Fig. 5.1
Radiographic appearance of an anteriorly placed femoral tunnel. The tunnel should appear as posterior in the notch as possible
A tunnel that is placed too high in the notch, i.e., too near the 12 o’clock position (Fig. 5.2), leads to a graft with less obliquity in the coronal plane, commonly referred to as a vertical graft. The coronally vertical graft maintains sagittal plane stability, but offers less resistance to rotatory forces and can result in a knee that remains rotationally unstable after ACL reconstruction [9, 16]. In addition, a graft that is vertical in the coronal plane causes impingement against the posterior cruciate ligament and increases graft tension in flexion [17], which may lead to loss of flexion and/or graft stretching.
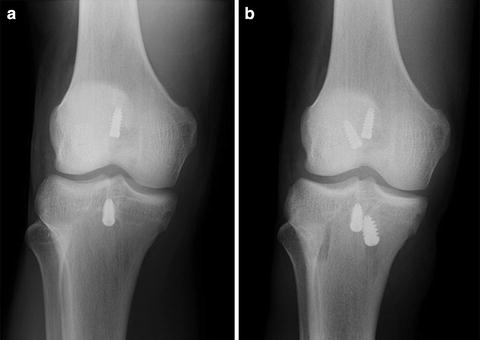
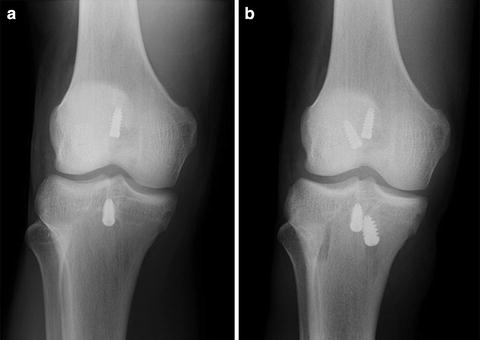
Fig. 5.2
(a) Radiographic appearance of a femoral tunnel placed near the 12 o’clock position in the notch, resulting in a vertical graft. (b) The subsequent failure was managed by placement of a new femoral tunnel near the 10:30 position, resulting in a more oblique graft
The femoral tunnel can also be malpositioned posteriorly, which may lead to blowout of the back wall of the tunnel, which can cause difficulty in obtaining adequate fixation or, if unrecognized, fixation failure altogether.
Tibial Tunnel
The ideal position of the tibial tunnel is in the middle of the native ACL footprint. Visualization of this landmark requires adequate debridement of the ACL remnants and determining the precise center of the footprint can be difficult, so placing the center of the tunnel 7 mm anterior to the PCL and just lateral to the medial tibial spine has been suggested [11]. Improper tibial tunnel placement has been implicated in 37 % of cases in which technical errors contributed to ACL graft failure [8]. The tibial tunnel can be malpositioned in any direction with different consequences for each.
Anterior placement of the tibial tunnel (Fig. 5.3) has been researched most extensively and leads to impingement of the graft on the intercondylar roof with the knee in extension. This impingement can lead to loss of complete knee extension or progressive elongation and subsequent failure of the graft [18]. On a lateral radiograph with the knee in full extension, any portion of the tibial tunnel that is anterior to an extrapolation of Blumensaat’s line should alert the surgeon to the possibility of intercondylar roof impingement. A tibial tunnel that is too medial can lead to graft impingement against the PCL, whereas too lateral a tunnel can lead to impingement on the medial aspect of the lateral femoral condyle. In either of these cases, repetitive impingement can lead to progressive graft elongation, loss of flexion, and eventual failure. Finally, a tibial tunnel that is malpositioned posteriorly will lead to a graft that has decreased obliquity in the sagittal plane and, as is seen with an anterior femoral tunnel, decreased effectiveness in resisting anterior tibial translation [9, 11]. A posteriorly placed tibial tunnel creates a graft that is excessively lax in flexion, as well.
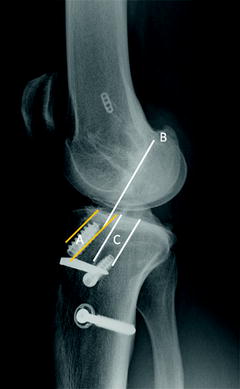
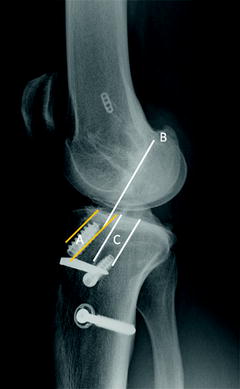
Fig. 5.3
Anteriorly malpositioned tibial tunnel (A), which is almost entirely anterior to Blumensaat’s line extended (B) with the knee in full extension. The subsequent failure was managed with appropriate tibial tunnel placement (C) posterior to Blumensaat’s line
Tunnel Preparation
Failure to adequately prepare the femoral or tibial tunnels may be an underappreciated technical cause of ACL graft failure. Tunnel drilling can leave sharp edges at the apertures that may impinge upon the graft after tensioning and fixation. At our institution, a shaver, angled arthroscopic rasp, or Gore-Tex smoother is routinely used to chamfer the tunnel apertures prior to graft passage.
Tunnel Enlargement
One technical cause of ACL graft failure that is specific to revision ACL reconstruction is failure to identify and manage tunnel enlargement, which can lead to both graft malposition and inadequate fixation [19]. It is important to critically evaluate preoperative radiographs for evidence of tunnel osteolysis (Fig. 5.4). A computed tomography (CT) scan should be obtained in cases where further detail is needed. Many techniques have been described to allow for revision ACL reconstruction in the setting of tunnel enlargement including using larger bone plugs, tying bone plug sutures around a screw and washer, using an endobutton, and impacting allograft bone struts into the enlarged tunnel along the bone plug to obtain a press fit, among others [20]. Cases in which the degree of tunnel enlargement will prevent appropriate placement and fixation of the ACL graft should be treated in a staged fashion, with initial tunnel debridement and bone grafting. After a period of 3–6 months, a repeat CT scan will typically confirm incorporation of the bone graft and the second stage of the revision reconstruction can be carried out. In the Multicenter ACL Revision Study (MARS) cohort, bone grafting of enlarged tunnels was performed at the time of the revision in 3 % of patients for the tibia and 3 % of patients for the femur. It was performed as a staged procedure before revision reconstruction in 9 % of patients for the tibia and in 8 % of patients for the femur [8].
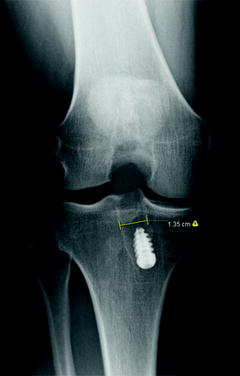
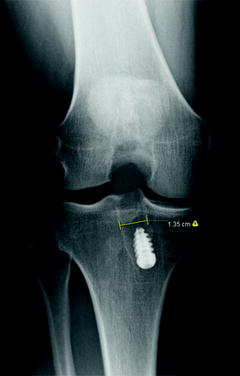
Fig. 5.4
Tibial tunnel enlargement in the setting of a failed primary ACL reconstruction
Graft Choice
The type of graft that is chosen for the primary ACL reconstruction can have a significant effect on the failure of the reconstruction. Studies attempting to compare allografts and autografts, both BTB and soft tissue, are numerous. Allograft reconstructions appear to undergo the same healing process as autografts, albeit at a much slower rate. At 6 months after surgery, allografts have decreased structural properties and slower incorporation [21], and animal models have shown that the center of the graft may heal incompletely [10]. These factors may lead to an increased risk of graft rupture.
Allografts need to be sterilized to prevent disease transmission and antigenic response in the host knee. Harvest, storage, sterilization, and processing techniques vary widely across tissue banks. Sterilization of grafts using ethylene oxide and gamma irradiation has been shown to cause increased clinical and mechanical failure, respectively [22]. Irradiated allografts have also been shown to develop laxity during follow-up at a higher rate than hamstring autografts, which may lead to increased failure rates [23]. These findings have led to a shift in practice that most allografts are fresh frozen specimens. However, a muted immune response still occurs to the donor tissue, which may cause tunnel enlargement [10], as well as changes in graft incorporation, revascularization, and remodeling [21].
Even after the initial healing period, allografts appear to fail at a greater rate than autograft counterparts in certain patients. In highly active patients under 50 years of age, BTB allografts have been shown to fail 2.6–4.2 times more frequently than in patients receiving BTB autografts and less active patients receiving allograft reconstructions [24]. Furthermore, allografts have been shown to fail more frequently in patients under 25 than BTB autografts [25]. Prospective, longitudinal, multicenter data also show allograft use to be an independent predictor of graft rupture [26]. Patients in the previously mentioned studies had undergone primary ACL reconstruction. It remains to be seen if these findings hold true for revision ACL reconstructions.
When considering the differences in failure rate between BTB autograft vs. multiple bundle hamstring autograft, data are mixed. A recent Cochrane review and a prospective cohort study with 10-year follow-up suggest that the failure rates between the two types of autografts are the same [27]. However, another recent systematic review found a twofold increase in graft failure after hamstring autograft reconstruction compared to BTB [22], and hamstring autografts have been shown to fail more frequently in patients under the age of 25 [25].
To avoid increased risk of ACL graft failure, we recommend the use of autograft ACL reconstruction in all patients under the age of 40 who wish to pursue an active lifestyle postoperatively. Furthermore, although the existing literature is inconclusive, it suggests that BTB autograft may have the lowest graft failure rate, especially in patients under 25 years of age.
Graft Fixation
The fixation of the graft is the weakest part of the ACL reconstruction in the first 8–12 weeks, until the graft is fully incorporated [28], and has been implicated in 7 % of cases in which technical errors contributed to graft failure [8]. The tibial fixation site is usually weaker than the femoral fixation site [29]. Failure of the fixation sites can be multifactorial and may include poor host bone quality, interference screw divergence, suture or knot failure, graft-tunnel mismatch, or improper fixation sizing. Interference screws have been shown to provide acceptable femoral and tibial fixation in both BTB and hamstring grafts [30], provided several characteristics of the screw are met.
Biomechanically, using 9 mm diameter interference screws for tibial-sided bone plug fixation results in higher pullout strength than 7 mm screws [31], while interference screws longer than 20 mm have been shown to provide no significant increase in strength of the construct [32]. The clinical significance of these findings is undetermined, although the senior author has had good results with routine use of 9 × 20 mm interference screws for bone plug fixation on both the femoral and tibial sides. It is important to note that screws should not exceed tunnel length, which may cause intra-articular abrasion and weakening of the graft.
Interference screw divergence can lead to inadequate graft fixation and subsequent failure. The divergence angle is rarely a problem on the tibial side as the insertion site is under direct visualization. However, at the femoral fixation site, there has been much written about interference screw divergence in both BTB and hamstring grafts. The technical difficulties associated with placement of the screw lead to divergence of the screw from the ideal axis, which is parallel to the tunnel. Some studies have suggested that in BTB grafts, divergence as low as 10° leads to increased pullout [33], others have shown that divergence resulted in increased pullout only starting at angles greater than 30° [34, 35]. Regardless, care should be taken to ensure that the interference screw is directed as parallel as possible to the tunnel without damaging the graft itself.
The use of titanium endobuttons for femoral graft fixation carries with it specific risks for graft failure. Ideally, the button should be deployed and confirmed with intra-operative fluoroscopy. The proper position for deployment is directly against the femoral cortex. If the button is deployed in the substance of the quadriceps (Fig. 5.5), it can cause underlying muscle necrosis and eventual graft slippage before the graft can fully incorporate. Alternatively, if the button is deployed in the femoral tunnel’s cancellous bone, there may initially be enough resistance to tension the graft intra-operatively. However, increased stress on the construct as the patient returns to activity may cause slippage through relatively soft cancellous bone and eventual graft failure [36].
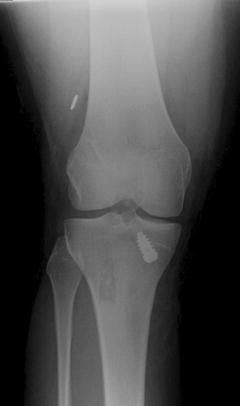
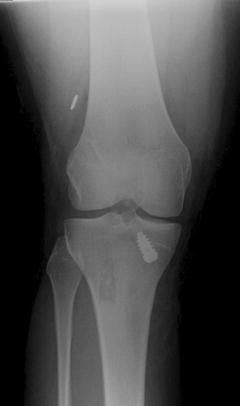
Fig. 5.5
Deployment of the femoral-sided endobutton within the substance of the quadriceps
Graft Tensioning
Proper graft tensioning remains a difficult element in ACL reconstruction and one that may be an under-recognized cause of graft failure. Undertensioning a graft dooms ACL reconstruction in the immediate postoperative period. Grafts do not contract over time, and the clinical result will be an unacceptable amount of residual laxity [9]. On the contrary, overtensioning a graft can lead to loss of joint motion, increased pressures on articular surfaces, premature arthritic changes, decreased graft strength, myxoid degeneration, and infrapatellar contracture syndrome [37–39]. Thus, it is imperative that the graft be appropriately tensioned at the time of surgery.
The optimal method of graft tensioning is widely debated. Many different recommendations have been made [10, 40, 41]. The inherent stiffness of BTB grafts is 3–4 times higher than hamstring grafts, and as a result, some have suggested tensioning BTB grafts with less tension than with hamstring grafts [10]. It should be noted that although there are “ideal” tensioning parameters, there is still high intra- and inter-surgeon variability in graft tensioning [42, 43]. The senior author recommends tensioning the graft with full-strength one-hand pull with the knee in full extension and has not encountered significant problems with graft failure, motion loss, infrapatellar contracture, or progressive arthritis using this technique. It is also prudent to cycle the knee through a normal range of motion 15–20 times under tension in order to eliminate stress laxity, as well as check for isometry [4, 9, 10].
Failure to Address Malalignment
Failure to address lower extremity malalignment, particularly genu varum deformity, can lead to increased stress on the reconstructed graft and contribute to graft failure (Fig. 5.6). Failure to address concomitant limb malalignment has been implicated as a cause of failure in 4 % of failed ACL reconstructions requiring revision [8]. Varus malalignment is classified as primary varus, double varus, or triple varus [44]. Distinction between these different groups is important in the ACL deficient knee, as it determines whether correction of alignment will improve the success of ACL reconstruction. In the primary varus knee, tibiofemoral geometry and possible medial meniscus damage or cartilage wear results in the weight bearing line (WBL) crossing through the medial compartment. In the double varus knee the WBL crosses further medial within the medial compartment and damage to the lateral ligamentous structures leads to separation of the lateral tibiofemoral joint during gait [44]. This opening of the lateral tibiofemoral compartment is seen as a varus thrust during early stance phase [45]. In the triple varus knee the addition of posterolateral ligamentous insufficiency causes increased external tibial rotation and hyperextension in addition to the lateral tibiofemoral compartment separation and a far medial WBL [45].
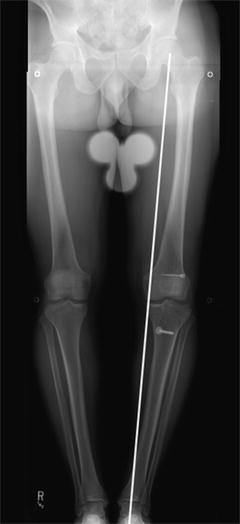
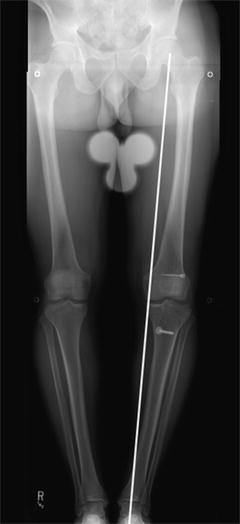
Fig. 5.6
Genu varum in the setting of failed primary ACL reconstruction, which should be treated with limb realignment prior to, or concurrent with, revision ACL reconstruction
Valgus high tibial osteotomy (HTO) is an accepted treatment option for patients with osseous varus alignment and medial compartment arthritis. The use of HTO has also been recommended in the double or triple varus ACL deficient knee in an order to protect the ACL graft. Multiple cadaver studies demonstrate increased force within the ACL with increasing varus alignment [46]. In order to protect the graft from excessive forces and potential failure, ACL reconstruction alone should not be performed in the varus knee in the setting of lateral joint opening or posterolateral ligamentous laxity. In the double varus knee, combined valgus HTO, either staged or concurrent, is recommended. In the triple varus knee, valgus HTO should be performed first followed by combined ACL and posterolateral corner (PLC) reconstruction if the patient has continued instability following the osteotomy [44]. In primary varus knees, valgus HTO has not been shown to enhance stability or protection of the graft from excessive forces and is not recommended in the absence of medial compartment arthrosis [47].
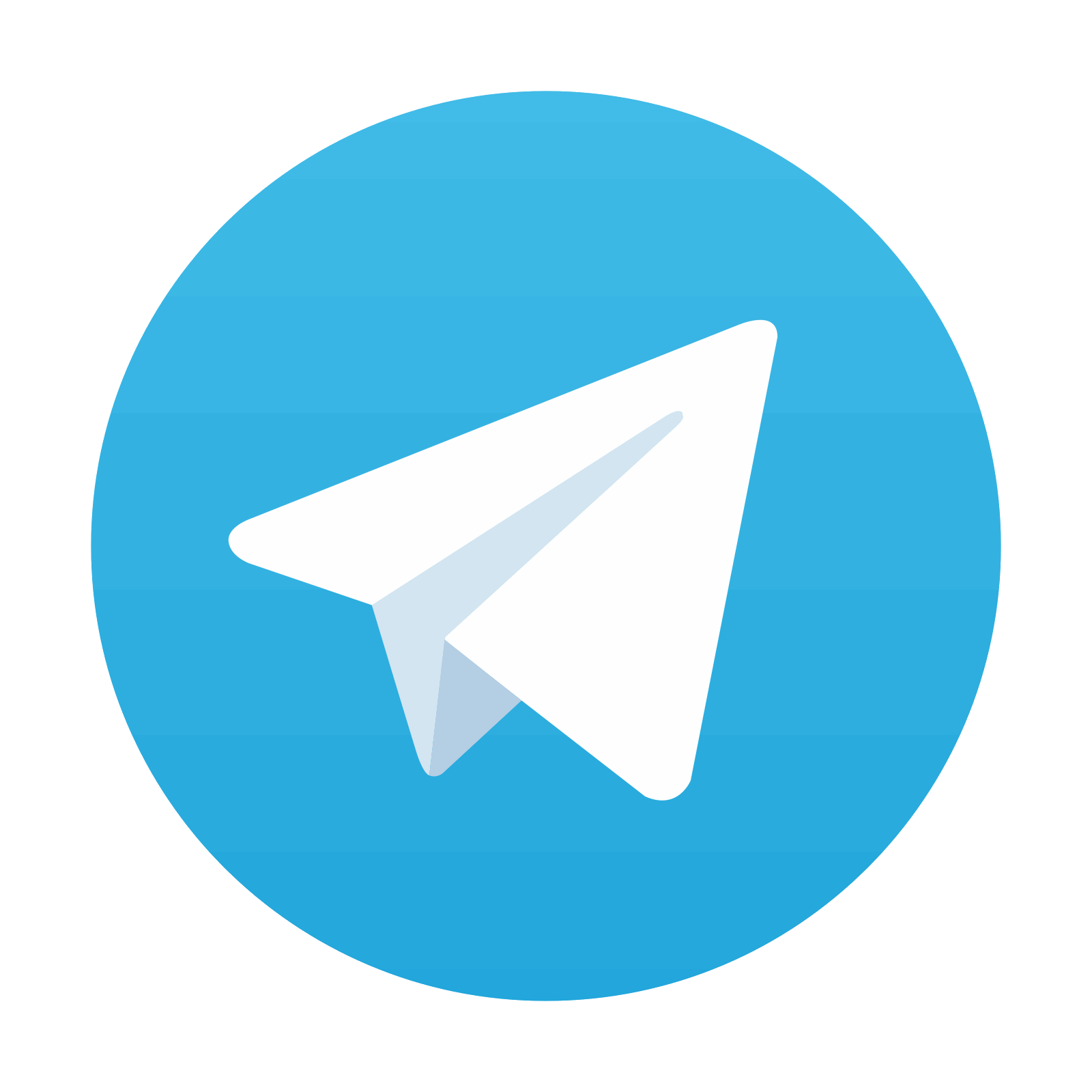
Stay updated, free articles. Join our Telegram channel

Full access? Get Clinical Tree
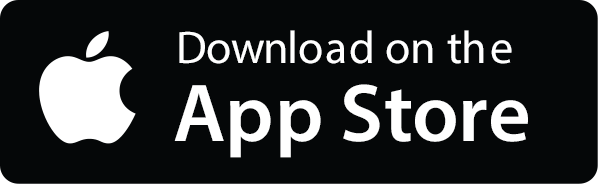
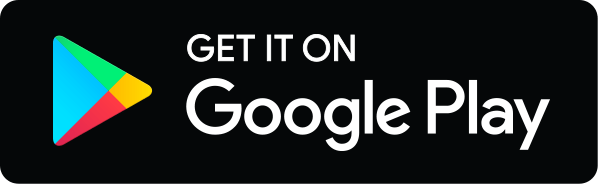