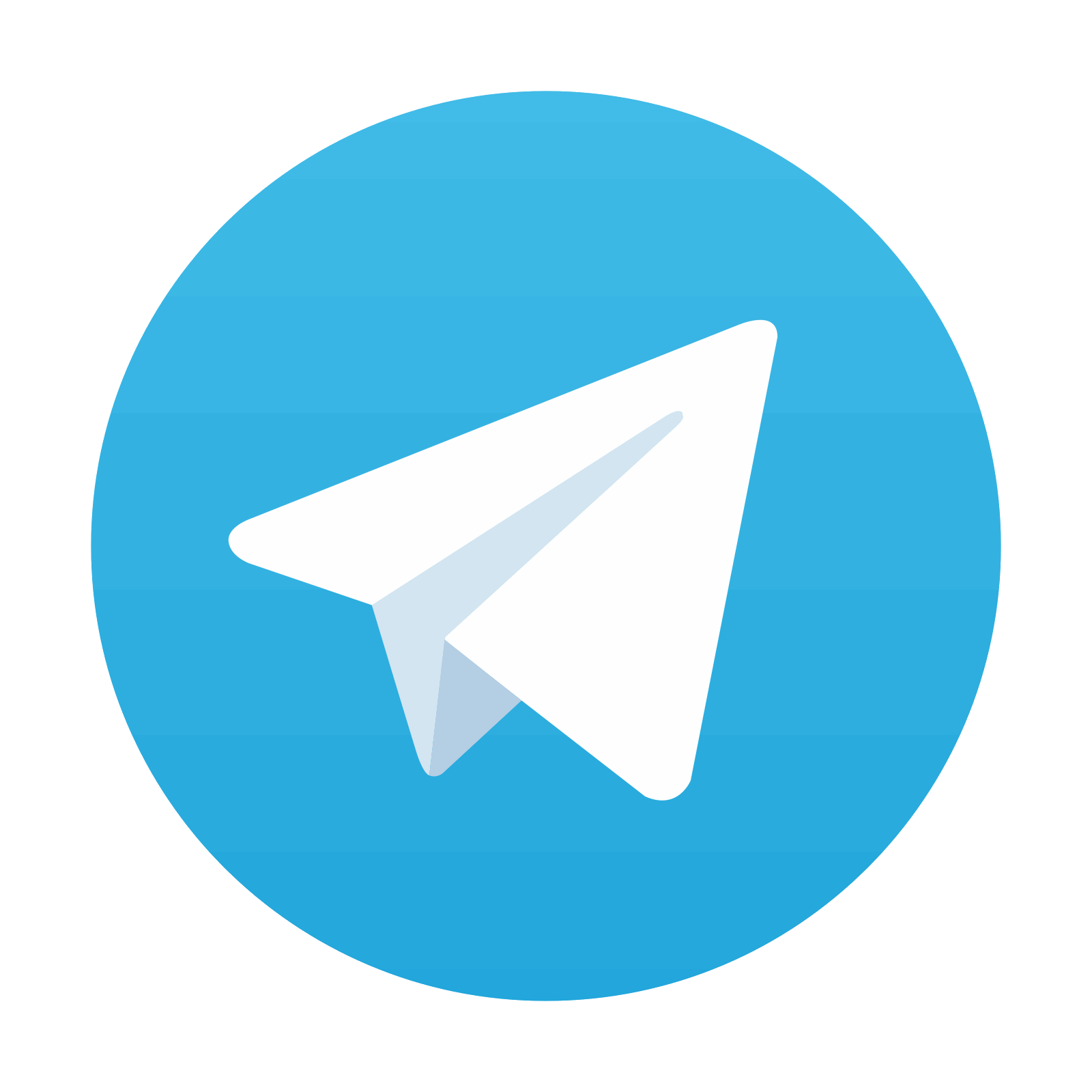
Stay updated, free articles. Join our Telegram channel

Full access? Get Clinical Tree
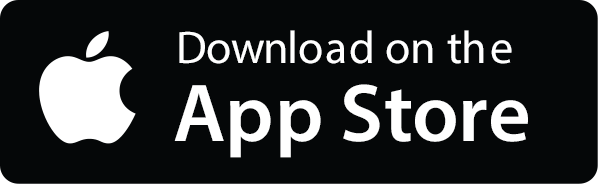
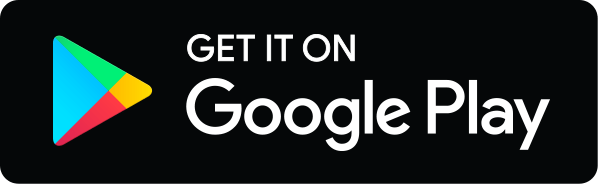