Fig. 5.1
Schematic illustration of the follicular penetration pathway which has to be divided into two steps: (1) intrafollicular penetration and (2) transfollicular penetration if allowed due to size reasons
In comparison to the intercellular penetration process, topical drug delivery via the hair follicles provides additional features such as fast delivery into the systemic circulation if transfollicular penetration is applicable (Otberg et al. 2008) as well as long-term intrafollicular storage (Lademann et al. 2006) if transfollicular penetration cannot be realized. These and more aspects will be discussed in this chapter.
5.2 Architectural and Physiological Features of the Hair Follicle with Regard to Follicular Penetration
Due to its complex and dynamic architectural structure, the hair follicle is predestined as a penetration and storaging organ, although the original functions of the hair follicle seem to be rather those of a sensory organ, sebum excretion and protection (Krause and Foitzik 2006). The infundibulum is the upper part of the hair follicle and consists of an upper and lower portion as depicted in Fig. 5.2. The epithelium of the upper infundibulum is continuous with the keratinized epidermis and covered by an intact stratum corneum, whereas the differentiation pattern of the lower infundibulum switches from epidermal to trichilemmal leading to an interrupted skin barrier with only few differentiated corneocytes remaining (Blume-Peytavi and Vogt 2011) that are more prone to transfollicular penetration in this region. Moreover, the whole follicular infundibulum is supplied by a dense capillary network and surrounded by a high number of immune cells, on the one hand allowing the rapid systemic uptake of substances once penetrated transfollicularly and, on the other hand, rendering the hair follicle to be a promising target for immune therapy or topical vaccination (Patzelt et al. 2011; Vogt et al. 2008).


Fig. 5.2
Schematic overview of the follicular architecture especially depicting the structures relevant to the follicular penetration process such as the infundibulum surrounded by the blood capillaries and dendritic cells, the sebaceous gland, and the bulge region with stem cells
Additionally, as depicted in Fig. 5.2, also the sebaceous gland and the bulge region of the hair follicle which is hosting the stem cells are interesting structures within the hair follicle, at the same time being attractive targets for therapeutic interventions (Patzelt et al. 2011). The sebaceous gland is the organ of sebum excretion and additionally associated with a diversity of pathologies such as acne (Thiboutot 2004). It was suggested that by increasing the distribution of corresponding drugs in the sebaceous gland, the therapeutic effectivity could be significantly improved (Rolland et al. 1993). Respective efforts have already been made (Morgan et al. 1993; Ridolfi et al. 2012; Rolland et al. 1993), and some promising antiacne products are already on the market. The multipotent, highly proliferative, and easily accessible stem cells located in the bulge region (Ohyama 2007) are also to be integrated in the therapeutic concepts for cutaneous regenerative medicine or gene correction of congenital hair disorders or genetic skin diseases.
In total, more than 20 different cell types are involved in the structure of the hair follicle which underlies cyclical activity (Rogers 2004). Three different hair follicle types, namely, lanugo, vellus, and terminal hair follicles, have to be distinguished. Whereas the same hair follicle produces lanugo hairs in the fetal period and vellus hairs in the childhood, it produces terminal hairs in the adulthood (Blume-Peytavi and Vogt 2011). In total, each human individual displays an estimated number of five million hair follicles (Krause and Foitzik 2006) underlining its potential in the penetration process. The morphometry of vellus and terminal hair follicles has been well documented already (Vogt et al. 2007). A schematic overview of the follicular architecture is depicted in Fig. 5.2.
5.3 Mechanisms of Follicular Penetration and Transfollicular Penetration
5.3.1 Mechanisms of Follicular Penetration
The follicular penetration of topically applied substances represents a complex process which has not been clarified in detail until now. Whereas the retention of the particles in the follicular duct has been well documented, researchers are controversially discussing which substances are able to penetrate transfollicularly into the deeper skin layers, whereby the size next to other physicochemical properties seems to be the predominant parameter (Labouta and Schneider 2013). In the last years, predominantly particulates such as liposomes and micro- and nanoparticles have attracted attention as a result of their capability to improve penetration into the hair follicle. Here, a clear size dependency could be observed demonstrating that 320 nm sized polymer particles covalently labeled with a fluorescent dye penetrated significantly deeper into the hair follicles than the same fluorescent dye in non-particulate form (Lademann et al. 2007). The optimum size for particles to penetrate deeply into the hair follicle was determined to be in the range of 400–700 nm, whereas larger and smaller particles reached significantly lower penetration depths (Patzelt et al. 2011) or remained even on the skin surface in the case of very large particles (Schaefer and Lademann 2001; Toll et al. 2004). As this effect was demonstrated for different solid particle preparations such as PLGA particles and silicium oxide particles, it was assumed that follicular particle penetration is a predominantly mechanical effect independently from the particle preparation (Patzelt et al. 2011). In Fig. 5.3, the dependency of the penetration depth on the particles’ size is schematically represented. Lademann et al. (2009) hypothesized that the surface structure of the hair and the hair follicle, which is determined by the thickness of the keratin cells in the cuticula, being 530 nm in human hairs and 320 nm in porcine hairs, might act as a pumping system delivering the particles deeply into the hair follicle as demonstrated in Fig. 5.4. Whereas the movement of the hair occurs physiologically in vivo, it could be shown that this effect can be simulated in vitro by massage appliance (Lademann et al. 2007; Patzelt et al. 2011).



Fig. 5.3
Dependency of the penetration depth of the particles on the particles’ size. Large particles (>3 μm) are only located on the skin surface and in the follicular orifice. Particles between 400 and 700 nm penetrate significantly deeper into the hair follicles than larger or smaller particles. A size threshold below which transfollicular penetration occurs has not been determined, yet

Fig. 5.4
Schematic cross section of a hair illustrating the specific structure of the cuticula cells. It has been hypothesized that this surface structure which is determined by the thickness of the cuticula cells might act as a pumping system delivering the particles deeply into the hair follicles when cuticula thickness and particle size are similar
Once penetrated into the hair follicles, substances are stored over several days (Lademann et al. 2006) within this protected area if transfollicular penetration is not feasible. Whereas the reservoir of the stratum corneum is relatively unprotected and thus easily depleted by daily processes such as textile or water contact in combination with the physiological desquamation process removing one layer of corneocytes per day, the hair follicle represents a protected reservoir which can only be depleted by such slow outward-directed processes as sebum flow and hair growth. Previous investigations could show that a particle-containing formulation was still detectable within the hair follicle after 10 days, whereas the stratum corneum reservoir had already been almost completely depleted after 1 day (Lademann et al. 2006) as presented in Fig. 5.5. This long-term storage effect could be effectively utilized for therapeutic purposes as the application of drug-loaded particles with retarded release could diminish the application frequency and thus increase the compliance of patients and the therapeutic outcome.


Fig. 5.5
The hair follicle is a long-term reservoir for topically applied substances. Directly after application, the applied particles are distributed on the skin surface and within the hair follicle. After 1 day, the stratum corneum reservoir is significantly depleted, whereas the particles are still available within the hair follicle. After 10 days, part of the particles are still detectable within the hair follicle
This therapeutic effectivity could even be enhanced when the correct skin site is chosen for the application. Otberg et al. (2004b) measured the follicular density, the volume of the follicular infundibula per square centimeter of skin, and the surface of the follicular infundibula per square centimeter of skin. The latter corresponds to the additional absorption area provided by the hair follicles. They could show that the forehead and the calf regions had the highest follicular volume per square centimeter of skin surface which was explained by the high follicle density on the forehead and the large hair follicles on the calf. It was estimated that the reservoir volumes of the hair follicles and of the stratum corneum were comparable in these body regions as demonstrated in Fig. 5.6, whereas the reservoir of the hair follicles in the region of the forearm was significantly lower by a factor of 20 in comparison to the stratum corneum reservoir.


Fig. 5.6
The largest follicular reservoir can be found on the calf and the forehead. Here, the follicular reservoir is comparable to the stratum corneum reservoir
The investigation of follicular penetration still represents a challenge as it requires spatial resolution. Nowadays, several methods are available to investigate follicular penetration reasonably. These methods have been recently summarized by Meidan et al. (2010) and include, inter alia, the selective artificial closing technique, where the hair follicles are selectively blocked with a varnish-wax mixture and thus excluded from the penetration process (Teichmann et al. 2006), the usage of a sandwich model (Barry 2002), where the top skin layer blocks the shunts in the bottom layer or the differential stripping method (Teichmann et al. 2005). Further novel optical devices are, e.g., autoradiography (Fabin and Touitou 1991), confocal laser scanning microscopy (Lademann et al. 2010) or combined confocal laser scanning microscopy with confocal Raman spectroscopy (Caspers et al. 2003).
Next to methodological challenges, also the selection of adequate model systems plays a superior role as could be demonstrated recently. Patzelt et al. (2008) could demonstrate that for experiments performed on the same volunteers and each on the contralateral skin site, the in vitro follicular reservoir was only 10 % of the in vivo follicular reservoir. It was assumed that the elastic fibers surrounding the hair follicle contract during the excision. Whereas the removed skin sample can be re-stretched to its original size by expanding the interfollicular elastic fibers, those surrounding the hair follicle remain contracted and reduce the follicular reservoir significantly. Based on these observations, it can be stated that excised skin is not an appropriate model to investigate follicular penetration. However, in vivo investigations are not always feasible as substances or methods are mostly too injurious. As a result, the porcine ear model has been determined to be a suitable ex vivo model as the full skin can remain fixed on the underlying cartilage during the experiments, and the good and well-documented similarities of porcine and human skin architecture and structure allow a reasonable evaluation of the obtained data (Lademann et al. 2010).
In this context, it has to be emphasized that full skin samples including the subcutaneous tissue are very important for follicular penetration investigations. For diffusion cell experiments, however, mostly split skin or epidermal skin is utilized where at least the subcutaneous tissue is discarded meaning that the lower part of the hair follicle which reaches deeply into the subcutaneous tissue is cut off. This means that the inferior part of the hair follicle is open and the topically applied substances can diffuse directly into the receptor medium as demonstrated in Fig. 5.7. Similar concerns have already been raised by Senzui et al. (2010).


Fig. 5.7
Removing the subcutaneous tissue leads to a violation of the distal hair follicle. If, as usual, only the upper part of the skin is utilized for diffusion cell experiments, the topically applied substance can just diffuse into the receptor medium via the open inferior part of the hair follicle. Thus, the utilization of diffusion cell experiments is not an appropriate method to investigate follicular penetration
In addition to physicochemical properties of the applied substance and the pumping effect transporting the substances into the hair follicle by hair movement, also the activity status of the hair follicles seems to play an important role and decides whether or not follicular penetration occurs at all. As mentioned earlier, each hair follicle undergoes continuous cycling, which includes the complete remodeling of its non-permanent portion and which influences the hair growth and sebum excretion activity of each hair follicle. A previous study could demonstrate that it can be distinguished between active hair follicles, which provide hair growth and/or sebum excretion and are open for penetration, and inactive hair follicles which provide neither hair growth nor sebum flow and are thus inaccessible for topically applied substances (Otberg et al. 2004a). For these inactive follicles a cover consisting of dried sebum and desquamated corneocytes was detected in the follicular orifices that prevents any penetration process. In the forearm region, one fourth of the hair follicles were shown to be unreceptive for penetration.
5.3.2 Mechanisms of Transfollicular Penetration
Transfollicular penetration of topically applied substances and mechanisms of transfollicular penetration have not been fully clarified, so far. It can be assumed, yet, that transfollicular penetration occurs mainly in the region of the infundibulum and specifically in the lower portion of the infundibulum where the barrier is interrupted due to the switch of a differentiation pattern as mentioned above and depicted in Figs. 5.1 and 5.3. For smaller non-particulates, i.e., substances such as caffeine or minoxidil, a rapid transfollicular penetration and systemic uptake has already been reported (Blume-Peytavi et al. 2010; Otberg et al. 2008). Interestingly, the systemic uptake was significantly faster (already after 5 min) when the hair follicles were accessible in comparison to skin areas where the hair follicles were selectively blocked previously. Here, the substances needed approximately 20 min to be detectable in the circulation. For particles the situation seems to be different, yet. There are clear indications that the transfollicular penetration process is predominantly determined by the size of the applied particles, although a clear size threshold for transfollicular penetration could not be defined so far. Recently, Labouta and Schneider (2013) reviewed the current literature focusing on skin penetration of inorganic particles. It could be shown that about half of the studies reported particle penetration or permeation. However, most studies’ protocols involved either mechanical or chemical penetration enhancers (Dixit et al. 2007; Krishnan et al. 2010; Labouta et al. 2011; Mortensen et al. 2008; Paliwal et al. 2006; Upadhyay 2006; Zhang and Monteiro-Riviere 2008) or utilized excised either animal or human skin in in vitro diffusion cell experiments. With regard to risk assessment or mechanistic investigations concerning transfollicular penetration, the utilization of penetration enhancers or of in vitro diffusion cell experiments seems to be not reasonable as described above as overestimation of particle penetration can occur if penetration is either artificially enhanced or investigations are performed with dermatomed or split skin which always includes a violation of the distal hair follicle so that topically applied substances can just diffuse into the receptor medium. Whereas the contraction effect of the elastic fibers surrounding the hair follicle assumed by Patzelt et al. (2008) might be able to inhibit the diffusion of larger particles, it might explain that the detected diffusion of very small particles into the receptor medium is erroneously interpreted as penetration. This theory is supported by the fact that for all human in vivo studies reported by Labouta and Schneider (2013), no particle penetration or permeation could be detected. Only some studies were performed in vivo on animal skin, and within this group, only few studies reported a penetration into deeper skin layers of very small particles. The authors assumed that the gold nanoparticles utilized in their study might interact hydrophobically with the skin lipids leading to a disruption of the skin lipid layer structure, subsequent increased skin permeability and penetration into deeper skin layers (Huang et al. 2010). This assumption still needs further verification.
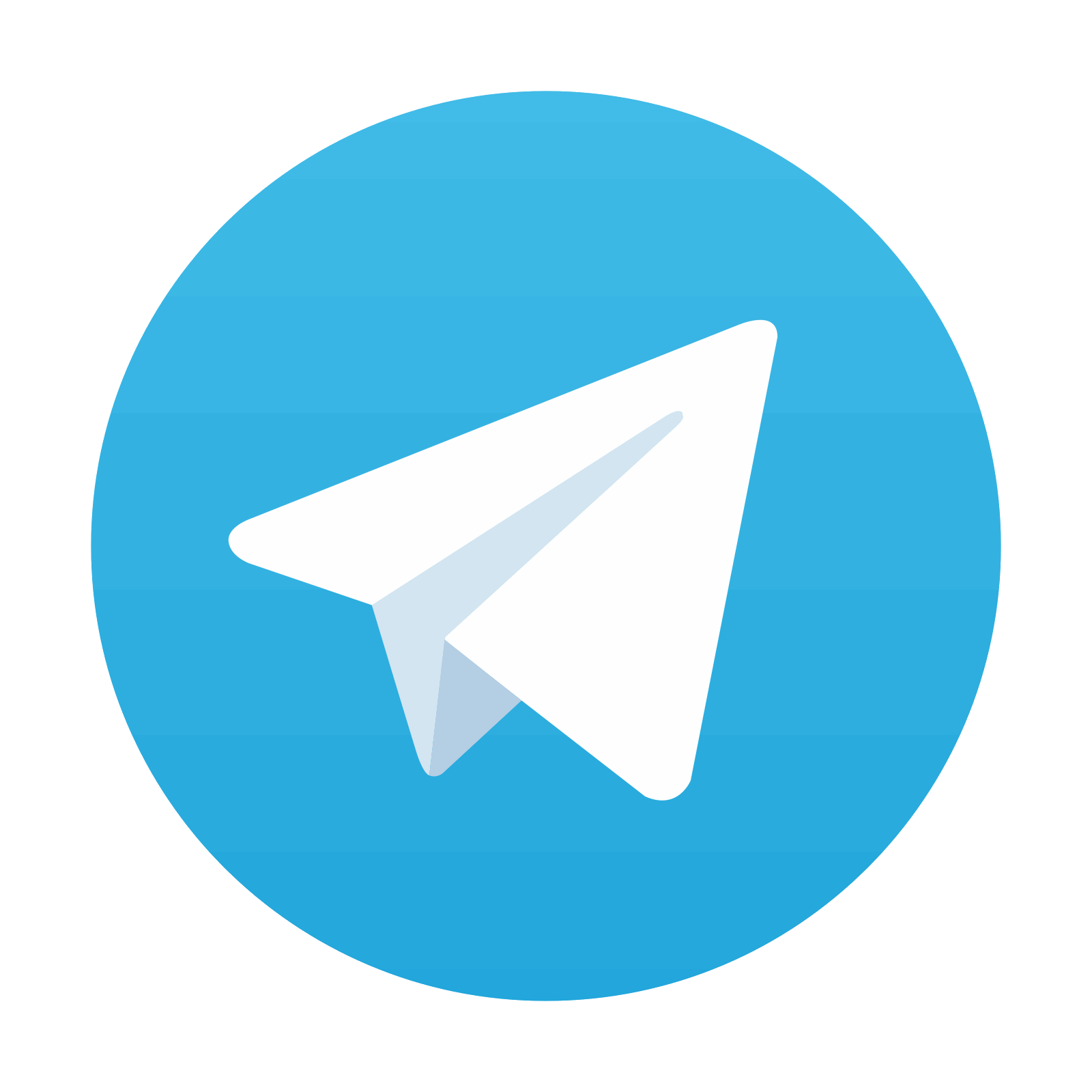
Stay updated, free articles. Join our Telegram channel

Full access? Get Clinical Tree
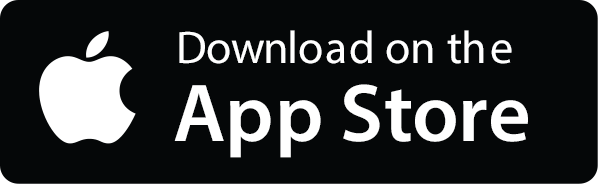
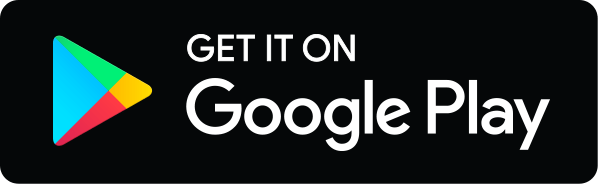