(1)
Dermatologische Praxis & Haarcenter, Wallisellen (Zürich), Switzerland
Wise to resolve, and patient to perform.
Homer
Clinical trichology should represent an integral part of medical training, and the dermatologist participates with the other medical disciplines in the diagnosis and treatment of all types of hair problems relating to systemic disease. On the other hand, hair loss is an important cause of discomfort and disability. The general physician is not always aware of the significance of hair loss and therefore may fail to refer patients with hair disorders to the dermatologist for appropriate management. Too often, the delay of correct diagnosis, and as a result the delay of appropriate therapy, leads to potentially irreversible loss of hair, prolongs the discomfort, and promotes the disfigurement. Knowledge of the main types of hair loss is prerequisite to providing appropriate patient care.
4.1 Congenital Atrichia and Hypotrichosis
By definition, alopecia is the acquired condition of recognizable hair loss. The term derives from Greek with reference to the loss of hair in patches in foxes afflicted with sarcoptic mange. In case of congenital absence or lack of hair, the correct terms are atrichia and hypotrichosis, respectively: While congenital atrichia is characterized by a total and permanent absence of hair, in congenital hypotrichosis the hair is diffusely thinned, but present.
Hereditary and congenital hypotrichosis and atrichia are among the most complex areas of disorders of hair growth with several distinctive entities occurring either as an isolated defect or as a feature of a complex hereditary syndrome in association with other ectodermal defects (ectodermal dysplasia).
In 1998, Angela Christiano and coworkers reported the identification of a missense mutation in the zinc finger domain of the hairless (HR) gene (arg620 to gln) on chromosome 8p21.3 underlying (congenital universal) atrichia with papular lesions. Almost complete absence of hair associated with papular lesions over most of the body are features of the disorder (Fig. 4.1a). Patients are born with hair that falls out and is not replaced. Histological studies show cystic malformation of the hair follicles. Although the identification of the HR gene has been shown not to be relevant with respect to the most frequent cause of hair loss and its treatment, androgenetic alopecia and alopecia areata, published estimates of the prevalence of congenital atrichia with papular lesions remain surprisingly low considering pathogenetic mutations of the HR gene have been found in distinct populations around the world.


Fig. 4.1
(a–d) Congenital atrichia and hypotrichosis: (a) Congenital universal atrichia with papular lesions. (b) Hereditary hypotrichosis simplex in father and daughter. (c) Marie Unna hereditary hypotrichosis, coarse hair, (d) and initial vertex alopecia
Some experts have assumed that congenital universal atrichia might be more common than previously thought and frequently mistaken for the autoimmune form of alopecia (areata) universalis and suggested that infants with presumed autoimmune alopecia universalis may warrant testing for HR gene mutations, particularly before embarking on therapeutic modalities that will fail.
Hereditary hypotrichosis simplex is a rare form of hereditary hypotrichosis with no associated abnormality or hair shaft anomaly. Affected individuals typically show normal hair at birth, but diffuse hair loss and thinning of the hair shaft start during early childhood and progress with age (Fig. 4.1b).
In contrast, Marie Unna hereditary hypotrichosis is distinguished by the presence of a peculiar twisting hair dystrophy. Characteristically coarse, wiry, twisted hair develops in early childhood and is followed by the development of alopecia beginning in the crown area (Fig. 4.1c, d).
Monilethrix represents another monogenic disorder presenting with hair loss. As opposed to hereditary hypotrichosis, loss of hair is due to a hair shaft abnormality with fragility and breakage. It is due to a disorder of hair cortex keratin resulting in brittle hair that tends to fracture and produce varying degrees of alopecia. Individuals with monilethrix have normal hair at birth but, within the first few months of life, sometimes later, develop alopecia. In the mildest forms, only the occipital regions of the scalp are involved, due the friction; however, in severe forms the eyebrows, eyelashes, and secondary sexual hair may also be involved. Follicular hyperkeratosis with predilection for the nape of neck is a consistent feature that is particularly helpful for initially suspecting the diagnosis (Fig. 4.2a). Light microscopic examination is diagnostic and reveals elliptical nodes of normal thickness and intermittent constrictions at which the hair easily breaks (Fig. 4.2b). There may be spontaneous improvement with time, especially during puberty, but the condition never resolves completely.


Fig. 4.2
(a, b) Monilethrix. (a) Follicular hyperkeratosis in the nape of the neck. (b) Dermoscopy: elliptical nodes of normal thickness and intermittent constrictions
Localized autosomal recessive congenital hypotrichosis is yet one more form of monilethrix-like, congenital hypotrichosis caused by mutation in the desmoglein 4 gene that has more recently been recognized. The clinical presentation is more severe than in the autosomal dominant form of monilethrix, with more extensive alopecia of the scalp, and body, and a papular rash involving the extremities and periumbilical region.
Associated abnormal features of congenital atrichia or hypotrichosis (with or without hair shaft abnormality) may include dysplastic or brittle fingernails, skin and tooth defects, or other abnormalities that have to be sought for systematically (for an extensive list, see Online Mendelian Inheritance in Man).
Typical examples include X-linked recessive anhidrotic ectodermal dysplasia of Christ–Siemens–Touraine (Fig. 4.3a) and hidrotic ectodermal dysplasia of Clouston (Fig. 4.3b, c). Also, the Rapp–Hodgkin/Hay–Wells syndrome shows characteristic features with coarse and sparse scalp hair, chronic inflammatory dermatitis of the scalp, midfacial hypoplasia, and cleft lip/palate (Fig. 4.3d), and the trichorhinophalangeal syndrome with fine and sparse scalp hair, a pear-shaped broad nose, and cone-shaped epiphyses of the middle phalanges (Fig. 4.4a, b).



Fig. 4.3
(a–d) Ectodermal dysplasia: (a) Christ–Siemens–Touraine type (anhidrotic) with typical facies (frontal bossing, midfacial hypoplasia, saddle nose, large chin, and thick lips). (b) Clouston-type (hidrotic), with (c) associated nail abnormality (milky white in childhood, and gradually become dystrophic, thick, and distally separated from nail bed) (d) Rapp–Hodgkin syndrome, with coarse hair and midfacial hypoplasia

Fig. 4.4
(a, b) Trichorhinophalangeal syndrome. (a) Fine and sparse scalp hair, pear-shaped nose. (b) Cone-shaped epiphyses of the middle phalanges
While in many of the congenital disorders of hair growth and/or structure, little more can be done than making the diagnosis and giving advice on genetic counseling and coping strategies, in others the genetic hair disorder may be a marker of more serious associated anomalies.
Examples are congenital sensorineural deafness associated with pili torti in Bjornstad syndrome and arrhythmogenic or dilated cardiomyopathy associated with woolly hair and palmoplantar keratoderma in Naxos disease and Carvajal syndrome, respectively, to name but a few.
4.2 Challenges in Non-scarring Alopecia
In daily clinical practice, we are usually dealing with the acquired disorders of hair growth and cycling.
The non-scarring alopecias represent the most frequent cause of acquired hair loss.
While androgenetic alopecia (75 %), telogen effluvium (10 %), and alopecia areata (10 %) represent the most common causes in the adult population, alopecia areata (60 %), tinea capitis (20 %), and trichotillomania (10 %) are among the most frequent in children (in brackets are frequencies determined at the Hair Consultation Clinic of the Department of Dermatology, University Hospital of Zurich, between 1999 and 2010).
4.2.1 Androgenetic Alopecia
Androgenetic alopecia is the single most frequent cause of male and female hair loss. It is understood to represent a hereditary and androgen-dependent, progressive thinning of the scalp hair that follows a defined pattern, though there exist significant differences with respect to frequency, age of onset, and pattern of alopecia between male and female androgenetic alopecia. Androgenetic alopecia affects at least 50 % of men by the age of 50 years and up to 70 % of all males in later life, while estimates of its prevalence in women have varied widely. Recent studies claim that 6 % of women aged under 50 years are affected, increasing to a proportion of 30–40 % of women aged 70 years and over. While male pattern androgenetic is characterized by its typical bitemporal recession of hair and balding vertex, female pattern androgenetic alopecia is set apart by its diffuse thinning of the crown and intact frontal hairline. Nevertheless, 4 % of males may present with the female pattern of androgenetic alopecia, while 13 % of premenopausal women and 37 % of postmenopausal women present with the male pattern of androgenetic alopecia. Androgenetic alopecia that is clinically manifest between the ages of 10 and 20 is called premature alopecia or alopecia praecox. Before puberty it presents in both females and males exclusively with the female pattern.
The diagnosis and treatment of androgenetic alopecia are relatively straightforward and easy, with 2 or 5 % topical minoxidil solution in men and in women and 1 mg oral finasteride in men having excellent evidence levels for their therapeutic use in androgenetic alopecia (either randomized, double-blind, comparative clinical studies of high quality, e.g., sample size calculation, flow chart of patient inclusion, ITT analysis, sufficient size, or meta-analysis, which includes at least one randomized double-blind, comparative clinical studies of high quality).
Minoxidil promotes hair growth through increasing the duration of anagen. It causes hair follicles at rest to grow and enlarges suboptimal follicles. Minoxidil was serendipitously discovered to be a hair growth-promoting agent, when patient taking the compound orally for treatment of arterial hypertension developed generalized hypertrichosis. Originally developed for treatment of androgenetic alopecia in men, topical minoxidil proves to be more effective in female androgenetic alopecia.
While the drug’s action as a vasodilating agent is best understood, its mechanism of action on hair growth remained unclear. Only recently, with the discovery of the mutation underlying hypertrichotic osteochondrodysplasia or Cantu’s syndrome, light has been shed on the molecular basis of the action of minoxidil. In Cantu’s syndrome, the hypertrichosis leads to thick scalp hair extending onto the forehead and a general increase in body hair. It is caused by a mutation in the ABCC9 gene that codes for sulfonylurea receptor 2, involved in ATP-sensitive potassium channels, while minoxidil has been understood to be a potassium-channel opener, with evidence that this effect is mediated by sulfonylurea receptor 2B. Both the vasorelaxant and hair growth-promoting effects of minoxidil are due to the actions of its sulfated metabolite, minoxidil sulfate. Minoxidil is sulfated by a group of enzymes known as sulfotransferases, some of which are expressed in the hair follicle with wide interindividual variations in the level of enzyme activity.
Recent studies have proposed that enzymatic assay of sulfotransferase activity in plucked hair follicles may predict response to topical minoxidil in the treatment of androgenetic alopecia. A subsequent analysis confirmed the clinical utility and validity of a sulfotransferase enzyme test in successfully ruling out 95.9 % of nonresponders to topical minoxidil for the treatment of androgenetic alopecia.
Finasteride is a competitive inhibitor of type 2 5-alpha reductase and inhibits the conversion of testosterone to dihydrotestosterone (DHT). The rationale for the use of finasteride to treat androgenetic alopecia in men is based on the absence of alopecia in men with congenital deficiency of type 2 5-alpha reductase and the presence of increased 5-α reductase activity and DHT levels in balding scalp. While oral finasteride has unanimously been shown to be effective in treatment of hair loss in men, its efficacy in women remains controversial: In a double-blind, placebo-controlled, multicenter trial, Price et al. demonstrated that oral finasteride, 1 mg/day, taken for 1 year did not slow progression of hair loss or promote hair growth nor improve follicular counts in horizontal sections of scalp biopsies in postmenopausal women with androgenetic alopecia. One explanation might be that the different pattern of hair loss in the majority of women from that usually seen in men may be due to differences in the relative levels of 5-α reductase, aromatase, and androgen receptors in scalp hair follicles in women compared with those in men. Thai and Sinclair were the first to report successful oral finasteride treatment of androgenetic alopecia in a postmenopausal woman with androgen levels within normal values. Trüeb et al. also reported successful treatment of androgenetic alopecia with 2.5 or 5 mg/day oral finasteride in 5 normoandrogenic, postmenopausal women. Improvement with growth of hair was observed as early as 6 months of therapy, irrespective of the pattern of hair loss. Efficacy was evaluated by patient and investigator assessments and review of photographs taken at baseline and at months 6, 12, and 18 by an expert panel. Finasteride treatment improved scalp hair by all evaluation techniques. The patients’ self-assessment demonstrated that finasteride treatment decreased hair loss, increased hair growth, and improved appearance of hair. These improvements were confirmed by investigator assessment and assessments of photographs. No adverse effects were noted. Shum et al. reported four cases of hair loss with characteristics of both male and female pattern hair loss in women with hyperandrogenism, in which finasteride improved the alopecia. Their patients differed from those in the trial reported by Price et al. in that the patients had increased androgen levels, and finasteride was used in a slightly higher dose (1.25 mg/day) and given for a longer period of time (24–30 months as opposed to 1 year). On the other hand, Carmina and Lobo did not find finasteride, 5 mg/day, to be effective in treatment of alopecia in hyperandrogenic women. In the so far largest series of 37 premenopausal women treated for 1 year with finasteride in doses of 2.5–5 mg daily, Iorizzo et al. showed some improvement in 62 % as assessed by global photography.
Differences in response of women to oral finasteride have led to the suggestion that not all types of female hair loss have the same pathophysiology, i.e., a distinction should be made between alopecia with early (premenopausal) or late (postmenopausal) onset and with or without hyperandrogenemia.
In an attempt to identify predictive factors for response of women with androgenetic alopecia to oral finasteride therapy, Keen and Goren aimed at analyzing the variant repeat nucleotide sequences in exon 1 of the androgen receptor (AR) gene that have been shown to determine androgen sensitivity in a variety of androgenic conditions in men and women. In a 6-month pilot study of 13 patients, women with greater androgen sensitivity (<24 cytosine, adenine, and guanine (CAG) repeats) were likely to have a significant response to finasteride 1 mg/day compared with patients treated with placebo and compared with patients with normal androgen sensitivity (≥24 CAG repeats) based on epigenetic weighted evaluation of the CAG alleles. Results of the present pilot study support the hypothesis that AR-CAG repeats, in conjunction with epigenetic factors, may help determine which women with hair loss will respond to finasteride therapy.
Finally, more recent studies demonstrated superiority of 0.5 mg oral dutasteride versus 1 mg oral finasteride in the treatment of male androgenetic alopecia.
Dutasteride is a dual inhibitor of 5-alpha reductase isotypes 1 and 2 (used for treatment of benign prostatic hyperplasia) and therefore capable of decreasing DHT levels to a greater extent than finasteride. A study performed by Gubelin Harcha et al. demonstrated efficacy and safety of 0.5 mg dutasteride in increasing hair growth and restoration in men with androgenetic alopecia while being well tolerated.
In another study performed by Jung et al., 0.5 mg dutasteride/day also proved to be effective in men with androgenetic alopecia recalcitrant to finasteride.
The plasma half-life time of dutasteride (3–5 weeks) is significantly longer than that of finasteride (age-dependent, from 5 to 8 h).
With respect to possible adverse effects related to therapeutic 5-alpha reductase inhibition, it may therefore be advisable to start patients on oral finasteride, and only if results are unsatisfactory at 6 months and tolerance is good, to switch from finasteride to dutasteride.
Olszewska and Rudnicka originally reported efficacy of 0.5 mg oral dutasteride for treatment of female androgenetic alopecia in a single case report: A 46-year-old female with androgenic alopecia, nonresponsive to minoxidil, who initially benefited from oral finasteride was switched to dutasteride due to only limited improvement under finasteride and persisting profound psychological distress resulting from the alopecia. Clinical evaluation and trichogram were applied for assessment of treatment. Additionally, mean hair diameter was monitored by means of computer-assisted dermoscopy. After 6 months of treatment, the authors noted significant improvement, and after 9 months the pattern hair loss was no longer recognizable. So far, efficacy of oral dutasteride for treatment of female androgenetic alopecia has not been confirmed in larger, and randomized, placebo-controlled trials.
Challenges in androgenetic alopecia are diagnosis and treatment of premature alopecia, early female androgenetic alopecia, androgenetic alopecia with comorbidities, and androgenetic alopecia with follicular microinflammation and fibrosis.
The first case series of premature alopecia in children in the age range from 6 to 10 years was reported by Tosti et al. Clinical examination showed hair loss with thinning and widening of the central parting of the scalp, both in boys and girls. There was a strong family history of androgenetic alopecia in all patients. All had normal physical development. In children and adolescents with early-onset alopecia, it is first important to differentiate if the hair loss is congenital or acquired. The differential diagnosis includes hypotrichosis simplex (without associated abnormalities) and ectodermal dysplasia (with associated delayed physical/psychological development, sweating, dental or nail abnormalities).
Premature androgenetic alopecia is an acquired hair loss with a characteristic pattern distribution and no associated abnormalities.
Therefore, it is important to assess the child’s general health status, physical and mental development, and particularly early onset of puberty. Premature androgenetic alopecia without signs of premature puberty is usually not associated with endocrinological abnormalities; nevertheless, referral to a pediatric endocrinologist is recommended.
Efficacy and safety data for topical minoxidil solution are not available before the age of 18 years; therefore, treatment occurs at the discretion of the prescribing physician (off-label use).
Systemic side effects of topical minoxidil (hypertrichosis and cardiovascular) are observed more frequently in children, especially at higher than the recommended dosages.
Nevertheless, treatment with topical 2 % minoxidil solution (before the age of 12 once daily, thereafter twice daily) is usually very effective.
Recognition of early female androgenetic alopecia may be challenging, when the patient complains of increased hair loss, but a widened hair part is yet not identifiable. At this stage, hair density is relative to the patient’s prior assessment of her hair, and only longitudinal observation would reveal the evolution over time.
Diversity of hair shaft diameter or anisotrichosis is an early diagnostic feature of androgenetic alopecia and is best appreciated in a central hair part with the dermatoscope.
Originally, Tosti et al. suggested that diversity of hair shaft diameter >20 % be diagnostic of androgenetic alopecia. Eventually, Rakowska et al. proposed more sophisticated criteria for diagnosis of female androgenetic alopecia based on dermoscopic findings:
Major criteria were (1) ratio of more than four empty follicles in four images (at 70-fold magnification) in the frontal area, (2) lower average thickness in the frontal area compared to the occiput, and (3) more than 10 % of thin hairs (<0.03 mm in diameter) in the frontal area.
Minor criteria were (1) increased frontal to occipital ratio of single-hair pilosebaceous units, (2) vellus hairs, and (3) peripilar signs.
Fulfillment of two major criteria or of one major and two minor criteria allows diagnosis of female androgenetic alopecia with a 98 % specificity.
Galliker and Trüeb performed a study to evaluate the value of trichoscopy as compared to the trichogram for the diagnosis of female androgenetic alopecia and found that trichoscopy is a valuable and superior method to the trichogram for diagnosis of female androgenetic alopecia, especially in early cases, with the highest yield irrespective of the formerly proposed cutoff of 20 % diversity of hair shaft diameter.
The coexistence of androgenetic alopecia with other morbid conditions may complicate the diagnosis, treatment, and course of androgenetic alopecia.
It must be borne in mind that hair loss often does not result from a single cause effect, but from a combination of factors that all need to be addressed simultaneously for success.
Particularly in women, the coexistence with iron deficiency, thyroid dysfunction, endocrine disorders, and hormonal treatments (oral contraceptives, replacement treatments) has an impact.
In a landmark study on hormonal diagnostics in female androgenetic alopecia, Moltz investigated 125 women aged 18–68 years with clinically typical androgenetic alopecia. Atypical uterine bleeding, seborrhea, acne, and hirsutism were frequently observed. Pathologic changes of one biochemical parameter was detected in 22.4 %, while 67.2 % revealed deviations of two or more parameters. The incidence rates of pathologic parameters were as follows: ferritin = 42 %, prolactin = 34 %, estradiol (E2) = 34 %, free testosterone (fT) = 29 %, dihydrotestosterone (DHT) = 28 %, sex hormone-binding globulin (SHBG) = 26 %, thyroid-stimulating hormone (TSH) = 20.8 %, DHEA-sulfate (DHEAS) = 19 %, testosterone = 14 %, 17α-hydroxyprogesterone = 11 %, folate = 7 %, Δ4-androstendione = 6 %, cortisol = 6 %, and vitamin B12 = 5 %.
Group and individual case analyses revealed significant correlations between the levels of the various androgens, prolactin, and TSH and the E2, sex hormone-binding globulin, and fT values; these in turn correlated with the occurrence of uterine bleeding anomalies (amount, duration, and interval) and resultant ferritin deficiency.
In a later study aiming at biochemical and trichological characterization of diffuse alopecia in women, Rushton et al. investigated one hundred women who presented with diffuse alopecia and compared them with 20 controls. In 40.9 % hormonal values were within control ranges. A raised DHT was found in 29.5 % and was the most frequently elevated androgenic finding. Thirty-four percent had changes in iron metabolism, while in 72.0 % serum ferritin levels were below the lowest control value. Taking high doses of multivitamins can influence iron absorption. According to Rushton a raised serum folic acid (>40 nmol/L) may indicate an excessive supplement intake. Rushton suggested that raised folic acid and vitamin B12 levels may induce excessive telogen hair shedding. On the other hand, vitamin B12 levels are adversely affected by cyproterone acetate/estradiol (CPA-E2) therapy; therefore, patients treated with CPA-E2 may require concurrent vitamin B12 supplementation in a suggested daily oral dosage of 100–200 μg to maintain their serum concentration levels above ideally 300 ng/L. With quantitative hair data available, no correlation between hormonal levels and any hair value was established.
The authors concluded that the endocrine dependence of diffuse alopecia appears to involve tissue dynamics such as receptor populations and binding protein phenomena, rather than specific levels of circulating hormones.
Diffuse alopecia was not significantly associated with any major endocrine disturbance; therefore, extensive laboratory investigations would seem unnecessary in the majority of women. However, a detailed hormonal profiling may be of value in women with marked bitemporal recession or when acne, hirsutism, or uterine bleeding anomalies coexist.
Finally, Schmidt et al. pointed out that androgen-dependent disorders of hair growth are due to more varied hormonal disturbances than elevated androgen serum levels alone. In view of the complex hormonal interactions between androgens, thyroid hormones, and prolactin, the thyrotropin-releasing hormone (TRH) stimulation test was performed in 38 female patients with androgen-induced alopecia, and the results were compared with those recorded in female control persons. The test is based on feedback mechanisms between hypothalamic TRH and hypophyseal TSH and prolactin and peripheral thyroid hormones. Baseline and stimulated TSH levels were significantly elevated. Therefore, hypothyroidism was recognized to be a significant contributing factor. In the case of prolactin, Schmidt et al. found stimulated levels also to be significantly elevated in the women with androgen-dependent alopecia. Orfanos and Hertel previously pointed out that functional hyperprolactinemia may yet be another cause of hair loss in women.
Hyperprolactinemia is the most frequent abnormality of the anterior pituitary gland. Clinical signs include inappropriate lactation, lack of menses, decreased libido, and infertility. General guidelines for diagnosing prolactin excess (hyperprolactinemia) define the upper threshold of normal prolactin at 25 μg/L for women. However, different methods for measuring prolactin are employed by different laboratories, and as such the serum reference range for prolactin is often determined by the laboratory performing the measurement. Furthermore, prolactin levels vary with age, sex, menstrual cycle stage, and pregnancy. The circumstances surrounding a given prolactin measurement (method and patient condition) must therefore be considered before the measurement can be accurately interpreted.
Diagnostic tests are useful when the probability of a disease being present is neither high nor low, since high degree of clinical certainty overrides the uncertainty of the laboratory data. The greater the number of different tests done, the greater the risk of getting false-positive or irrelevant leads. The possibilities for laboratory errors increase in the automated multiple-screen procedures. Therefore, remaining open-minded for the possibility of a multitude of cause-relationships underlying alopecia on the one hand, laboratory testing must be kept sharply focused on the other hand. Clinical suspicion is the determinant, and knowledge of clinical dermatology is the prerequisite for combining medical sense with economic sense in requesting the appropriate tests.
The most consistent of endocrinological abnormality associated with female androgenetic alopecia is polycystic ovary syndrome (PCOS). Originally described by Irving Stein and Michael Leventhal in 1935 in a case series of seven women with anovulation and polycystic ovaries discovered during surgery, the syndrome has most recently been defined by the Rotterdam criteria (Table 4.1). Given the broad definition described by the Rotterdam criteria, the prevalence of PCOS has been estimated to range from 15 to 18 %.
Table 4.1
2003 Rotterdam criteria for the diagnosis of polycystic ovary syndrome (PCOS)
1. Oligo- or anovulation |
<9 menses a year or missed menses for ≥3 months |
2. Clinical and/or biochemical signs of hyperandrogenism |
Hirsutism, acne, alopecia |
Serum levels of androgens, including androstenedione and testosterone, may be elevated. The free testosterone level is thought to be the best measure, with ca. 60 % of patients demonstrating elevated levels. Some other blood tests are suggestive but not diagnostic. The ratio of LH (luteinizing hormone) to FSH (follicle-stimulating hormone), when measured in international units, is elevated. Common cutoffs to designate abnormally high LH/FSH ratios are 2:1 or 3:1 as tested on day 3 of the menstrual cycle |
3. Echogenic evidence of polycystic ovaries and exclusion of other causes of hyperandrogenism and anovulation |
Polycystic ovaries on ultrasound are defined by the presence of >12 follicles in each ovary (each follicle measuring 2–9 mm in diameter) and/or increased ovarian volume of >10 mL |
Differential diagnosis of PCOS (laboratory evaluation): pregnancy (elevated serum or urine HCG), premature ovarian failure (elevated FSH and LH, low-normal estradiol levels), hypothyroidism (elevated TSH, low thyroxine levels), hyperprolactinemia (elevated prolactin level), late-onset congenital adrenal hyperplasia (elevated day 5 morning level of 17 hydroxyprogesterone), virilizing ovarian/adrenal tumor (total testosterone >200 ng/dL, DHEAS >700 μg/L, and elevated androstenedione), Cushing’s syndrome (elevated 24-h urinary free cortisol level, unsuppressed morning serum cortisol during the low-dose dexamethasone suppression test, and elevated midnight salivary cortisol) |
Requires 2 out of 3 for diagnosis of PCOS |
PCOS represents a complex disorder with several aberrant hormonal and metabolic pathways. Hyperandrogenism is one of the most important clinical features of the syndrome and the most relevant to the role of the dermatologist in diagnosis and management of the condition. Up to 60 % of women with PCOS have hirsutism, frequently seen on the upper lip, chin, areola, chest, back, and lower abdomen. Acne is another common cutaneous manifestation of hyperandrogenism in PCOS. Acne that originates or persists into adulthood and that is refractory to conventional treatment should raise suspicion to underlying PCOS. Compared with pubertal acne, women with PCOS tend to have more inflammatory lesions, especially on the lower face, neck, chest, and upper aspect of the back. Since 19–37 % of women with moderate to severe acne meet the diagnostic criteria for PCOS, these patients need to be investigated for PCOS.
Among women with PCOS, alopecia is a less frequent manifestation of hyperandrogenism than hirsutism and acne, though it responds less well to topical minoxidil than in female androgenetic alopecia that is not associated with PCOS, and therefore should be treated with combined antiandrogen therapy.
Most importantly, PCOS presents with potentially serious systemic long-term implications, including risks of metabolic syndrome and of endometrial carcinoma. PCOS is associated with several metabolic complications, including obesity, metabolic syndrome, and insulin resistance. Obesity is present in 75 % of women with PCOS, and up to 47 % of women with PCOS have metabolic syndrome. The diagnostic criteria for metabolic syndrome are summarized in Table 4.2.
Table 4.2
Diagnostic criteria for metabolic syndrome (Adult Treatment Panel III)
Requires >3 of the following criteria |
Waist circumference >88 cm |
Triglyceride level ≥150 mg/dL |
High-density lipoprotein (HDL) cholesterol <50 mg/dL |
Blood pressure ≥130/85 |
Fasting glucose level ≥100 mg/dL |
Insulin resistance is common among patients with PCOS independent of obesity. The cutaneous signs of hyperinsulinemia include acanthosis nigricans and acrochordons. Insulin plays an important role in the development of anovulation and hyperandrogenism, which explains the ability of insulin-sensitizing agents, such as metformin, to lower androgen levels and induce ovulation.
Women with PCOS have an increased risk of cardiovascular disease; however, it is not clear whether PCOS is an independent risk factor or whether cardiovascular disease results from the comorbidities associated with PCOS. In general, patients should be closely monitored and managed for obesity, diabetes, hyperlipidemia, and hypertension.
Treatment of PCOS includes weight loss, exercise, glucose control, combined oral contraceptives and antiandrogen agents (cyproterone acetate, drospirenone), insulin-sensitizing drugs (metformin), and topical therapies for hair removal (laser hair removal, topical eflornithine), acne (standard dermatologic therapies), and hair growth (topical minoxidil), as needed.
Although combined oral contraceptive pills are widely considered first-line therapy in PCOS, a drawback may be metabolic side effects and risk of thromboembolism. The current recommendation suggests low-dose ethinyl estradiol (<50 μg), avoiding androgenic progestins, and addition of metformin to offset insulin resistance.
There has been significant recent media discussion regarding venous thromboembolic events in conjunction with drospirenone use.
Relative to nonusers, drospirenone increases venous thromboembolus risk 6.3-fold, levonorgestrel 3.6-fold, gestodene 5.6-fold, cyproterone acetate 6.8-fold, and desogestrel 7.3-fold. The absolute venous thromboembolus risk of drospirenone-containing oral contraceptive pills is 23–137 events/100,000 women-years, and comparatively risk in pregnancy 199.7 events/100,000 women-years.
The observation that many women show increased shedding of hair from 2 weeks to 3–4 months after they stop taking an oral contraceptive probably simulates that which is commonly seen after parturition.
During the second half of pregnancy, the percentage of anagen hairs increases from the normal 85–95 %, at this time also the percentage of hairs of large shaft diameter is higher than in nonpregnant women of the same age. After partuition the follicles, in which anagen has been prolonged, rapidly enter catagen and then telogen, with an increased shedding of hair evident after 3–4 months (postpartum effluvium). Most women will return to their usual hair growth cycle between 6 and 12 months after birth. Postpartum hair loss usually returns the hair to pre-pregnancy thickness, unless it leads over to female androgenetic alopecia. In case of persistent postpartum effluvium (>12 months), excessive hair loss may be caused by common conditions, such as female androgenetic alopecia, iron deficiency, or hypothyroidism. Lesser common conditions include persistent hyperprolactinemia (Chiari–Frommel syndrome) and postpartum hypopituitarism (Sheehan syndrome) caused by pituitary necrosis due to blood loss and hypovolemic shock during childbirth.
More frequently, contraceptive pills or hormone replacement therapies with progestogens that possess net androgenic activity, such as norethisterone, levonorgestrel, and tibolone, induce hair loss in genetically predisposed women.
It has been proposed that in the presence of a genetic susceptibility, it is the estrogen to androgen ratio that might be responsible for triggering hair loss in women. In the same line is the observation of hair loss induced in the susceptible women by treatment with tamoxifen or with the aromatase inhibitors letrozole, anastrozole, and formestane for breast cancer.
Besides the aforementioned comorbid conditions, lifestyle factors, such as malnutrition, obesity, smoking, exposure to ultraviolet radiation (UVR), and stress, may play an additional role.
The quantity and quality of hair are closely related the nutritional state of an individual. Normal supply, uptake, and transport of proteins, calories, trace elements, and vitamins are of fundamental importance in tissues with a high biosynthetic activity such as the hair follicle. Because hair shaft is composed almost entirely of protein, protein component of diet is critical for production of normal healthy hair. The rate of mitosis is sensitive to the calorific value of diet, provided mainly by carbohydrates stored as glycogen in the outer hair root sheath of the follicle. Finally, a sufficient supply of vitamins and trace metals is essential for the biosynthetic and energetic metabolism of the follicle.
Debate exists regarding protein intake requirements. The amount of protein required in an individual’s diet is determined by overall energy intake, the body’s need for nitrogen and essential amino acids, body weight and composition, rate of growth of the individual, physical activity level, individual’s energy and carbohydrate intake, and presence of illness or injury. Physical activity and exertion as well as larger muscular mass increase the need for protein. Requirements are also higher during childhood for growth and development, during pregnancy and lactation, or when the body needs to recover from malnutrition, trauma, or after an operation. In case of lack of energy through nutrition, the body utilizes protein from the muscle mass to meet energy needs, eventually leading to muscle wasting. If the individual’s intake of protein is inadequate, then the muscle will also waste as more vital cellular processes, such as respiration enzymes and blood cells, recycle muscle protein for their proper requirements. Recommended dietary allowance in grams of protein needed per day are listed in Table 4.3.
Table 4.3
Recommended dietary allowance in grams of protein per day
Children ages 1–3 years | 13 g of protein per day |
Children ages 4–8 years | 19 g of protein per day |
Children ages 9–13 years | 34 g of protein per day |
Girls ages 14–18 years | 46 g of protein per day |
Boys ages 14–18 years | 52 g of protein per day |
Women ages 19–70+ years | 46 g of protein per day |
If pregnant or breastfeeding | 71 g of protein per day |
Men ages 19–70+ years | 56 g of protein per day |
The generally accepted daily protein dietary allowance, measured as intake per kilogram of body weight, is 0.8 g/kg. However, this recommendation is based on structural requirements but disregards the use of protein for energy metabolism. This requirement is for a normal sedentary person, while several studies have come to the conclusion that active people and athletes may require more elevated protein intake due to increase in muscle mass and sweat loss, as well as need for body repair, and energy source. Suggested amounts vary between 1.6 and 1.8 g/kg, while a proposed maximum daily protein intake would be at least 10 %, but not more than 35 % of energy requirements, i.e., approximately 2–2.5 g/kg.
Micronutrients relevant to the hair and their recommended daily allowances are listed in Table 4.4.
Table 4.4
Micronutrients relevant to the hair and recommended daily allowances
Vitamins |
B vitamins |
Biotin (vitamin H): 300 μg |
Niacin (vitamin B3): 20 mg |
Folic acid: 300 μg |
Riboflavin (vitamin B2): 1.7 mg |
Pantothenic acid (vitamin B5): 5 mg |
Pyridoxine (vitamin B6): 1.5 mg |
Cyanocobalamin (vitamin B12): 3 μg |
Antioxidants |
Vitamin A/beta-carotene: 6 mg |
Alpha-tocopherol (vitamin E): 10 IU |
Trace metals |
Iron: depending on age and sex (in men: 8–10 mg) |
Zinc: 15 mg |
Selenium: 50 μg |
It appears that on a typical Western diet, the hair follicle should have no problem in producing an appropriate hair shaft.
Nevertheless, vitamin and nutritional deficiencies are not uncommonly observed in adolescent females and young women with eating disorders (anorexia and bulimia nervosa) and are especially common in the elderly population.
In general, malnutrition is due to one or more of following factors: inadequate food intake, food choices that lead to dietary deficiencies, and illness that causes increased nutrient requirements, increased nutrient loss, poor nutrient absorption, or a combination of these factors.
Obesity is a risk factor for multiple health problems. In a cross-sectional study conducted in Taiwan, the authors attempted to determine the association between body mass index (BMI) and alopecia severity in men with male pattern androgenetic alopecia. The medical charts and photographs of 189 men with a clinical diagnosis of male pattern androgenetic alopecia were reviewed. The men had a mean age of 30.8 years. In those with male pattern androgenetic alopecia (n = 142), men with severe alopecia (grades V–VII) had higher BMI than those with mild to moderate alopecia (grades I–IV) (25.1 vs. 22.8 kg/m2, p = 0.01). After multivariate adjustments, the risk for severe alopecia was higher in the overweight or obese (BMI ≥24 kg/m2) subjects with androgenetic alopecia (odds ratio 3.52, p < 0.01). In early-onset male pattern androgenetic alopecia (n = 46), the risk for having severe alopecia was also higher in the overweight or obese subjects (odds ratio 4.97, p = 0.03).
The authors concluded that higher BMI was significantly associated with greater severity of hair loss in men with male pattern androgenetic alopecia, especially in those with early-onset androgenetic alopecia.
Since previous investigations had revealed an association of severe early-onset androgenetic alopecia with ischemic heart disease, Hirsso et al. examined the possible association between early-onset alopecia and low-grade inflammation measured by high-sensitivity C-reactive protein (hs-CRP) that has been recommended for the assessment of the cardiovascular disease risk. The study population consisted of 727 young Finnish men, aged 25–34 years, participating in a national survey. The grade of alopecia was assessed by a trained nurse using the Hamilton–Norwood Classification Scale. Men with moderate to extensive alopecia (17 %) had a BMI and larger waist, upper arm, hip and waist circumference than those with little to no alopecia (p < 0.05), and statistically insignificant differences were seen in the waist-to-hip circumference ratio (WHR), diastolic blood pressure, and hs-CRP. With increasing hs-CRP, the mean WHR increased, but only among men with moderate to extensive alopecia (p = 0.043).
The authors concluded that there is a relation between moderate to extensive alopecia and low-grade inflammation, a predictor of a future cardiovascular disease, especially combined with central obesity, among men younger than 35 years.
Ultimately, a study was performed to analyze the presence of cardiovascular risk factors included in the Adult Treatment Panel III criteria for metabolic syndrome, the prevalence of carotid atheromatosis, hormonal (aldosterone, insulin, testosterone, and sex hormone-binding globulin) factors, and acute phase reactant (C-reactive protein, fibrinogen, D-dimers, erythrocyte sedimentation rate) variables in male and female patients with androgenetic alopecia and in a control group and to analyze differences among the groups. The case–control study included 154 participants, 77 with early-onset androgenetic alopecia (40 males and 37 females) and 77 healthy control subjects (40 males and 37 females) from the Department of Dermatology at a University Hospital in Granada, Spain. Metabolic syndrome was diagnosed in 60 % of male patients with androgenetic alopecia (odds ratio [OR] = 10.5, 95 % confidence interval [CI] 3.3–32.5), 48.6 % of female patients with androgenetic alopecia (OR = 10.73, 95 % CI 2.7–41.2), 12.5 % of male control subjects, and 8.1 % of female control subjects (p < 0.0001). Atheromatous plaques were observed in 32.5 % of male patients with androgenetic alopecia (OR = 5.93, 95 % CI 1.5–22.9) versus 7.5 % of male control subjects (p = 0.005) and 27 % of female patients with androgenetic alopecia (OR = 4.19, 95 % CI 1.05–16.7) versus 8.1 % of female control subjects (p = 0.032). Aldosterone and insulin levels were significantly higher in the male and female patients with androgenetic alopecia versus their respective control subjects. Mean values of fibrinogen were significantly higher in male patients with androgenetic alopecia, whereas values of fibrogen, C-reactive protein, and D-dimers were significantly higher in female patients with androgenetic alopecia versus their respective control subjects. The authors concluded that the determination of metabolic syndrome and ultrasound study of carotid arteries may be useful screening methods to detect risk of cardiovascular disease in male and female patients with early-onset androgenetic alopecia and signal a potential opportunity for early preventive treatment.
Finally, Starka et al. suggested that premature androgenetic alopecia and insulin resistance are a clinical constellation that may represent the male homologue or phenotype of polycystic ovary syndrome. Because of its association with metabolic syndrome and altered glucose metabolism, both men and women with early androgenetic hair loss should be screened for impaired glucose tolerance and diabetes mellitus II.
Besides being the single most preventable cause of significant cardiovascular and pulmonary morbidity and an important cause of death in the general population, tobacco smoking has been associated with various adverse effects on the skin and hair. Premature skin aging has attracted the attention of the medical community only since the 1960s, although a relation between smoking and skin complexion was first suggested as early as 1856. In 1996, Mosley and Gibbs originally reported a significant relationship between smoking, premature gray hair, and baldness in patients visiting a general surgical outpatient clinic in the United Kingdom. Subsequently, Trüeb’s observation of strikingly dissimilar androgenetic alopecia in a 52-year-old monozygotic male twin pair led to further speculations on the possibility of an association between smoking and hair loss, since studies on the degree of alopecia among monozygotic twins aged over 50 have shown that intrapair differences are negligible in 92 %, slight in 8 %, and striking in none. A salient feature differing the twin brothers in their personal histories was that the balding brother admitted to long-standing, heavy cigarette smoking, while the other was a nonsmoker. Eventually, a population-based cross-sectional survey among Asian men 40 years or older showed statistically significant positive associations between moderate or severe androgenetic alopecia and smoking status, current cigarette smoking of 20 cigarettes or more per day, and smoking intensity. The odds ratio of early-onset history for androgenetic alopecia grades increased in a dose–response pattern. Risk for moderate or severe androgenetic increased for family history of first-degree and second-degree relatives, as well as for paternal relatives.
The mechanisms by which smoking accelerates hair loss have not been examined, but are believed to be multifactorial and related to effects of cigarette smoke on the microvasculature, cutaneous collagen, and elastic tissue, to pro-oxidant effects of smoking, and to increased hydroxylation of estradiol, as well as inhibition of the enzyme aromatase, which converts androgens to estrogens, creating a relative hypoestrogenic state. Evidence of the consequence of impaired circulation and wound healing is a higher complication rate of hair restoration surgery in smokers versus nonsmokers. Besides inducing local ischemia, the decreased capillary blood flow in the dermal papilla of the hair follicles may focally shunt more toxic substances. Cigarette smoke contains over 4800 chemicals, many of which are known to be toxic to cells and 69 are known to cause cancer. Both nicotine and cotinine are detected in hair samples of smokers. Smoke genotoxicants metabolized in hair follicle cells may cause DNA damage through the production of DNA adducts, and smoking-associated mitochondrial DNA mutations have been shown in human hair follicles, though the relevance of these for hair follicle pathology is as yet unknown. Since substantial extracellular matrix remodeling is involved in the hair follicle growth cycle, especially during catagen-associated hair follicle regression, it is conceivable that cigarette smoke-induced imbalance in the intra- and perifollicular protease/antiprotease systems controlling tissue remodeling may also affect the hair follicle growth cycle. Finally, smoking-induced oxidative stress and a disequilibrium of antioxidant systems may lead to the release of proinflammatory cytokines from follicular keratinocytes, which by themselves have been shown to inhibit the growth of isolated hair follicles in culture.
The fact that cigarette smoke-associated hair loss is of the androgenetic type indicates that genetic factors contribute. On the other hand, recent studies on the evolution of androgenetic hair loss have focused on oxidative stress: Naito et al. analyzed the effect of the lipid peroxides on hair follicles and observed that the topical application of linolein hydroperoxides, one of the lipid peroxides, leads to the early onset of the catagen phase in murine hair cycles. Furthermore, they found that lipid peroxides induced apoptosis of hair follicle cells. They also induced apoptosis in human epidermal keratinocytes by upregulating apoptosis-related genes. These results indicate that lipid peroxides, which can cause free radicals, induce the apoptosis of hair follicle cells, and this is followed by early onset of the catagen phase.
Ultimately, Bahta et al. cultured dermal hair papilla cells (DPCs) from balding and non-balding scalp and demonstrated that balding DPCs grow slower in vitro than non-balding DPCs. Loss of proliferative capacity of balding DPCs was associated with changes in cell morphology, expression of senescence-associated beta-galactosidase, decreased expression of proliferating cell nuclear antigen and Bmi-1, upregulation of p16(INK4a)/pRb, and nuclear expression of markers of oxidative stress and DNA damage including heat shock protein-27, superoxide dismutase catalase, ataxia-telangiectasia-mutated kinase (ATM), and ATM- and Rad3-related protein.
The finding of premature senescence of balding DPC in vitro in association with expression of markers of oxidative stress and DNA damage suggests that balding DPCs are particularly sensitive to environmental stress.
While the consequences of yet another environmental stress, namely, sustained UVR on unprotected skin, are well appreciated, mainly photocarcinogenesis and solar elastosis, the effects of UVR on the evolution of androgenetic alopecia have only recently found attention.
However, some clinical and morphological observations suggest that UVR has some negative effect on hair growth.
Camacho et al. originally reported a peculiar type of telogen effluvium following sunburn of the scalp after 3–4 months with hairstyles that left areas of scalp uncovered during prolonged sun exposure. The clinical features were increased frontovertical hair shedding along with a trichogram that disclosed an increase of telogen hairs and dystrophic hairs. In women the hairs on the frontal region appeared unruly, and the frontovertical alopecia showed loss of the frontal hair implantation line.
The pathomechanism of this type of telogen effluvium is not clear. It has been proposed that the columns of the cells in the hair shaft act as an efficient fiber-optic-type system, transmitting UV light downward into the hair follicle. Morphologically, the keratinocytes within the hair shaft are arranged in compressed linear columns that resemble the coaxial bundles of commercial fiber-optic strands. Thus, hair follicular melanocytes located in the region of the hair matrix may function as UV biosensors and respond to photic inputs. Depending on the quantity of UVR exposure, it is conceivable that also photodamage may occur at this site, resulting in telogen effluvium.
Histopathologically, elastosis is regularly found in scalp biopsies, especially in alopecic conditions, but so far has largely been ignored. Up to date, no controlled study has been performed on the degree of scalp elastosis in relation to the pace of development, duration, or grade of androgenetic alopecia, though it would seem to be a good marker for exposure to UVR penetrating the skin. Interestingly, UVB irradiation has been found to stimulate the synthesis of elastic fibers by modified epithelial cells surrounding the hair follicle and sebaceous glands in mice.
As a consequence of increased leisure time with a growing popularity of outdoor activities and holidays in the sun, awareness of sun protection has become important. Topically applied chemicals that act as sun protectors are widely utilized and offer the most convenient means of protecting the glabrous skin against acute (sunburn) and chronic pathologic effects of UVR. Out of cosmetic reasons, their use on the hair-bearing scalp is problematic, unless complete baldness is present. Although hats provide the best protection of the scalp from UVR, not all patients find it convenient or acceptable for this purpose.
Ultimately, alternatives have been the focus of more recent investigation to overcome the problems associated with the topical use of sunscreens: studies illustrate photoprotective properties of topical melatonin and of supplemented antioxidants, particularly beta-carotene (pro-vitamin A), α-tocopherol (vitamin E), and L-ascorbate (vitamin C). Topical melatonin has been found to suppress UV-induced erythema and UV-induced reactive oxygen species in a dose-dependent manner. Preclinical studies illustrate photoprotective properties of supplemented antioxidants; however, clinical evidence that these prevent, retard, or slow down solar skin damage is still impending.
Nevertheless, these results suggest the probable utility of combining topical melatonin, supplemented antioxidants, and sunscreens to maximize photoprotection.
Finally, the limited success rate of treatment of androgenetic alopecia with minoxidil and finasteride—even in the absence of comorbid conditions—means that further pathogenic pathways may be taken into account. On histological examination of scalp biopsies, the miniaturization of terminal hairs is frequently associated with perifollicular lymphocytic infiltration and eventually fibrosis. Therefore, it is conceivable that the role of this microscopic follicular inflammation causing fibrosis below the shortened balding follicle has been underestimated, though it seems likely that this would prevent the follicle to reform a terminal hair follicle.
The implication of microscopic follicular inflammation in the pathogenesis of androgenetic alopecia has emerged from several independent studies.
An early study referred to an inflammatory infiltrate of activated T-cells and macrophages in the upper third of the hair follicles, associated with an enlargement of the follicular dermal sheath composed of collagen bundles (perifollicular fibrosis), in regions of actively progressing alopecia. Horizontal section studies of scalp biopsies indicated that the perifollicular fibrosis is generally mild, consisting of loose, concentric layers of collagen that must be distinguished from cicatricial alopecia.
Mahé et al. proposed the term follicular microinflammation, because the process involves a slow, subtle, and indolent course, in contrast to the inflammatory and destructive process in the classical inflammatory scarring alopecias. However, the significance of follicular microinflammation and fibrosis in androgenetic alopecia has remained controversial.
Nevertheless, morphometric studies in male patients with androgenetic alopecia treated with topical minoxidil showed that 55 % of those with microinflammation had regrowth in response to treatment, in comparison to 77 % in those patients without inflammation and fibrosis.
In general, androgenetic alopecia is understood to represent a noninflammatory and non-scarring process that eventually leads to permanent hair loss of the affected scalp. In Zinkernagel and Trüeb’s original description of fibrosing alopecia in a pattern distribution, patients displayed progressive scarring alopecia in a pattern distribution. Close clinical examination reveals obliteration of follicular orifices, perifollicular erythema, and follicular keratosis limited to the area of androgenetic hair loss (Fig. 4.5a–c). Histological findings of androgenetic alopecia, i.e., increased numbers of miniaturized hair follicles with underlying fibrous streamers, are evident in the majority of patients and associated with a perifollicular lymphocytic infiltrate. A pattern of follicular interface dermatitis targeting the upper follicle is found in early lesions, whereas perifollicular lamellar fibrosis and the presence of selectively fibrosed follicular tracts characterize late lesions.


Fig. 4.5
(a–c) Fibrosing alopecia in a pattern distribution: (a) Patterned scarring alopecia. (b) Follicular erythema. (c) Follicular keratosis
The pattern distribution and histological findings share features with Kossard’s original description of postmenopausal frontal fibrosing alopecia. Frontal fibrosing alopecia represents a peculiar condition originally described in women in the postmenopause. The condition presents with a symmetric, marginal alopecia along the frontal and frontotemporal hairline, often with concomitant thinning or complete loss of the eyebrows (Fig. 4.6a). Affected women typically present with the complaint of asymptomatic, progressive recession of their frontal hairline. The affected scalp skin is pale and smooth with loss of follicular orifices; often perifollicular erythema and follicular keratinization are observed marking the underlying inflammatory process. Histopathologic examination of affected scalp shows again a reduced number of hair follicles, which have been replaced by fibrous tracts, and a perifollicular, lymphocytic, lichenoid infiltrate that is typical of lichen planopilaris. Kossard et al. later interpreted this type of alopecia as a frontal variant of lichen planopilaris on the basis of histopathologic and immunohistochemical studies.


Fig. 4.6
(a–g) Frontal fibrosing alopecia: (a) Female frontal fibrosing alopecia. (b) Male frontal fibrosing alopecia, with (c) loss of sideburns. (d, e) Loss of peripheral body hair in an anterolateral leg alopecia-like pattern and on the lateral aspects of the thigh. (f) Facial papules. (g) Lichen planus-like nail changes
Since Kossard’s original description in 1994, the number of cases of frontal fibrosing alopecia has exploded exponentially worldwide, while its etiology remains obscure. The condition has meanwhile also been described in men with the typical presentation of scarring alopecia of the sideburns (Fig. 4.6b, c). Moreover, it has been recognized to represent a more generalized rather than localized process of inflammatory scarring alopecia, with extension beyond the frontotemporal hair line, and loss of peripheral body hair (Fig. 4.6d, e).
More recently, peculiar facial papules have been reported in association with frontal fibrosing alopecia representing facial vellus hair involvement and described by patients as “roughening” of facial skin (Fig. 4.6f).
Follicular microinflammation and fibrosis, frontal fibrosing alopecia, and fibrosing alopecia in a pattern distribution seem to represent a continuum from microscopic inflammation and fibrosis to localized, more generalized forms of clinically perceptible inflammatory scarring alopecia. Moreover, considerable overlap exists among frontal fibrosing alopecia, lichen planopilaris, and fibrosing alopecia in a pattern distribution: postmenopausal frontal fibrosing alopecia has been described in association with lichen planus elsewhere, including the oral cavity and the nails (Fig. 4.6g), and frontal fibrosing alopecia-type changes as well as facial papules (Fig. 4.7) can also be observed in patients with fibrosing alopecia in a pattern distribution.


Fig. 4.7
Facial papules in fibrosing alopecia in pattern distribution
Ultimately, Olsen acknowledged existence of clinically significant inflammatory phenomena and fibrosis in androgenetic alopecia and proposed the term cicatricial pattern hair loss for these conditions.
4.2.2 Aging Hair
Hair length, color, and cosmetic properties play an important role in people’s physical appearance and self-perception. Civilized mankind’s ancient preoccupation with hair is heightened as today’s increasing life expectancy fuels the desire to preserve youthfulness. This attention reflects a hair care market that is a multibillion dollar enterprise.
The study of hair aging focuses on two main streams of interest: on the one hand, the esthetic problem of aging hair and its management, in other words everything that happens outside the skin, and, on the other hand, the biological problem of aging hair, in terms of microscopic, biochemical (hormonal, enzymatic), and molecular changes, in other words the “secret life” of the hair follicle in the depth of the skin.
Most literature on age-related hair changes focuses on alopecia, but it is equally important that the hair fibers that emerge from the scalp exhibit significant age-related changes that have an equal impact on the overall cosmetic properties of the hair. It is these changes that are ultimately experience by subjects that have retained their hair as they age.
Aging of hair affects hair color, hair production, and structural properties of the hair fiber with its consequence for manageability and overall appearance of hair. Age-related deterioration of cosmetic properties of hair is related to changes in hair pigmentations (graying), diameter, curvatures, structural properties (stretching, bending, torsional rigidity), lipid composition, and the interdependence of these changes. Depending on the individual’s original hair color, quantity, quality, and hair care habits, there is a great variability in the age of onset of first signs of hair aging.
Hair graying is closely related to chronological age, and the age of its onset is largely controlled by genetics. The normal incidence of hair graying is 34 ± 9.6 years in Caucasians and 43.9 ± 10.3 years in Africans. As a rule of thumb, by 50 years of age, 50 % of people have 50 % gray hair. This graying incidence appears irrespective of sex and hair color. In men, graying usually begins at the temples and in the sideburns. Women will usually start around the perimeter of the hairline. Gradually, the gray works its way back through the top, sides, and back of the hair. Finally, the perception of graying is dependent on the original hair color, with the perception of “any gray” occurring earlier in dark hair and later in light hair. Vice versa, the perception of “completely gray” is perceived to occur earlier in light hair and later in dark hair.
Hair is said to gray prematurely if it occurs before the age of 20 in Caucasians, of 25 in Asians, and of 30 in Africans.
While premature canities most commonly appear without underlying pathology, presumably inherited in an autosomal dominant manner (familial premature graying), it has also been linked to a similar cluster of autoimmune disorders observed in association with vitiligo, i.e., pernicious anemia, and autoimmune thyroid disease, as well as with rare premature aging syndromes (progerias), such as Hutchinson–Gilford and Werner’s syndrome.
Reports—again—linking cigarette smoking with premature hair graying have drawn on the one hand the attention to the role of oxidative stress on hair growth and pigmentation and on the other hand to canities as a marker for the general health status.
A possible explanation of the observation may be that smoking-related diseases increase aging in general, including pigmentation. However, more direct effects, e.g., via smoke genotoxin-induced apoptosis, may also be involved. Wood et al. demonstrated for the first time that human white scalp hair shafts accumulate hydrogen peroxide (H2O2) in millimolar concentrations and almost absent catalase and methionine sulfoxide reductase (MSR) protein expression in association with functional loss of methionine sulfoxide repair in the entire gray hair follicle. Accordingly, methionine sulfoxide formation of methionine residues (Met), including Met 374 in the active site of tyrosinase, the key enzyme in melanogenesis, limits enzyme functionality, which eventually leads to loss of hair color. While the entire hair follicle is subject to H2O2-mediated stress, it is tempting to assume that, besides tyrosinase and MSR, other proteins and peptides, including anti-apoptotic Bcl-2 protein, are targets for oxidation, which in turn could explain melanocyte apoptosis in the gray hair follicle. Moreover, H2O2-mediated oxidation has been documented for many other important regulators of pigmentation, including the proopiomelanocortins a-melanocyte-stimulating hormone (MSH) and β-endorphin, the prohormone convertases, and the synthesis and recycling of the ubiquitous cofactor 6-tetrahydrobiopterin.
Whether canities, premature or otherwise, is a predictor or risk marker for disease remains controversial, mainly due to poor epidemiologic study design. Moreover, if it exists at all, it is more likely to reflect associated genetic effects rather than direct linkage.
Anecdotal evidence indicates that gray hair becomes coarser and less manageable than pigmented hair. Gray hair is said to often fail to hold a temporary or permanent set and to be more resistant to incorporating artificial color, both of which suggest changes to the underlying substructure of the hair fiber. Gray hair has been found to have increased sensitivity to weathering, increased cysteic acid residues and decreased cystine, and increased fiber reactivity to reducing and oxidizing agents. Moreover, gray hair is more sensitive to UVR. Photochemical impairment of the hair includes degradation and loss of hair proteins as well as degradation of hair pigment. UVB radiation is responsible for hair protein loss, and UVA radiation is responsible for hair color changes. Absorption of radiation in photosensitive amino acids of the hair and their photochemical degradation is producing free radicals. They have adverse impact on hair proteins, especially keratin, while melanin can partially immobilize free radicals and block their entrance in keratin matrix.
Given the close interaction of melanin-transferring melanocytes with hair shaft-forming pre-cortical keratinocytes, it is conceivable that other functions of these cell types are affected by this activity. One possibility is that melanin transfer decreases keratinocyte turnover and increases keratinocyte terminal differentiation. Indeed, white beard hair has been shown to grow up to four times the rate of adjacent pigmented hair. In this way, aging hair follicles may reprogram their matrix keratinocytes to increase production of medullary, rather than cortical, keratinocytes. In fact the medulla is often enlarged and collapsed, forming a central cavity in gray and white hairs.
An evolutionary basis for the increased medullation of senile white hair may reflect the enhanced insulation provided by these hairs which would confer an important benefit for temperature regulation. In this way, it may compensate for the loss of sunlight-absorbing and thus heat-trapping properties of melanized dark hair.
Hair diameter changes with age are likely to impart the largest impact on overall perception of hair aging. The largest study on female hair diameter in relation to age was performed in 1988 by Otsuka and Nemoto on >18,000 Japanese females aged 10 through 60 years. The study showed that hair diameter versus age does not represent a linear relationship, but rather a curvature that increases to a maximum near the age of 40 and thereafter decreases. The second largest study performed more recently by Robbins et al. on 1,099 Caucasian females aged 18 through 66 years with perceived hair loss revealed the age for maximum diameter to be 43–46 years. Several smaller studies on age versus diameter were in reasonable agreement with the conclusions of these two large studies, indicating that the age of maximum diameter for females is near the 40s. One exception is the study of Birch et al. on >300 Caucasian females concluding that the age for maximum hair diameter was near the 30s.
Postmenopausal women were shown to have significantly lower hair fiber diameters (lower frontal scalp hair density and lower growth rates) than premenopausal women for the frontal but not the occipital scalp region. This effect was independent of age, together with the co-localization with androgenetic alopecia, suggesting an impact of the hormonal effects of menopause on hair diameter.
In contrast to females, again, Otsuko and Nemoto found in Japanese men scalp hair fiber diameter to increase to a maximum in the late teenage years and then to decrease relatively rapidly with increasing age. Trotter and Dawson found similar effects in a study on 82 male French Canadians. Courtois et al. studied 10 French male subjects aged 25–49 over a period of 14 years and demonstrated that the diameter of hair shafts decreased with increasing age beginning at age 25.
Changes in hair fiber curvature with age has an important effect on almost all important cosmetic properties. Nagase et al. studied curvature of hair from 132 Japanese females aged 10 through 70 years and found an increase in curvature with age. In a different publication by the same authors, frizziness was explained as a lack of synchronization in the curvature of neighboring hair fibers in an assembly of hair. Respective data for Caucasian women and men are as yet not available.
Changes in hair fiber diameter and curvature with age also affect structural properties of hairs increasing combing forces and therefore breakage. Combing forces have been shown to increase with decreasing fiber curvature and increasing fiber curvature. Therefore, combing forces can be expected to increase with age, leading to increase breakage of hair.
Hair breakage is a multifactorial phenomenon involving bending, stretching, and torsion deformations and including:
Tangle formation, with hair looped over other hairs
Knots (trichonodosis)
Impact breakage or pulling a comb or brush through a tangle
Physical damage or wear by abrasion from specific grooming devices such as combs, picks, or brushes
Robbins et al. described the effects of diameter and hair density in relation to age and proposed a new metric relative scalp coverage for the perception of the amount of hair on one’s head. This metric is defined as a two-dimensional parameter as the average of fiber cross-sectional area multiplied by the number of hair fibers per square centimeter. Considering diameter and density, relative scalp coverage was found to peak at age 35, as a result of hair diameter increasing until about age 45, and density peaking in the late 20s. Robbins et al. further proposed that when additional relevant parameters are taken into account for relative scalp coverage, it will provide a multidimensional parameter involving diameter, density, fiber curvature, and color. Ultimately, as the color of hair changes with age, the perception of scalp coverage will change. Also, the graying effect with age will decrease luster.
Finally, hair lipid levels and hair lipid composition change with age: the two major sources of hair lipids are the hair matrix cells (cholesterol, cholesterol sulfate, ceramides, covalent fatty acids, 18-methyl eicosanoic acid) and the hair follicle-associated sebaceous glands (squalene, wax esters, triglycerides, total fatty acids). The amount of sebum produced varies with the size of sebaceous glands, being low before puberty, rapidly increasing at puberty, and remaining at a high level until 45–50 where it declines. The decline is greater in females than in males.
Age-related lipid changes affect hair greasiness, shine, softness, and smoothness.
At last, basic scientists interested in the biology of hair growth and pigmentation have exposed the hair follicle as a highly accessible and unique model that offers important opportunities also to the gerontologist for the study of aging. Its complex multicell-type interaction system involving epithelium, mesenchyme, and neuroectoderm and its unique cyclical activity of growth, regression, rest, and regrowth provides the investigator with a range of stem, differentiating, mitotic, and postmitotic terminally differentiated cells, including cells with variable susceptibility to apoptosis, for study.
Aging is a complex process involving various genetic, hormonal, and environmental mechanisms. A number of intrinsic and extrinsic modulating factors for hair growth and pigmentation have been identified and are being further tested in vitro.
Much like the skin, the hair is subject to intrinsic or chronologic aging and extrinsic aging due to environmental factors. Both occur in conjunction with the other and are superimposed on each other.
Intrinsic factors are related to individual genetic and epigenetic mechanisms with interindividual variation. Examples are familial premature graying and androgenetic alopecia. Extrinsic factors include ultraviolet radiation (UVR), smoking, and nutrition.
Senile involutional or senescent alopecia has been defined as non-androgen-dependent hair thinning found in those over 60 years of age. Much like androgenetic alopecia, it involves a progressive decrease in the number of anagen follicles and hair diameter. It frequently occurs together with androgenetic alopecia, further complicating its delineation from the latter.
In their study on aging and hair cycles over an exceptionally long duration of 8–14 years, Courtois et al. found a reduction in the duration of hair growth and in the diameter of hair shafts and a prolongation of the interval separating the loss of a hair in telogen and the emergence of a replacement hair in anagen (latency phase).
These phenomena resemble those observed in the course of androgenetic alopecia, although their development is less marked, suggesting androgenetic alopecia to represent a premature aging phenomenon.
This aging process was evidenced by a reduction in the number of hairs per unit area and deterioration in the quality of scalp hair. The reduction in density was manifested to different degrees in different individuals. It amounted to less than 10 % in 10 years in the individuals with the least alopecia and was much more pronounced in the balding subjects. The maximal length of hair diminished as the subjects aged; in parallel, the hairs became finer. However, among non-balding subjects, there was a tendency for the proportion of thicker hairs to increase. Finally, aging did not appear to follow a perfectly regular course over time. Periods of stability, or even partial remission, alternated with periods of more marked evolution, reflecting perhaps the influence of individual factors such as the subject’s general health, lifestyle, and risk factors for accelerated aging.
Recent data comparing androgenetic alopecia and senescent alopecia using microarray analysis have demonstrated significant differences in the gene expression patterns suggesting they represent different entities.
In androgenetic alopecia, genes required for anagen onset (Wnt-beta-catenin, TGF-alpha, TGF-beta, Stat-3, Stat-1), epithelial signal to dermal papilla (PPARd, IGF-1), hair shaft differentiation (Notch, Msx2, KRTs, KAPs), and anagen maintenance (Msx2, Activin, IGF-1) were downregulated, and genes for catagen (BDNF, BMP2, BMP7, VDR, IL-1, ER) and telogen induction and maintenance (VDR, RAR) were upregulated. In senescent alopecia, genes involved in epithelial signal to dermal papilla (FGF5), actin cytoskeleton (DST, ACTN2, TNNI3, PARVB), and mitochondrial function (JAK2, PRKD3, AK2, TRAP1, TRIO, ATP12A, MLL4, STK22B) were downregulated, while oxidative stress and inflammatory response genes were upregulated.
More recently, Nishimura found mouse hair follicles to age through defective renewal of hair follicle stem cells much in the manner as maintenance of melanocyte stem cells becomes incomplete with aging.
Hair production is fueled by stem cells, which transition between cyclical bouts of rest and activity. Aged hair follicle stem cells exhibit enhanced resting and abbreviated growth phases and are delayed in response to tissue-regenerating cues. Ultimately, aged hair follicle stem cells are poor at initiating proliferation and show diminished self-renewing capacity upon extensive use.
Aging-related loss of hair follicle stem cell marker expression starts well before hair follicles have shortened. Using genomic instability syndromes and exposure to ionizing radiation as models, Nishimura proposed an accumulation of DNA damage to be involved in the aging process (oral communication, 7th World Congress for Hair Research, May 4–6, 2013, Edinburgh, Scotland).
Finally, though being exceedingly rare, the progerias (Hutchinson–Gilford and Werner’s syndrome), together with other rare conditions associated with premature hair loss, such as the Laron syndrome and myotonic dystrophy (Curschmann–Steinert), give insights into the roles of telomeres, mitochondrial function, human growth hormone (HGH), and insulin-like growth factor 1 (IGF-1) for the growth and aging of hair.
Telomeres represent repeated TTAGGG DNA sequences that cap the end of the chromosomes. The 3’ end strand extends by 75–300 bases beyond the complementary 5’ strand of DNA, leaving a single-stranded G-rich overhang at the very end. This overhang forms a loop structure by inserting into the proximal telomeric double helix. With each cell cycle, the DNA polymerase is unable to replicate the outermost end of the DNA, resulting in progressive shortening of telomeres with each new cell division until they shorten to a critical length driving the cell into senescence, in which cell growth diminishes and then cell division stops altogether, or into apoptosis (programmed cell death) via the p53 pathway (Fig. 4.8).


Fig. 4.8
Intrinsic and extrinsic aging, telomere signaling, p53, and senescence versus carcinogenesis (From: Trüeb 2006a)
Telomere length and integrity of its loop structure are understood to be crucial in maintaining the stability of the chromosome and viability of the cell, respectively.
When telomere shortening reaches a critical point, signals are produced to induce cell senescence. Therefore, it is believed that the progressive shorting represents the internal clock that determines the onset of senescence of cells and therefore plays a pivotal role in intrinsic aging. Accordingly, older individual’s cells have shorter telomeres. In Werner’s syndrome, the defective gene product unwinds G-rich DNA structures and is implicated in telomere maintenance.
Environmental factors, on the other hand, play a major role in the process of extrinsic aging. A typical example that has been extensively studied in skin aging is UV radiation, which can lead to oxidative stress (UVA) and to mutations of coding DNA (UVB). Oxidative damage toward the GGG residues, produced through UV radiation, cigarette smoking, or cellular metabolism, may accelerate telomere shortening in order to counteract carcinogenesis. The p53 signaling pathway has many mechanisms of anticancer function: It can activate DNA repair mechanism. It can arrest growth by holding the cell cycle long enough for the DNA repair mechanisms to fix the damage allowing the cell to continue the cell cycle. Ultimately, it can initiate apoptosis if DNA damage proves to be irreparable.
Telomerase is an enzyme with reverse transcriptase activity (TERT) that is capable of adding telomeric sequences to the ends of the telomere, thereby preventing its shortening. In the hair follicle, its degree of activity is highest in the hair rapidly proliferating cells of the hair bulb, while it is low in the region of the hair follicle stem cells. Therefore, any damage to the hair follicle stem cell niche, elicited by any type of genotoxic injury, including UVR and chemotherapy, may lead to telomerase deficiency, resulting in shortened telomeres and cellular senescence. The importance of telomerase is underlined by the observation of premature hair graying, alopecia, and decreased wound healing in mice deficient of telomerase, a phenotype with analogies to progeria in humans. Vice versa telomerase is expressed in 90 % of all tumors. Therefore, telomerase appears to be stringently repressed in normal human somatic tissues but reactivated in cancer, where immortal cells are likely required to maintain tumor proliferation.
Mitochondrial damage, particularly as a result of oxidative injury, contributes to changes, such as deletions or mutations, in mitochondrial DNA. Analysis of plucked hair from participants ranging in age from 5 days to 91 years has revealed an increase in the incidence of a specific mitochondrial deletion over time, with 4,977 base-pair deletions by year 91. Moreover, smoking-associated mitochondrial DNA mutations have also been identified in human hair follicles.
The Laron syndrome (primary growth hormone insensitivity) sheds light on the role of HGH and IGF-1 for hair growth. The syndrome represents an autosomal recessive disorder characterized by insensitivity to growth hormone, caused by a variant of the growth hormone receptor. The principal feature of the syndrome is abnormally short stature (dwarfism). The hair of patients with Laron syndrome is thin and easy to pluck. Young adults have various degrees of alopecia, more pronounced in males. Accordingly, Olszeska et al. originally reported in their publication on methods of hair loss evaluation in patients with endocrine disorders, hair loss to be a symptom in growth hormone deficiency (Fig. 4.9). Not unexpectedly, scalp hair loss has been reported both with octreotide therapy for acromegaly and after transsphenoidal adenomectomy in patients with acromegaly. Finally, in the course of hormonal anti-aging protocols containing recombinant human growth hormone (hGH) at the Palm Springs Life Extension Institute, Chein claims improvement of hair thickness and structure in 38 % of patients, darkening of hair in some, and increased hair growth in few.


Fig. 4.9
Hair loss in primary growth hormone deficiency
Curschmann–Steinert’s myotonic dystrophy is an autosomal dominant disorder of trinucleotide repeat characterized by wasting of the muscles (muscular dystrophy), cataracts, heart conduction defects, endocrine changes, and myotonia. Myotonia is the designation for a peculiar form of muscle disorder characterized by delayed relaxation (prolonged contraction) of the skeletal muscles after voluntary contraction or electrical stimulation. Although early frontoparietal alopecia is one of the most common symptoms in myotonic dystrophy (Fig. 4.10a. b), it has not received the deserved attention.


Fig. 4.10
(a, b) Myotonic dystrophy Curschmann–Steinert. (a) Frontal alopecia and myopathic facies in an affected male. (b) Vertex alopecia in an affected female
Altering the delicate balance between cellular senescence and cellular proliferation and carcinogenesis by manipulating the respective signaling pathways with the aim to prevent or reverse aging seems rather precarious.
IGF-1 has shown some interesting results in studies examining its use as a hair growth-promoting agent. IGF-1 regulates cellular proliferation and migration during the development of hair follicles and is known to be regulated by androgens, and its deficiency is related to premature alopecia in the Laron syndrome. Dermal papilla cells from balding scalp follicles have been found to secrete significantly less IGF-1 than their non-balding counterparts, and the expression of IGF-1 in follicular dermal papillae has been shown to correlate with the therapeutic efficacy of finasteride in androgenetic alopecia.
Currently, commercial products containing IGF-1 exist for transdermal application using a dermaroller, usually in combination with other growth factors, such as bFGF (basic fibroblast growth factor), and VEGF (vascular endothelial growth factor), copper peptide, hyaluronic acid, coenzyme Q, vitamins B3, B6, and B5, and amino acids (arginine and lysine). However, their efficacy has as yet not been sufficiently substantiated in clinical studies published in peer-reviewed scientific publications.
Alternatively, liposomal IGF-1 and VEGF in topical gel formulation to promote hair growth have been studied in the hamster (Mesocricetus auratus): efficacy was determined by dermatoscopic analysis of hair density and microscopic analysis of hair diameter. Hair was found to grow faster and thicker in the 3 % liposomal IGF-1 and VEGF groups than in the respective 1 % or control groups.
Finally, another potential signaling pathway involved in the aging of hair as it relates to hair cycling is the bone morphogenic protein (BMP)-/calcium-regulated nuclear factor of activated T-cell c1 (NFATc1) pathway. Although the complex mechanisms underlying hair cycling and stem cell proliferation in humans remains ill-defined, the role of BMP and NFATc in hair growth and cycling has been unravelled in the mouse. NFATc1 is activated by BMP signaling, in turn leading to quiescence of stem cells. Transcriptional profiling presents differences consistent with defects in aged hair follicle stem cell activation. Notably, NFATc1 becomes recalcitrant to its normal downregulating cues. Given these findings, prolonged telogen phase in aging hair may be the result of increased expression of the NFATc1 transcription factor. Given the propensity for NFATc1 to put off stem cell proliferation, an inhibition of BMP signaling and/or NFATc1 results in increase of hair growth in the mouse model due to a decrease in the time that the stem cells spend in the quiescent state.
For the time being, current available treatment modalities for age-related hair loss remain topical minoxidil, and oral finasteride, though with some limitations. Moreover, older patients often suffer from a variety of conditions that may affect the hair: nutritional deficiency, endocrine disorders, psychological problems, and drug-related adverse effects. Therefore, one must remain suspicious of the possibility of a more general problem underlying the patient’s complaint in taking care of the elderly with hair problems.
It has been known for over 30 years that minoxidil stimulates hair growth, and clinical trials with topical 2 or 5 % minoxidil solution in male and female hair loss have all shown remarkably rapid increase in hair growth, measured by hair counts or hair weight: The increase is evident within 6–8 weeks of treatment and has generally peaked by 12–16 weeks.
Local application of minoxidil 2 % twice daily has also proven to be safe and effective for eyebrow enhancement.
However, topical minoxidil has not been studied in the specific perspective of aging and senescent alopecia. In an analysis of clinical trial data in 636 males and 630 females, a therapeutic benefit of topical 2 and 5 % minoxidil solution was compared to age, duration of balding, and diameter of balding vertex area in males and age and duration of hair loss in females: Age was found to be the denominator for predicting treatment success for both males and females. The younger subjects experienced better efficacy than the older subjects.
Nevertheless, clear treatment benefits of topical minoxidil solution are also noted in the older age group that has retained some hair.
Males showed an inverse relationship between effect and duration of balding. Males with duration of balding <5 years showed a significantly better effect than those with duration of balding >21 years. Females, in contrast, showed no correlation with duration of balding. The diameter of vertex balding in men showed an inverse relationship with efficacy of minoxidil. Males with <5 cm diameter vertex balding area showed a better effect of treatment than subjects with diameters >15 cm. Finally, duration of hair loss less than 1 year compared to more than 10 years at onset of treatment resulted in a significantly more effective treatment with respect to stabilization of alopecia and new hair growth.
One milligram oral finasteride daily has also proven to be effective in aging male, though with a lesser degree of efficacy and a higher frequency of sexual adverse effects compared to men between 18 and 40 years.
With respect to menopause and hormonal replacement therapy (HRT), the focus tends to be on the issues recently covered by the Women’s Health Initiative. Consequently, many women have become reluctant toward systemic estrogen substitution therapy. Alternatively, topical estrogen supplementation with estradiol has been suggested to be of some benefit. Unfortunately, these studies are relatively small. Also no literature exists comparing efficacy and safety of topical versus systemic estrogens in postmenopausal women.
As a general rule, hormone replacement therapies with progestogens that possess net androgenic activity, such as norethisterone, levonorgestrel, or tibolone, should be avoided, since they may precipitate alopecia.
Finally, oral 2.5–5 mg oral finasteride or 0.5 mg dutasteride may be tried in postmenopausal women not responsive or intolerant to topical minoxidil without the teratogenicity issues in premenopausal women, though with mixed results. Adverse effects include dry skin, libido reduction, and breast tenderness.
Thyroid hormones have influence on the growth and differentiation of many tissues and total energy expenditure of the organism and on the turnover of many substrates, vitamins, and other hormones. Thyroid activity affects oxygen consumption, protein synthesis, and mitosis and is therefore essential for the formation and growth of hair. Schell et al. originally demonstrated by means of DNA flow cytometry the influence of thyroid hormones on in vivo cell cycle kinetics of human scalp hair bulbs. Expression of the thyroid hormone receptor beta1 was demonstrated in the human hair follicle, and it was shown that triiodo-L-thyronine significantly enhances human hair survival in vitro.
The impact of thyroid hormone activity on the hair is most notable during deficient (hypothyroidism) or excess (hyperthyroidism) states. Thyroid dysfunction represents a frequent disorder related to hair loss, especially in women: hypothyroidism is about ten times more frequent in women than in men and particularly affects women between the ages of 40 and 60 years. Dull, coarse, brittle hair or diffuse alopecia may be present with particular thinning of the lateral eyebrows. The hair growth rate is slowed, with an increase in the proportion in telogen. The alopecia is of very gradual onset. Such symptoms may easily be overlooked or ascribed merely to aging. Also, there is an increasing prevalence of high levels of TSH with age, particularly in postmenopausal women, while the symptoms of thyroid disease can be similar to postmenopausal complaints. Finally, HRT in women with hypothyroidism treated with thyroxine causes changes in free thyroxine and TSH. Increased binding of thyroxine to elevated thyroxine-binding globulin causes an elevation of TSH by feedback. Since adaptation is insufficient, there is an increased need for thyroxine in women taking HRT.
Long-standing hypothyroidism is often associated with patterned hair loss. The mechanism has been presumed to be due to an increase in plasma-free androgens. While normally, the hair shows a Gauss distribution of shaft diameters with a peak at 80 μm, in both androgenetic alopecia and hypothyroidism, hair shaft diameters are decreased with a bimodal distribution, suggesting that a genetic predisposition to patterned hair loss may be preliminary to the development of alopecia of hypothyroidism.
Currently, thyroid receptor agonists are in development for the treatment of androgenetic alopecia. A thyroid receptor beta subtype-selective thyromimetic 5 was found to be efficacious in both mouse and monkey hair growth models after topical application.
Coenzyme Q10 (CoQ10) has previously been used for its anti-aging effects on aging skin, where it has been theorized that there is an increase in anaerobic metabolism due to a decrease in mitochondrial function as a result of oxidative damage. Expanding the cutaneous application to the hair, it has been shown with quantitative polymerase chain reaction techniques that CoQ10 administration results in an increase in particular keratins in the hair root compared to placebo. Specific keratin and keratin-associated protein levels have been found to be decreased in aged hair compared to fibers from a younger individual. A decrease in keratin levels correlates with a decrease in interactions between the filaments and keratin-associated proteins resulting in a decline in the mechanical strength of the hair fiber.
However, as with all cosmetic anti-aging treatments, the question arises whether and to what extent a quantifiable improvement in a particular parameter measured translates into improvement perceived by the consumer.
The efficacy of hair growth-promoting agents or anti-aging substances in shampoo form is questionable given their dilution with water and short contact time, unless they can be absorbed in effective quantities.
Nevertheless, cosmetic products represent a key integral part of management of aging hair, since they may significantly improve the condition of the hair fiber with more immediate effects, while pharmacologic agents take their time.
Since hair fiber diameter has been recognized to represent another key contributor to hair thinning, a novel leave-on technology has been developed at Procter & Gamble that combines caffeine, niacinamide, panthenol, dimethicone, and an acrylate polymer (CNPDA) to affect the diameter and behavior of individual scalp hair fibers as an approach to improve decreasing fiber diameter. Utilizing laser scan micrometer for assessment of fiber diameter measures and tensile break stress and torsion pendulum testing for assessment of behavioral properties, it was found that CNPDA significantly increased the diameter of individual terminal scalp hair fibers by 2–5 μm, which yields an increase in the cross-sectional area of approximately 10 %. Beyond the increase in hair fiber diameter, the CNPDA-thickened fibers demonstrated enhanced mechanical properties characteristic of thicker fibers, such as increased suppleness/pliability and better ability to withstand force without breaking.
Although cosmetic treatments will not reverse the process of hair aging, new technologies may help to mitigate the effects of age-related thinning of hair.
Finally, there are a number of botanicals with alleged anti-aging effects from both traditional Chinese medicine (TCM) and from Ayurvedic medicine (the literal meaning of Ayurveda is “life-knowledge”). Asia, Korea, China, and Japan have legally adopted the traditional Oriental medical system along with the Western system. A number of traditional herbal drugs including the polypharmacy type of prescription (combination of multiple herbs) are available and widely dispensed. This polypharmacy type of herbal therapy allegedly exhibits holistic effectiveness by exerting multitargeted effects. The Traditional Oriental Medicine Database (TradMed 2000 DB) represents a database of traditional Oriental herbal therapy containing specific information such as formulas, chemical information on ingredients, botanical information on herbal materials, and a dictionary of disease classifications. Using the TradMed 2000 DB, Chang from Seoul National University, Korea, identified more than a dozen herbs with anti-aging effects used in traditional Oriental herbal therapy, of which Polygonum multiflorum (Ho Shou Wu: the name literally means “Mr. Ho’s hair is black.” The name refers to the story of a 58-year-old man named Ho, whose gray hair returned to its black color after taking the herb. He also became more youthful and was able to father several children. Supposedly he lived to become 160, retaining his black hair), and Ginseng Radix (Ren Shen: Fig. 4.11) probably represent the commercially most utilized in hair cosmetics, also on the contemporary Western market.


Fig. 4.11
Panax Ginseng. The Ginseng root is among the most prized among traditional Oriental herbal remedies based upon a similia similibus type of selection for its anthropomorphic form (Gift from Alexander Navarini, MD, PhD, University Hospital of Zurich)
However, the effective constituents of the traditional herbal remedies have not been fully elucidated, though there is a great opportunity to study them systematically with respect to current research trends in anti-aging.
Whatever the cause, the follicle tends to behave in a similar way. To grasp the meaning of this generalization requires understanding of the hair cycle and its derangements.
4.2.3 Telogen Effluvium
Diffuse shedding of hair has originally been called “defluvium capillorum.” In 1932 Sabouraud restricted the term to sudden diffuse loss of hair following shortly after a severe emotional shock, while others applied it to all forms of alopecia. In 1961 Kligman revealed the pathodynamics of one common pattern of response of hair follicles to a variety of insults and named it telogen effluvium.
As originally proposed by Mildred Trotter, the hair follicle is subject to constant turnover in the course of perpetual cycles through phases of proliferation in anagen, involution in catagen, and resting in telogen, with regeneration in the successive hair cycle (Fig. 4.12a).


Fig. 4.12
(a, b) Hair cycle. (a) As originally described by Mildred Trotter. (b) Extended concept, including kenogen, exogen, and neogen
It is a major characteristic of anagen (duration: 2–6 years) that not only the hair shaft is growing but that most epithelial hair follicle compartments undergo proliferation, with the hair matrix keratinocytes showing the highest proliferative activity.
During catagen (duration: 2 weeks), hair follicles enter a process of involution that is characterized by a burst of programmed cell death (apoptosis) in the majority of follicular keratinocytes. The resulting shortening of the regressing epithelial strand is associated with an upward movement of the follicle.
In telogen (duration: 3 months), the hair shaft matures into a club hair, which is held tightly in the bulbous base of the follicular epithelium, before it is eventually shed. It was unresolved, whether shedding of the telogen hair (teloptosis) is an active, regulated process or represents a passive event that occurs at the onset of subsequent anagen, as the new hair grows in.
Cyclic hair growth activity occurs in a random mosaic pattern with each follicle possessing its own individual control mechanism over the evolution and triggering of the successive phases, though systemic factors as well as external factors linked to the environment have influence, such as:
Hormones
Cytokines and growth factors
Toxins
Deficiencies of nutrients, vitamins, and energy (calories)
Finally, there are considerable variations in length of these phases depending on the body site location, with the duration of anagen determining the type of hair produced, particularly its length. In general, the anagen phase is longer in women than in men.
On the scalp, hairs remain in anagen for a 2- to 6-year period of time, whereas that of telogen is approximately 100 days, resulting in a ratio of anagen to telogen hairs of 9:1. On average, the amount of new scalp hair formation matches the amount that is shed, thereby maintaining a consistent covering. With a range of 75,000–150,000 hairs on the head, the reported average daily telogen hair shedding varies from 35 to 180 hairs.
Since the original description of the hair growth cycle, additional phenomena relevant to hair growth and shedding have been recognized (Fig. 4.12b): In 1996, Guarrera et al. observed that anagen hairs may fail to replace telogen hairs in androgenetic alopecia. By using the phototrichogram, a novel phenomenon was discovered: emptiness of the hair follicle following teloptosis. Rebora chose to call this phenomenon kenogen, deriving from the Greek word for “empty.” During kenogen, the hair follicle rests physiologically, but duration and frequency were shown to be greater in androgenetic alopecia, possibly accounting for baldness. In addition to the classical cycle, the hair follicle may follow an alternative route during which the telogen phase, not accompanied by a coincident new early anagen, ends with teloptosis leaving the follicle empty.
In 2002, Stenn et al. recognized the shedding phase of the hair growth cycle, then renamed exogen, to be a uniquely controlled final step in the hair cycle involving a specific proteolytic step.
Finally, in 2013 Bernard et al. from L’Oréal Research and Innovation laboratories identified hypoxia markers in the human hair follicle stem cells and proposed hypoxia signaling mediated by the hypoxia-inducible transcription factor HIF1 to be important for reentry of the follicle into a new hair cycle in the course of a novel neomorphogenic hair cycle phase, named neogen. Ultimately, it was further hypothesized that molecules that mimic hypoxic signaling may figure as a new approach to sustain hair growth and cycling.
Therefore, besides the well-recognized systemic and external factors linked to the environment (hormones, cytokines, toxins, and nutrients), the local milieu at the level of the stem cells, such as hypoxia, has been recognized to also impact hair cycling.
Consequently, it seemed conceivable to develop a biomimetic of the effects of a hypoxic local environment to optimize functionality of hair follicle stem cells. Pyridine-2,4-dicarboxylic acid diethyl ester (Stemoxydine®) 5 % in a hydroalcoholic solution applied daily represents the first compound developed at L’Oréal Research and Innovation laboratories as a competitive inhibitor of hypoxia-inducible transcription factor (HIF1) that is functionally able to induce hypoxia-like signaling in vitro and results in hair kenogen phase shortening with an increase in visible scalp hair density in vivo.
Many factors can lead to pathologically increased hair loss. The pathologic dynamics of hair loss can be related to disorders of hair cycling.
Telogen effluvium is by far the most common cause of hair loss and results from increased shedding of hairs from the telogen phase of the hair cycle. An increase in the percentage of follicles in telogen >20 % leads to a pathological increased shedding of normal club hairs.
In Kligman’s original description, telogen effluvium is an acute and diffuse hair loss brought about by a variety of triggers. Clinical experience, however, proves that chronic telogen effluvium also exists. It is defined as diffuse telogen hair loss that persists longer than 6 months.
While a number of attempts have been made with respect to the underlying pathologic dynamics of telogen effluvium and its classification, Headington’s original classification of the functional types of telogen effluvium remains unabated the most comprehensive.
Telogen effluvium may result either from synchronization phenomena of hair cycling or from decrease of the anagen phase duration.
On the basis of the changes in different phases of the follicular cycle, Headington proposed further classification of telogen effluvium into five functional types.
In immediate anagen release, follicles would normally complete a longer cycle by remaining in anagen prematurely enter telogen. It is a very common form of telogen effluvium, typically occurring after periods of physiologic stress including episodes of high fever. In fever, the pyrogens, basically circulating cytokines, drive the hair follicle keratinocytes into apoptosis initiating catagen with following telogen. Because the shedding is dependent on transition from anagen through catagen and telogen with subsequent release of telogen hairs, hair loss occurs 3–4 months after the inciting event. A host of different triggers has been implicated and identifies the clinical species of the genus, e.g., post-febrile, posttraumatic, post-interventional, psychogenic effluvium, etc. Severe febrile illness, accidental trauma or surgical operations with a large hemorrhage, a crash diet, and severe emotional distress are among the most common causes. Most are self-limiting and will undergo normal reversal.
A careful patient history with respect to the chronology of events usually reveals the diagnosis and the cause.
In delayed anagen release, hair follicles remain in prolonged anagen rather than cycling into telogen. When finally released from anagen, the clinical sign of increased shedding of telogen hair will be found. This type of telogen effluvium underlies postpartum hair loss. During the second half of pregnancy, the percentage of anagen hairs increases from the normal 85–95 %; at this time also the percentage of hairs of large shaft diameter is higher than in nonpregnant women of the same age. After partuition the follicles, in which anagen has been prolonged, rapidly enter catagen and then telogen, with an increased shedding of hair evident after 3–4 months.
Most women will return to their usual hair growth cycle and pre-pregnancy thickness of hair between 6 and 12 months after birth. In case of persistent postpartum effluvium (>12 months), excessive hair loss may be caused by common conditions, such as female androgenetic alopecia, iron deficiency, or hypothyroidism. Lesser common conditions include persistent hyperprolactinemia (Chiari–Frommel syndrome) and postpartum hypopituitarism (Sheehan syndrome) caused by pituitary necrosis due to blood loss and hypovolemic shock during childbirth.
In immediate telogen release, hair follicles normally programmed for release of the club hair after an interval of usually 100 days after the end of anagen are prematurely stimulated to cycle into anagen. There is premature teloptosis. This type of telogen effluvium underlies the shedding of hair upon initiation of therapy with topical minoxidil solution (shedding phase).
Seemingly ironic, upon initiation of treatment with the hair growth-promoting agent minoxidil, patients frequently experience increased shedding of hair. Patients should be prepared and informed that this represents a physiological response to treatment, since minoxidil not only increases the duration of anagen but triggers an immediate telogen release.
In delayed telogen release, hair follicles remain in prolonged telogen rather than being shed and recycling into anagen. When finally teloptosis sets in, again the clinical sign of increased shedding of club hairs is observed. This process underlies molting in mammals and probably also seasonal shedding of hairs in humans or mild telogen effluvia following travel from low-daylight to high-daylight conditions. The cyclical activities of the hair follicle is the mechanism by which mammals change their coat of hair to meet the exigencies of growth, seasonal changes in the ambient environment, and, perhaps, normal wear and tear. It seems likely that environmental factors, such as the photoperiod, mediate through the optic pathway and the neuroendocrine system coat phenotype and function to photoperiod-dependent environmental changes. Originally, Orentreich reported three women in New York who experienced maximum hair loss in November. Studying a group of 14 men during 18 months, Randall and Ebling showed that the proportion of telogen hair and of hair shedding were maximal in September. Courtois et al. observed ten men, with or without alopecia, for a period of between 8 and 14 years and also demonstrated a maximal proportion of telogen hairs at the end of summer. Some subjects also exhibited a periodicity approximately corresponding to two annual peaks. Ultimately, Kunz et al. published a study of 823 otherwise healthy women with telogen effluvium observed over a period of 6 years and demonstrated the existence of overall annual periodicity in the growth and shedding of hair, manifested by a maximal proportion of telogen hairs in July. A second peak seemed to exist, although less pronounced, in April (Fig. 4.13a). The telogen rate was lowest toward the beginning of February. The fact that human hair follicles, just as those of other mammals, undergo cyclical activity and are influenced by hormones implies that human hair is not unaffected by these phenomena.


Fig. 4.13
(a, b) Seasonality of hair growth and shedding. (a) Fluctuations in frontal telogen rates (n = 823) in relation to the day of the year in women complaining of hair loss (From: Kunz et al. 2009) (b) Fluctuations of shed hair in a 39-year-old healthy male, counted between 1996 and 2011. The mean counted values were plotted in relation to the month of the year
From an evolutionary point of view, the maintenance of the low winter level of hair shedding and the postponement of hair fall until the end of summer might, perhaps, are postulated as having a selective advantage with respect to isolation of the head against the cold in winter and protection of the scalp against the midday sun in summer, respectively.
Finally, a 39-year-old long-haired male presented at the Center for Dermatology and to Hair Disease Professor Trüeb with early androgenetic alopecia. He had counted his hairs shed in the course of hair washing every 3 days from the years 1996–2011 and plotted their mean value against the month of the year (Fig. 4.13b). There was a striking congruence with the graphic formerly demonstrated for women by Kunz et al., which proves that men are subject to the same seasonal changes of hair growth and shedding as women.
The difference is that seasonal hair shedding is less noticeable in men, who commonly wear their hair shorter. Moreover, men usually have a lesser sensitivity than women for the condition of their hair.
Finally, the existence of a short anagen phase was proposed by Headington in his classification of telogen effluvium. Headington suggested the existence of a mild form of persistent telogen effluvium in association with decreased hair length due to a short anagen phase. The existence of short anagen hair as an isolated disorder in otherwise healthy children was later confirmed by Barraud-Klenovsek and Trüeb, who reported two children with a peculiar type of isolated congenital hypotrichosis. Both presented with persistent short, fine hair since birth (Fig. 4.14). Evidence was provided that the short hair observed in these patients was due to a short anagen phase of the hair cycle, with a normal rate of hair growth. Shortening of the anagen phase of the scalp hair cycle leads to a decrease in the maximal hair length and an increase in the number of hairs in telogen, resulting in an increase in hair shedding. Alternatively, Sinclair provided evidence of short anagen hair with a persistent synchronized pattern of scalp hair growth in a 4-year-old boy. One patient had affected family members with an apparently autosomal dominant mode of inheritance. The disorder appears to resolve spontaneously during puberty and adulthood. The syndrome of short anagen hair was subsequently confirmed by Olsen who proposed methods for diagnosing this entity by clinical examination, trichogram, light microscopic examination of the hair shaft, scalp biopsy, and measurement of scalp hair growth rate.


Fig. 4.14
(a, b) Short anagen hair
Short anagen hair appears to be an uncommon though probably underreported condition, whose incidence is poorly documented in the medical literature. The most important differential diagnosis includes short anagen hair in trichodental syndrome, loose anagen hair, hereditary hypotrichosis simplex, and premature androgenetic alopecia. Treatment is not necessary, though one would expect—as in androgenetic alopecia—efficacy of topical minoxidil from reported successful treatment of a short anagen hair nevus.
Chronic telogen effluvium. Diffuse shedding of telogen hairs that persists longer than 6 months either represents a primary disorder and is then a diagnosis of exclusion, or it is secondary to a variety of identifiable systemic disorders, such as iron deficiency, thyroid dysfunction, systemic lupus erythematosus, and syphilis. In comparison, other dietary deficiencies, metabolic diseases, connective tissue disorders, and malignant or chronic infectious diseases are infrequent causes of hair loss in office-based clinical practice. Drugs known to cause hair loss, such as anticoagulants (heparin, warfarin), oral retinoids (acitretin, isotretinoin), interferon, agents with antithyroid action (carbimazole, propylthiouracil, amiodarone), hypolipidemic agents (fibrates), colchicine, antimetabolites (methotrexate, azathioprine, cyclophosphamide), and hormones with pro-androgen action (norethisterone, levonorgestrel, tibolone). Finally, dietary habits should be addressed. Especially in young women, if anorexia nervosa/bulimia is suspected, the aid of a psychiatrist should be sought; in other cases, the informed patient will be happy to abandon any dietary culprit.
The cause of chronic telogen effluvium may be multifactorial and difficult to establish.
While symptomatic telogen effluvium is triggered by one or several identifiable events, in primary chronic telogen effluvium, no specific trigger is evident. It predominantly affects women, while men with short hair tend not to notice increased hair shedding. The presentation of this type of diffuse hair loss tends to be distinctive and was originally described 1959 in detail by Guy and Edmundson as “diffuse cyclic hair loss in women” and revived by Whiting in 1996, who additionally characterized histopathologic features differing the condition from androgenetic alopecia. The typical patient is a vigorous otherwise healthy woman between 30 and 60 with a full, thick head of hair. On examination there are some bitemporal thinning (Fig. 4.15a) and a positive hair pull test equally over the vertex and occiput. There is no widening of the central part, as is common in androgenetic alopecia. Nevertheless, patients are adamant that they previously had more hair and are distressed by the prospect of going bald. Many frequently bring large balls of hair for inspection (Fig. 4.15b) but despite this do not show any obvious balding. The condition tends to run a fluctuating course. It is has been proposed that the disorder may be due to synchronization phenomena of the hair cycle, shortening of the anagen phase, or premature teloptosis.


Fig. 4.15
(a, b) Chronic telogen effluvium: (a) Temporal thinning of hair. (b) Ball of shed hair
On the long run, chronic telogen effluvium appears to be self-limiting, and it is important to reassure patients that this condition represents exaggerated shedding rather than actual hair loss.
In an attempt to find a simple method to evaluate reliably the diagnosis and activity of androgenetic alopecia and telogen effluvium, Guarrera et al. adopted the modified wash test, which accomplishes such a task through the assessment of the number of shed hair and the vellus percentage. As in the wash test, 5 days after the last shampoo, the patient is instructed to wash the hair in the sink with its drain covered by a gauze. The hairs entrapped in the gauze are then collected, counted, and divided into ≤3 and ≥5 cm in length. The test demonstrated that in female with androgenetic alopecia the proportion of vellus hairs is significantly higher than in chronic telogen effluvium (58.9 % versus 3.5 %).
Nevertheless, differential diagnosis of chronic telogen effluvium from androgenetic alopecia is often complicated through considerable overlap. Ultimately, synchronization phenomena of hair cycling, often on a seasonal basis, are more pronounced in androgenetic alopecia, since with a shorter anagen phase there is a tendency to more pronounced synchronization of hair follicles.
More recently, an inflammatory or “autoimmune” type of telogen effluvium has been proposed on the basis of the observation of a high frequency of associated scalp dysesthesia (trichodynia), associated autoimmune phenomena (Hashimoto’s thyroiditis), and response to topical corticosteroid treatment.
Up to date, histopathological evidence is lacking for the presence of follicular inflammation in telogen effluvium (as opposed to androgenetic alopecia). In 2002, Sato-Kuwamora et al. suggested naming a peculiar type of inflammatory non-cicatricial alopecia that is characterized by marked female predominance and a uniquely short clinical course acute diffuse and total alopecia of the female scalp. It is basically identical with a subtype of alopecia areata presenting with diffuse hair loss as originally proposed in the German literature by Braun-Falco and Zaun 40 years earlier in 1962.
Acute diffuse and total alopecia of the female scalp, alopecia areata incognita (yet another synonymous designation proposed by Rebora in 1987), and diffuse alopecia areata all represent the same alopecia areata variant that is characterized by diffuse hair shedding in the absence of the patches otherwise typical for the disease. The condition usually affects women over 40 years of age, who complain of diffuse alopecia and may be misdiagnosed as having telogen effluvium.
The presence of yellow dots and short regrowing miniaturized hairs seen in the terminal hair-bearing scalp by trichoscopy, as well as associated autoimmune phenomena (Hashimoto’s thyroiditis and/or circulating antithyroid and anti-parietal cell antibodies), represents important clues to the diagnosis. The diagnosis is usually confirmed by histopathology and/or usually excellent response with complete hair regrowth to a course of systemic corticosteroids.
Ultimately, the designation inflammatory telogen effluvium should be reserved for telogen effluvium related to inflammatory conditions of the scalp, such as severe seborrhoeic dermatitis, acute allergic contact dermatitis (usually to PPD in hair coloring agents), erythroderma (of psoriasis or lymphoma: Fig. 4.16), or interface dermatitis from lupus erythematosus or dermatomyositis.


Fig. 4.16
Diffuse inflammatory effluvium due to erythroderma (Sézary syndrome)
Treatment of telogen effluvium is quite straightforward: Acute telogen effluvium is usually self-limiting (within 6 months by definition), providing that the culprit has been identified and eliminated.
Reassuring patients that they are not going bald and that the telogen effluvium is temporary is usually sufficient. If the cause is not obvious from the patient’s history, iron studies, thyroid function tests, syphilis serology, and an antinuclear antibody titer should be performed. A drug history and in women a change in the contraceptive pill or hormone replacement therapy should be inquired about, as these are common causes of telogen effluvium related to female androgenetic alopecia.
Recommendations for treatment of primary chronic telogen effluvium are scanty and include topical or systemic corticosteroids, topical minoxidil, and dietary supplements on the basis of millet extract, pantothenic acid, biotin, their combination, or combinations of L-cystine, medicinal yeast, and pantothenic acid (CYP-complex).
Finally, the issue of overvalued ideas in relation to the condition of the hair is not always easy to resolve; however, it is important to control stress as a complication of hair loss or fear of hair loss. For this purpose, strong psychological support is essential to help limit patient anxiety, and patients need to be educated about the basics of the hair cycle. Information about the hair cycle can be useful to explain how hair loss not related to cycle shortening (as in androgenetic alopecia) generally precedes new regrowth and why considerable patience is required for effective cosmetic recovery.
Most importantly, women with chronic telogen effluvium need to be reassured that it represents exaggerated shedding rather than actual hair loss and that the shed hair is mostly being replaced; therefore, the risk of total baldness is remote.
4.2.4 Alopecia Areata
Novalis (Georg Philipp Friedrich von Hardenberg, poet, author, and philosopher of early German Romanticism, 1772–1801) wrote: “when we dream that we are dreaming, the moment of awaking is at hand.” I personally realized the extent of psychological traumatism resulting from the loss of hair in clumps on the occasion of a respective dream during a lecture tour on hair. I dreamt awaking in the morning before my presentation in the hotel room and pulling out hairs in clumps in front of the bathroom mirror when attempting to groom them. In that moment I realized it was alopecia areata but almost simultaneously also that it must be a dream.
Since hair is a power symbol, as the Biblical Samson teaches us in Judges 16, it is customary that hair falling out in dreams is interpreted as loss of power and control. The same type of helpless feeling is experienced in waking life when hair is lost. The hair loss dream is typically a manifestation of a stressful situation. Ultimately, hair dreams represent a situation that is beyond one’s control.
Alopecia areata is considered to be of autoimmune origin with an organ-specific, T-cell-mediated assault on the hair follicle at the level of the bulb. The hair bulb is an immune protected site with deficient MHC expression. There is circumstantial evidence suggesting that alopecia areata results from loss of immune privilege with presentation of autoantigens. A peribulbar lymphocytic infiltrate induces hair follicle keratinocytes to undergo apoptosis resulting in inhibition of cell division in the hair matrix. It represents the most frequent cause of dystrophic anagen effluvium, either localized or diffuse, occurring in the otherwise healthy child or adult. It is a common hair loss condition characterized by an acute onset of non-scarring hair loss in usually sharply defined areas. Any hair-bearing area can be affected, but the most noticeable are the scalp, the beard area, and the eyebrows. The characteristic patch of alopecia areata is usually round or oval and is completely bald and smooth. Trichoscopic examination reveals yellow dots, dystrophic hairs (cadaverized hairs, exclamation mark hairs), and/or short regrowing miniaturized hairs.
The progress of alopecia areata in an individual patient is unpredictable, though a large surface area, a long disease duration, and associated nail abnormalities have been connected with a poorer prognosis.
Some patients lose hair in only a small patch, while others may have more extensive or less frequently diffuse involvement. Alopecia totalis is the loss of all scalp hair; alopecia universalis is the loss of all scalp and body hair.
A recent meta-analysis of published trials on treatment of alopecia areata states that only few treatments have been well evaluated in randomized trials. The authors found no randomized controlled trials on the use of diphenylcyclopropenone (DCP), intralesional corticosteroids, or dithranol, although commonly used in clinical practice. Although topical steroids and minoxidil are widely prescribed and appear to be safe, there is no convincing evidence that they are beneficial in the long term. Most trials have been reported poorly and are so small that any important clinical benefits are inconclusive. Of 17 trials including 6–85 participants with a total of 540 participants assessing a range of interventions that included topical and oral corticosteroids, topical ciclosporin, photodynamic therapy, and topical minoxidil, none showed significant treatment benefit in terms of hair growth when compared with placebo. The authors came to the conclusion that considering the possibility of spontaneous remission, especially for those in the early stages of the disease, the options of not being treated therapeutically or, depending on individual preference, of wearing a wig may be alternative ways of dealing with this condition.
Nonetheless, depending on patient age, surface area, and disease duration, a treatment algorithm can be designed (Fig. 4.17). Any treatment of alopecia areata should fulfill the following criteria:


Fig. 4.17
Algorithm for treatment of alopecia areata, depending on patient age, surface area, and disease duration
Remission rates superior to the spontaneous remission rates of alopecia areata
Proof of efficacy in half side treatment of alopecia totalis or universalis
Good safety profile with minimal toxicity
The spontaneous remission rates for patchy alopecia areata are one third within 6 months, half within 1 year, and two thirds within 5 years; thereafter, complete remissions are rare. Recurrence rates within 5 years are 80 % and within 20 years 100 %. Total remission rates for alopecia totalis or universalis with a disease duration of 5 years or more are 1 % in children and less than 10 % in adults.
Single patches of alopecia areata are best treated with intralesional triamcinolone acetonide 10 mg/ml by jet injector or insulin syringe on a monthly basis, for an average of three to a maximum of six consecutive treatments (in children 5 mg/ml, for eyebrows 2.5–5 mg/ml, and for beard 5 mg/ml by insulin syringe).
Since patchy alopecia areata is the most prevalent form of the disease and intralesional corticosteroid injections are the most frequently practiced treatment for this condition, Samrao et al. conducted a retrospective cross-sectional case series on 18 patients with patchy alopecia areata treated at 4- to 8-week intervals with intralesional triamcinolone acetonide for at least 20 months to evaluate the effect on bone mineral density (BMD). Patients were evaluated for BMD using dual-energy X-ray absorptiometry (DXA). Follow-up DXA measurements were obtained in those with abnormal findings. Nine out of 18 patients (50 %) had abnormal DXA results. Patients with the following risk factors were more likely to have abnormal BMD: age older than 50 years, body mass index less than 18.5 kg/m2, lack of weight-bearing exercise, smoking history, postmenopausal status, past stress fracture, family history of osteopenia or osteoporosis, and a cumulative intralesional triamcinolone acetonide dose of greater than 500 mg.
The authors concluded that patients with patchy alopecia areata who receive chronic intralesional triamcinolone acetonide therapy should be counseled on preventive measures for osteoporosis and monitored for effects on BMD.
In an attempt to circumvent side effects related with systemic corticosteroid therapy for widespread disease, pulsed administration of corticosteroids has been proposed in the treatment of alopecia areata: Sharma originally proposed 300 mg oral prednisolone pulses at 4-week intervals, for a minimum of four doses or until cosmetically acceptable hair growth was obtained. 58.3 % of patients with widespread alopecia areata showed cosmetically acceptable hair growth. Response was evident on average after 2–3 months of therapy. In a subsequent study, Sharma and Muralidhar reported efficacy and safety of monthly oral corticosteroid pulse therapy also for treatment of widespread alopecia areata in young patients (up to 18 years of age), including children. Children aged less than 12 years received betamethasone sodium phosphate as soluble equivalent to prednisolone 5 mg/kg body weight every month. Side effects were minimal and included transient giddiness, headache, and epigastric burning.
Subsequently, Kar et al. confirmed in the first placebo-controlled study usefulness of oral prednisolone pulse therapy in alopecia areata.
Agarwal et al. alternatively suggested twice-weekly 5 mg betamethasone oral pulse therapy on two consecutive days per week for a total duration of 12 weeks.
To determine the effectiveness of an intravenous pulse of methylprednisolone, much in the same way as for treatment of other autoimmune diseases, Friedli et al. originally performed an open prospective study of patients with rapid and extensive hair loss for less than 1 year (first occurrence or relapse) with the bald area exceeding 30 % of the scalp. Two hundred and fifty milligram intravenous methylprednisolone was administered twice a day on three consecutive days. A single series of intravenous pulse of methylprednisolone was well tolerated and appeared to be effective in patients with rapidly progressing extensive multifocal alopecia areata, but not those with ophiasic and universal alopecia areata.
Subsequently, Nakajima confirmed the efficacy of intravenous pulse of methylprednisolone in a larger study of 139 patients aged >15 years with alopecia areata. With intravenous pulse corticosteroid therapy (500 mg methylprednisolone on three consecutive days, in three cycles, 4 weeks apart) within 6 months of disease onset, remission rates were 88 % for multilocular alopecia areata with surface area <50 %, 59.4 % with surface area >50 %, and 21.4 % in alopecia totalis/universalis. After 6 months of disease onset, the remission rate was 15.8 %.
Finally, Im et al. studied the outcome in 70 patients with severe alopecia areata treated with intravenous methylprednisolone pulse therapy on three consecutive days. All of patients had rapid and extensive hair loss with the bald area exceeding 50 % of the scalp. Seventy percent of patients showed terminal hair growth, and 41.4 % complete recovery with acceptable cosmetic results.
The prognostic factors that influenced successful outcome of i.v. methylprednisolone pulse therapy of alopecia areata were disease duration before treatment in relation to the type of alopecia areata. A good response was obtained for all types of alopecia areata with a duration of 3 months or less before treatment and for the multifocal type of alopecia areata with a duration of <6 months.
Tosti et al. evaluated topical clobetasol for treatment of widespread and long-standing (severe and refractory) alopecia areata. With 0.05 % topical clobetasol ointment under occlusion (Saran wrap) on six consecutive nights per week over 6 months, regrowth of hair was achieved in alopecia totalis or universalis in 17.8 %. Negative prognostic features for treatment success were positive family history for alopecia areata, first manifestation of disease before the age of 10 years, and association with atopic disease or autoimmune thyroid disease. Folliculitis and acne were frequent side effects. Despite positive clinical results obtained using clobetasol ointment with occlusive dressing, this approach has a low patient compliance, especially in patients with residual hair. Therefore, in a subsequent study, 0.05 % clobetasol foam was applied twice daily on five consecutive days per week and proved to also be effective, safe, and well tolerated, with good cosmetic acceptance and patient compliance. Folliculitis occurred in a smaller proportion of patients. No significant modifications in cortisol and ACTH blood levels were observed.
In summary, the art of using corticosteroids for treatment of alopecia areata is maximizing efficacy and minimizing toxicity.
Figure 4.18a–c illustrates typical cutaneous adverse effects from the use of intralesional or high-potency topical corticosteroids.


Fig. 4.18
(a–c) Typical cutaneous adverse effects from corticosteroid treatments: (a) subcutaneous atrophy after steroid injection in eyebrow area, (b) Bateman’s purpura from occlusive clobetasol propionate treatment for alopecia areata in a 76-year-old female patient, (c) steroid-induced striae distensae rubrae (stretch marks) of upper arms from uncontrolled use of clobetasol propionate ointment for treatment of alopecia areata in a 38-year-old male patient. The patient also had evidence of adrenal suppression
Topical immunotherapy has been used since the 1970s to treat dermatologic conditions understood to result from an altered immunological state, mainly widespread, long-standing alopecia areata and resistant viral warts. Any of three universal contact sensitisers may be used for topical immunotherapy, but diphenylcyclopropenone (D(P)CP) has advantages over dinitrochlorobenzene (DNCB) and squaric acid dibutylester (SADBE). In a retrospective study of 68 patients with severe alopecia areata (>40 % scalp hair loss) treated for at least 5 months with topical DCP, Pericin and Trüeb found an overall response rate of 70.6 % with 30.9 % complete remission and 39.7 % partial remission. Among the investigated prognostic factors for the outcome of DCP therapy, only the extent of alopecia areata at the time of initiation of treatment was found to be of significance. Total remission rates for multilocular alopecia areata were 43.8 %, for subtotal alopecia areata and ophiasis 33.3 %, and for alopecia totalis or universalis 21.4 %, irrespective of disease duration. Subsequent authors confirmed that topical immunotherapy with DCP is effective and usually well tolerated. The extent of hair loss before therapy is the main predictor for therapeutic success. However, DCP therapy is associated with a high relapse rate of which patients should be informed. A long period of therapy is needed and may increase the percentage of responders, especially in alopecia totalis and universalis. Maintenance therapy is recommended to reduce the risk of relapse.
Ultimately, Tosti et al. found that severity of alopecia areata at time of first consultation is an important prognostic factor, response to topical immunotherapy may be associated with better prognosis, in children the prognosis is worse, and alopecia areata may worsen over time.
Finally, it must be borne in mind that DCP is photosensitive, which is why the substance must be stored in the dark, and the scalp must be protected from light after application of DCP until contact dermatitis is elucidated.
Side effects of DCP therapy include itching, blistering, cervical lymphadenopathy, dyschromia in confetti (Fig. 4.19a), vitiligo, erythema multiforme-like eruption (Fig. 4.19b), exacerbation of (pre-existent) discoid lupus erythematosus, (contact) urticaria, and lentiginous eruption.


Fig. 4.19
(a, b) Adverse effects from treatment of alopecia areata with DCP. (a) Dyschromia in confetti. (b) Erythema multiforme-like eruption
Sensitization of medical, nursing, and pharmacy staff may be avoided by careful handling of the DCP solutions.
Oral fexofenadine, an H1-receptor antagonist, may partially inhibit the itch of contact dermatitis induced by DCP in patients with alopecia areata. Moreover, Inui et al. suggested that oral fexofenadine may enhance the efficacy of contact immunotherapy for extensive alopecia areata in patients with an atopic background (atopic alopecia areata).
Inosine pranobex or Isoprinosine is a combination of inosine, acetamidobenzoic acid, and dimethylaminoisopropanol used as an immunomodulating drug used to treat patients suffering from viral infections such as subacute sclerosing panencephalitis, varicella, and herpes simplex types 1 and 2 and as an adjunct in the treatment of genital warts. Its use for treatment of alopecia areata has been originally reported in the 1980s, with observation of patients with alopecia areata associated with autoantibodies showing response to therapy. Treatment with Isoprinosine produced a clinical response, as judged by total or partial hair growth in 9 of the 14 patients treated. It was striking to observe that seven of the nine responders had autoantibodies prior to treatment. These disappeared or decreased with Isoprinosine therapy. The authors concluded that alopecia areata is a heterogeneous condition subdivided by the presence or absence of autoantibodies, since clinical response to Isoprinosine was mainly obtained in patients with autoantibodies. Subsequent studies failed to confirm efficacy of Isoprinosine in inducing total remission in alopecia areata, either as a monotherapy or in combination with topical immunotherapy with DCP.
Also, treatment of therapy-resistant alopecia areata with fumaric acid esters has been proposed. Fumaric acid or trans-butenedioic acid is the chemical compound with the formula HO2CCH = CHCO2H. This white crystalline compound is one of two isomeric unsaturated dicarboxylic acids, the other being maleic acid. The salts and esters are known as fumarates. Fumarate is also an intermediate in the citric acid cycle used by cells to produce energy in the form of adenosine triphosphate from food. It is formed by the oxidation of succinate by the enzyme succinate dehydrogenase. Fumarate is then converted by the enzyme fumarase to malate. Human skin naturally produces fumaric acid when exposed to sunlight. Dimethylfumarate significantly reduces disability progression in multiple sclerosis and is used for treatment of psoriasis. In patients with relapsing–remitting multiple sclerosis, dimethylfumarate significantly reduced relapse and disability progression in a phase 3 trial. It activates the Nrf2 antioxidant response pathway, the primary cellular defense against the cytotoxic effects of oxidative stress. Fumaric acid ester therapy has also proved to be safe and effective in patients with psoriasis vulgaris. This treatment was introduced nearly 30 years ago but is only now gaining renewed interest among dermatologists. Although the mode of action of fumaric acid esters in the treatment of psoriasis is not fully understood, recent experimental data point toward a skewing of the Th1-dominated T-cell response in psoriasis to a Th2-like pattern and inhibition of proliferation of keratinocytes. More recently, therapy-resistant alopecia areata was treated with fumaric acid esters within the bounds of an open, non-placebo-controlled pilot study for a period of 6 months and a maximum dose of 120 mg dimethylfumarate per day. Six of ten patients took benefit from the therapy with fumaric acid esters, with very good results in three, presenting an almost entire remission. Validation of this therapeutic approach in a placebo-controlled and multicenter study with higher numbers of patients is pending.
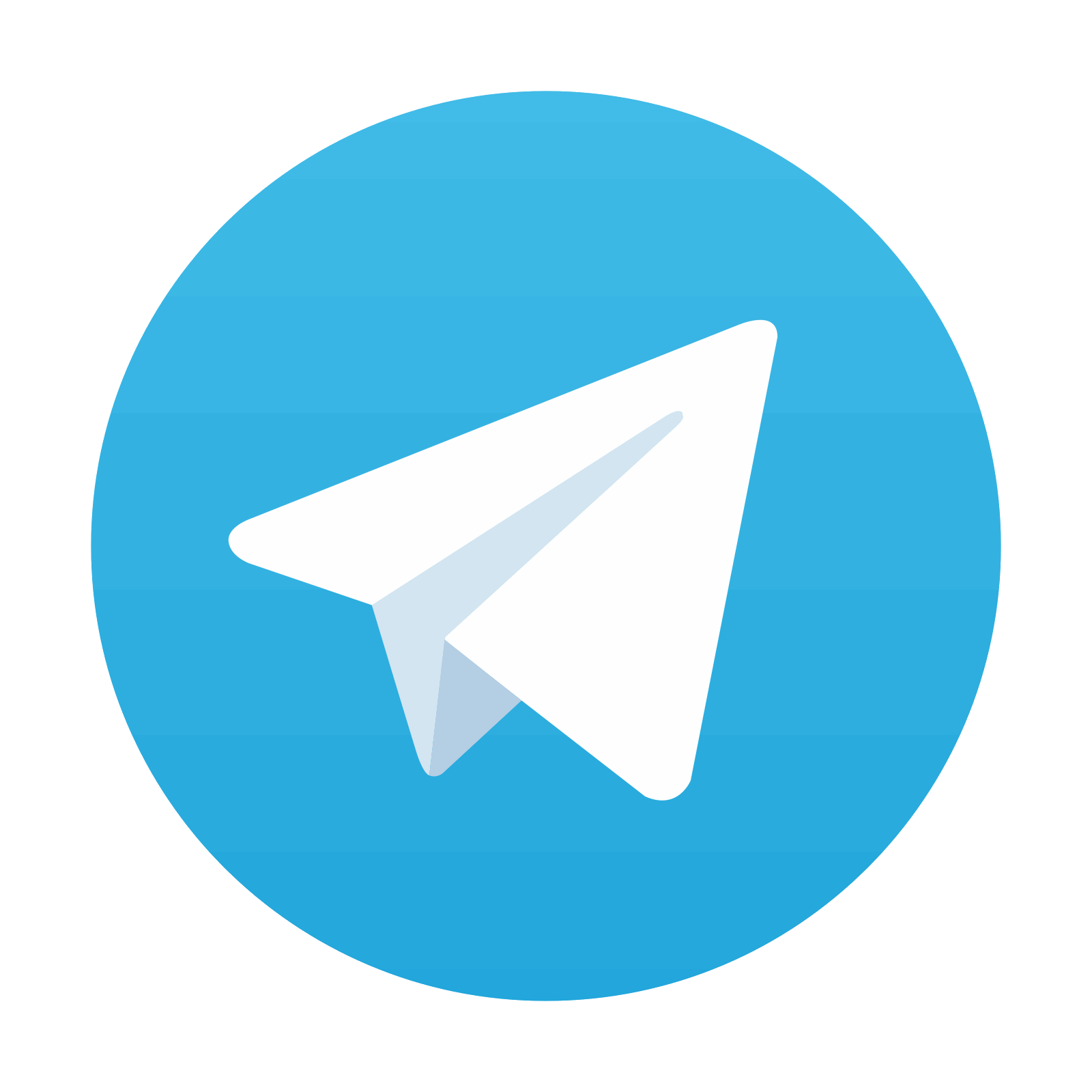
Stay updated, free articles. Join our Telegram channel

Full access? Get Clinical Tree
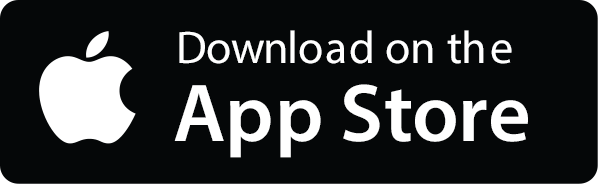
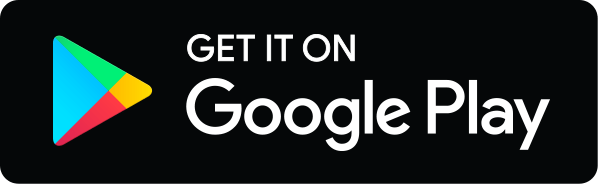