© Springer-Verlag Wien 2015
Hartmut Geiger, Heinrich Jasper and Maria Carolina Florian (eds.)Stem Cell Aging: Mechanisms, Consequences, Rejuvenation10.1007/978-3-7091-1232-8_11. Stem Cell Aging: An Overview
(1)
Buck Institute for Research on Aging, 8001 Redwood Boulevard, Novato, CA 94945-1400, USA
Abstract
Stem cell dysfunction is associated with age-related degenerative and proliferative diseases. Recent work in a range of model organisms has focused on characterizing the causes and consequences of stem cell aging. The insight obtained from these studies is likely to impact our ability to promote healthy aging and to develop new therapies against age-related diseases. This book provides an overview of such studies, aiming to present a comprehensive assessment of the current status of the field.
Loss of tissue homeostasis is a hallmark of aging, resulting in degenerative as well as proliferative diseases like cancer. A decline in stem cell (SC) function is a likely cause for these pathologies, and recent work in model organisms has focused on characterizing the causes and consequences of stem cell aging. Limiting these consequences of stem cell aging is likely to impact our ability to promote healthy aging and to develop new therapies against age-related diseases. Furthermore, the promise of regenerative medicine implies that mastering stem cell biology may open new avenues for truly rejuvenating therapies. For this promise to become reality, we need to understand not only the stem cell-intrinsic changes occurring as the organism ages but also the changes in local and systemic conditions that impact stem cell function and regenerative capacity in a variety of tissues. A wide range of studies in a variety of model systems have begun dissecting the mechanisms and consequences of stem cell aging, resulting in a coalescence of new concepts and models that promise to provide a comprehensive understanding of the decline of regenerative capacity in aging tissues (Jones and Rando 2011; Rando 2006; Sharpless and DePinho 2007).
This book provides an overview of such mechanisms, aiming to present a comprehensive assessment of the current status of the field. To achieve an accurate and exhaustive account of our understanding of stem cell aging, we have assembled a group of authors that study aging processes in a variety of model systems, including yeast, worms, and flies, as well as various mouse models of regenerative processes. The work of these authors thus represents a broad overview of stem cell aging ranging from cell-intrinsic mechanisms of replicative aging in yeast, over germline and somatic stem cell aging and niche-stem cell interactions in flies and worms to complex tissue/stem cell interactions in various vertebrate tissues, and strategies to improve regeneration and potentially design rejuvenating therapies in vertebrate models. We believe that this comprehensive approach is likely to achieve the most informative and timely overview of the field and allows identifying similarities and differences between individual stem cell systems. Here, I provide a broad overview of themes and insights that are elaborated on in more detail in the corresponding chapters.
1.1 SC-Intrinsic Changes in the Aging Organism: SC Dysfunction as Anticancer Mechanism
Age-related dysfunction of SCs is caused by both cell-intrinsic and cell-extrinsic mechanisms. While the germline stem cell lineage is in principle immortal, and should therefore have acquired mechanisms to prevent age-related decline, their proliferative activity is affected by changes in local and systemic support processes that decay in aging animals, leading to reproductive senescence. Somatic SCs, in turn, while being affected by systemic changes, also seem to age due to intrinsic mechanisms that limit their replicative lifespan. These include telomere dysfunction, DNA damage-induced cellular senescence, and age-related increases in the expression of cell cycle inhibitors (Gunes and Rudolph 2013; Jones and Rando 2011; Rando 2006; Sharpless and DePinho 2007). It has been proposed that these age-related changes in somatic stem cells are part of anticancer mechanisms that prevent deregulation of SC proliferation. The inability of somatic SCs to sustain efficient tissue regeneration in aging organisms would thus be a trade-off of processes required for cancer prevention (Campisi 2013; Sharpless and DePinho 2007). Supporting this view, recent studies confirm that deregulation of stem cell proliferation can contribute to tumor formation (Barker et al. 2009; Lapouge et al. 2011; White et al. 2011; Youssef et al. 2010). Furthermore, the identification of molecular similarities between cancer stem cells and tissue-specific stem cells highlights the importance of preventing deregulation of these cells to ensure long-term tissue homeostasis (Merlos-Suarez et al. 2011). This insight suggests that to promote tissue health and ultimately organismal lifespan, it is key to find strategies to modulate somatic SC function and increase their regenerative capacity without promoting excessive and deregulated proliferation.
1.2 Diverse Aging Processes in SCs
While stereotypical age-related processes that limit regeneration can thus be defined, somatic SCs exhibit a large proliferative diversity, reflecting the specific requirement of individual tissues. Specific modes of SC proliferation include (i) continuously cycling stem cells of high-turnover tissues, such as intestinal stem cells (Li and Clevers 2010; Simons and Clevers 2011; van der Flier and Clevers 2009) and short-term hematopoietic stem cells (HSCs) (Fuchs 2009); (ii) SCs whose proliferation is induced by injury, including airway basal epithelial stem cells and muscle satellite cells (Abou-Khalil and Brack 2010; Dhawan and Rando 2005; Rock et al. 2011); and (iii) stem cells with alternate quiescent and proliferative periods, such as hair follicle stem cells (Fuchs 2009). This diversity results in distinct age-associated deficiencies that contribute to regenerative decline of individual tissues and highlights the need to study aging of distinct SC systems separately to achieve a comprehensive understanding of the regenerative decline in aging organisms. Here, we take account of this diversity of aging processes in different stem cell populations by focusing on the age-associated dysfunction of individual stem cell populations separately in individual chapters.
1.3 SC Aging Through Evolutionarily Conserved Stress Responses
While cell-intrinsic age-related changes in somatic stem cells often specifically impact cell cycle regulation (and can thus be defined as anticancer “programs” that contribute to tissue aging), somatic SCs are also impacted by the random age-related accumulation of molecular damage, as well as by changes in local and systemic conditions in the aging organism. This includes inflammatory conditions and oxidative stress, as described in the hematopoietic system of the mouse and in the posterior midgut of flies (Biteau et al. 2011; Tothova and Gilliland 2007). The resulting accumulation of damage to DNA and other macromolecules is thus likely an important accelerator of SC aging (Biteau et al. 2011; Jones and Rando 2011; Rudolph et al. 2009; Tothova and Gilliland 2007). Mechanisms that allow cells to cope with these challenges and processes that contribute to the development of proliferative dysfunction in aging SCs are likely to be conserved from yeast to humans, and studies exploring the regulation of replicative lifespan in yeast are thus expected to provide important insight into somatic SC aging. One interesting example is the observation that budding yeast ensures rejuvenation of newly formed cells by asymmetrically segregating damaged and old macromolecules, providing a new “young copy” of such molecules to daughter cells (Aguilaniu et al. 2003; Shcheprova et al. 2008). Recent studies suggest that similar processes occur in human embryonic stem cells and in germline and somatic stem cells of flies (Bufalino et al. 2013; Fuentealba et al. 2008).
The complexity of somatic stem cell lineages in vertebrates often causes difficulties in definitively characterizing age-associated dysfunctions at the stem cell level in vivo. Characterization of stem cell aging in simpler model organisms in which lineage relationships are clearly defined, and which allow lineage tracing with relative ease to study stem cell proliferation and pluripotency, is thus necessary to provide a conceptual framework of stem cell aging and the loss of regenerative capacity. Work in flies and worms has provided such insight in recent years. This work initially focused on the effects of aging on germline stem cells (GSCs), and a large body of literature has examined mechanisms of stem cell aging in the worm and fly germline, as well as identified endocrine mechanisms by which GSCs influence metabolism and longevity of the animal (Arantes-Oliveira et al. 2002; Crawford et al. 2007; Drummond-Barbosa and Spradling 2001; Hsin and Kenyon 1999; Hsu et al. 2008; Jones and Rando 2011; LaFever and Drummond-Barbosa 2005; Mair et al. 2010; Pan et al. 2007; Pinkston et al. 2006; Toledano et al. 2012; Ueishi et al. 2009; Wang et al. 2008). These studies have established that in the aging animal, GSC maintenance is affected by a decline in specific niche factors, by metabolic imbalances, as well as by oxidative stress.
Adult somatic stem cells have been identified in the fly gonad, the intestine, and the Malpighian tubules (Decotto and Spradling 2005; Fox and Spradling 2009; Gonczy and DiNardo 1996; Margolis and Spradling 1995; Micchelli and Perrimon 2006; Ohlstein and Spradling 2006; Singh et al. 2007; Takashima et al. 2008). Among these stem cell populations, intestinal stem cells (ISCs) have served as a model to characterize processes and signaling mechanisms that regulate regenerative responses and whose deregulation contributes to the age-related decline in regenerative capacity (Biteau et al. 2011; Casali and Batlle 2009). These include signaling pathways required for homeostatic epithelial renewal (such as EGF and insulin signaling), stem cell maintenance (such as Wnt and Tor signaling), as well as stem cell stress responses (JNK, Jak/Stat, and Hippo signaling). Characterizing these signaling mechanisms is likely to not only provide insight into basic mechanisms of stem cell regulation but also elucidate the molecular etiology of tissue dysfunction, including age-related degeneration and cancer (Radtke and Clevers 2005; Rossi et al. 2008; Sharpless and DePinho 2007). It is important to note that the processes driving stem cell aging appear to be conserved from flies to mammals. FoxO transcription factors, for example, promote stem cell maintenance and function in both mice and flies (Biteau et al. 2010, 2011; Tothova and Gilliland 2007).
1.4 Oxidative Stress and Diet: Major Environmental Parameters Promoting Stem Cell Aging
1.4.1 Redox State and Stem Cell Function
The intracellular redox state has an important influence on stem cell function, and age-related changes in redox state or redox homeostasis have been implicated in age-related stem cell dysfunction of a wide range of stem cells. In mice, elevated ROS levels result in reduced regenerative potential and self-renewal in a wide range of stem cell populations, including neuronal and glial progenitors and HSCs (Diehn et al. 2009; Ito et al. 2004; Le Belle et al. 2011; Liu et al. 2009; Miyamoto et al. 2007; Smith et al. 2000; Tothova and Gilliland 2007; Tothova et al. 2007; Tsatmali et al. 2005). In flies, oxidative stress has been implicated in the age-associated loss of germline stem cells and in the age-associated deregulation of intestinal stem cell proliferation (Hochmuth et al. 2011; Pan et al. 2007). A low intracellular concentration of reactive oxygen species (ROS) is thus increasingly recognized as a critical condition for stemness, self-renewal, and pluripotency of stem cells.
Work in flies over the last decade has begun unraveling the signaling mechanisms mediating this effect. Increased ROS concentration primes hematopoietic progenitors of the larval lymph gland for differentiation (Owusu-Ansah and Banerjee 2009) and promotes ISC proliferation in the adult gut (Biteau et al. 2008; Buchon et al. 2009; Choi et al. 2008; Hochmuth et al. 2011). In the intestine, a Duox-mediated innate immune response in ECs produces ROS to control commensal and pathogenic bacteria, stimulating ISC proliferation (Biteau et al. 2008; Buchon et al. 2009; Choi et al. 2008; Ha et al. 2005; Hochmuth et al. 2011; Yang et al. 2009). The Drosophila homologue of the Nrf2 transcription factor, CncC, which regulates the expression of antioxidant enzymes, regulates ISC proliferation rates and limits ISC hyper-proliferation in aging flies (Hochmuth et al. 2011). It is likely that this mechanism is conserved in vertebrates, as mice deficient in Keap1, the negative regulator of Nrf2, show significant hyperkeratosis of the esophageal epithelium (Wakabayashi et al. 2003). Redox regulation by the Keap1/CncC regulatory module thus emerges as central to the control of SC proliferation and epithelial regeneration.
1.4.2 Metabolic/Dietary Effects on Stem Cell Function
Dietary conditions significantly influence lifespan, as exemplified by the robust lifespan extension observed in most tested organisms that are maintained on low-calorie food (dietary restriction, DR) (Jasper and Jones 2010; Kapahi et al. 2010; Katewa and Kapahi 2010). Somatic (as well as germline) stem cell function is strongly affected by nutritional conditions, suggesting that the longevity effects of DR are, at least in part, mediated by the enhanced regenerative capacity of a large number of tissues (Arantes-Oliveira et al. 2002; Mair et al. 2010). Many studies elucidating the interaction of dietary conditions, stem cell function, and lifespan have been performed in invertebrate model organisms, but recent work has also extended this inquiry into vertebrates.
In flies and worms, GSC proliferation is regulated by the nutrient-sensing insulin/IGF signaling (IIS) pathway (Drummond-Barbosa and Spradling 2001; Hsu et al. 2008; LaFever and Drummond-Barbosa 2005; Pinkston et al. 2006; Ueishi et al. 2009). IIS activity acts cell autonomously to maintain male GSCs (Drummond-Barbosa and Spradling 2001; Hsu et al. 2008; LaFever and Drummond-Barbosa 2005; McLeod et al. 2010; Ueishi et al. 2009), and DR conditions that extend lifespan delay the age-associated loss of GSCs (Mair et al. 2010). At the same time, poor diets can increase the rate of GSC loss during aging in females, where IIS activity also influences GSC maintenance in a non-autonomous manner by regulating the interaction between GSCs and the niche (Hsu and Drummond-Barbosa 2009). The Tor signaling pathway contributes to the regulation of GSC and somatic stem cell maintenance in the Drosophila ovary, as it promotes germline and somatic stem cell proliferation independently of insulin signaling, and needs to be suppressed by the tuberous sclerosis complex (TSC) to prevent premature differentiation of GSCs (LaFever et al. 2010; Sun et al. 2010; Voog and Jones 2010).
Dietary conditions also influence somatic SC populations, affecting their proliferative activity, lineage commitment, and maintenance. Not surprisingly, the IIS and Tor signaling pathways play important roles in influencing SC function in these conditions. FoxO transcription factors, which are activated in conditions of low IIS activity, promote quiescence, long-term maintenance, and regenerative capacity of various somatic SC populations in flies and mice: In the Drosophila midgut, insulin signaling and FoxO regulate proliferation of intestinal stem cells, influencing the proliferative response of ISCs to tissue damage and the extent of age-related intestinal dysplasia (Amcheslavsky et al. 2009; Biteau et al. 2010). Accordingly, moderate reduction of IIS activity in the ISC lineage promotes intestinal homeostasis and extends fly lifespan (Biteau et al. 2010). The role of FoxO in the control of SC quiescence is conserved in mammalian hematopoietic stem cells (HSCs) and neuronal progenitor cells (Renault et al. 2009; Tothova and Gilliland 2007; Tothova et al. 2007), where it affects SC proliferation, in part, by its ability to regulate antioxidant gene expression (Renault et al. 2009; Tothova and Gilliland 2007; Tothova et al. 2007). The Tor signaling pathway plays a critical role in promoting stem cell maintenance in the intestinal epithelium of flies, where its activity has to be suppressed by TSC proteins in SCs to prevent premature differentiation and loss of these cells (Amcheslavsky et al. 2011; Kapuria et al. 2012).
It can be anticipated that the role of reducing IIS/Tor signaling in the lifespan extension by DR is mediated, at least in part, by its beneficial consequences for somatic stem cell maintenance and proliferative control (Biteau et al. 2010; Kapuria et al. 2012). Whether DR can promote somatic stem cell function by influencing the activity of IIS in mammalian systems remains unclear. However, the decreased cancer incidence in dietary-restricted mice suggests that SC maintenance and quiescence are improved in DR (Kalaany and Sabatini 2009).
It is likely that other nutrient-sensing signaling pathways also influence stem cell function and regenerative capacity. This includes signaling through the Sir2/SIRT1 family of NAD+-dependent deacetylases (Firestein et al. 2008; Guarente and Picard 2005).
Understanding the signaling mechanisms translating nutritional status to stem cell state and regenerative capabilities is likely to not only provide new insight into avenues to improve stem cell maintenance in aging animals but also to provide new therapies for proliferative diseases associated with metabolic dysfunction.
1.5 Age-Related Niche Dysfunction
A central cause of stem cell and regenerative dysfunction in aging organisms appears to be the loss of support from the local microenvironment, the “niche” (Jones and Rando 2011). Age-related niche dysfunction has been characterized primarily in the male and female germline of Drosophila, but studies in mice support the notion that similar processes are conserved in vertebrates. The interaction between GSCs and their niche is disrupted in aging females due to increased oxidative stress (Pan et al. 2007), while a decline in trophic support from the niche drives GSC decline in males (Jones and Rando 2011; Toledano et al. 2012). Interestingly, this decline is caused by an increase in the expression of the microRNA let-7, which negatively regulates the RNA-binding protein Imp (IGFII mRNA-binding protein), which in turn is required to stabilize the mRNA of the IL-6 like ligand Upd in the niche. Highlighting the potential conservation of processes driving age-related stem cell dysfunction, the let-7 microRNA has been implicated in stem cell self-renewal and differentiation in vertebrates, and deregulation of IL-6 expression by inhibition of let-7 promotes cell transformation into cancer stem cells in a breast cancer paradigm (Iliopoulos et al. 2009; Melton et al. 2010).
The influence of the local and systemic environment on somatic SC function has been explored extensively in mice. Studies using heterochronic parabiosis and assessing the regenerative capacity of satellite cells (muscle stem cells) suggest that changes in the exposure to growth and differentiation factors, including Wnt ligands, play a critical role in age-related stem cell dysfunction (Brack et al. 2007, 2008; Conboy et al. 2005; Conboy and Rando 2005; Jones and Rando 2011; Wagers and Conboy 2005).
A recent study further shows the importance of niche-derived factors for long-term maintenance of intestinal stem cells in mice and for the effects of nutritional status on stem cell maintenance: Paneth cells express the ligands Wnt3, Wnt11, and EGF to promote stem cell function in vitro and in vivo and can be considered “niche” cells for these SCs (Sato et al. 2011). Nutrient-responsive Tor signaling in Paneth cells influences their ability to promote SC maintenance, most likely by regulating the expression of bone stromal antigen 1 (Bst1), an ectoenzyme that produces the paracrine factor cyclic ADP ribose (Yilmaz et al. 2012).
1.6 SCs and Lifespan
While it is clear that improved regenerative capacity of stem cells promotes tissue health, the effects of stem cell function on overall lifespan of the organism have only recently begun to be understood. Studies in worms and flies have uncovered significant effects of germline stem cells on lifespan, primarily through endocrine effects on metabolism. But also somatic stem cell function in the fly intestine has recently been shown to affect lifespan, primarily by influencing the integrity of this critical tissue (Biteau et al. 2010; Rera et al. 2011, 2012). Strikingly, these studies revealed that stem cell activity has to be maintained within a critical window to improve tissue function and extend lifespan, highlighting the delicate balance between maintaining regenerative capacity and promoting dysplasia or cancer when stem cell proliferation is enhanced. Interestingly, IIS activity influences ISC function, suggesting that the lifespan effects of modulating IIS activity are mediated, at least in part, by its effects on ISC maintenance and activity. Similar effects may be conserved in vertebrates: Similar to flies, where dysplasia in the intestine is triggered by hyper-activation of IIS (Biteau et al. 2010), loss of FoxO negatively impacts NSC and HSC behavior in mammals (Renault et al. 2009; Tothova and Gilliland 2007; Tothova et al. 2007). It is likely that an optimal level of IIS is achieved during DR and contributes to SC maintenance (Mair et al. 2010).
The relationship between stem cell deregulation and tumor formation suggests that modulating somatic stem cell function in vertebrates should have significant effects on lifespan (Radtke and Clevers 2005). In the intestinal epithelium of mice, for example, intestinal stem cell proliferation and intestinal cancer have been linked: stress signaling can induce cell proliferation in the intestinal crypt and increases tumor incidence and growth in an inflammation-induced colon cancer model (Sancho et al. 2009). The precise role of stem cell-specific stress signaling in the development of tumors remains unclear, however, and the detailed analysis of stem cell activity in the Drosophila
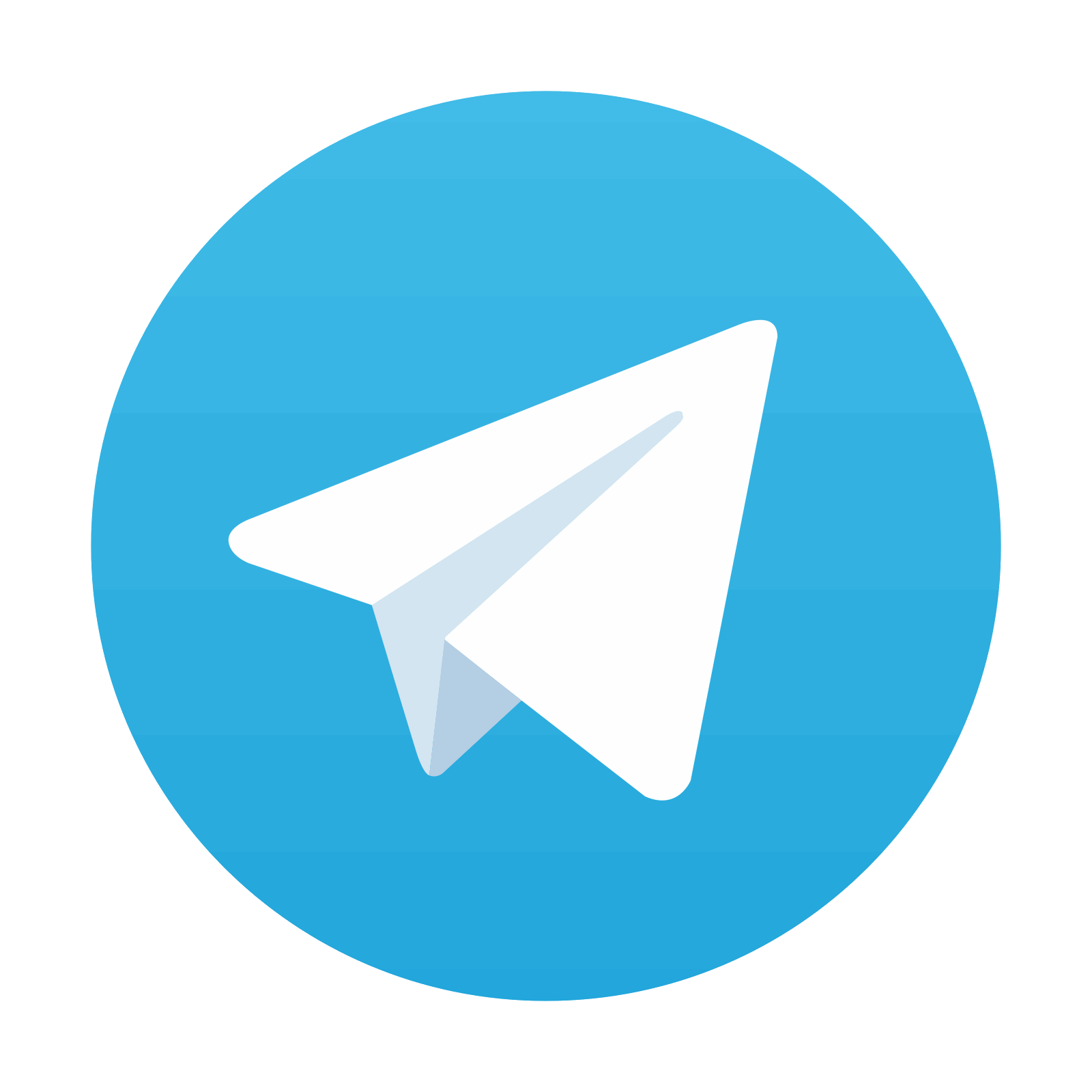
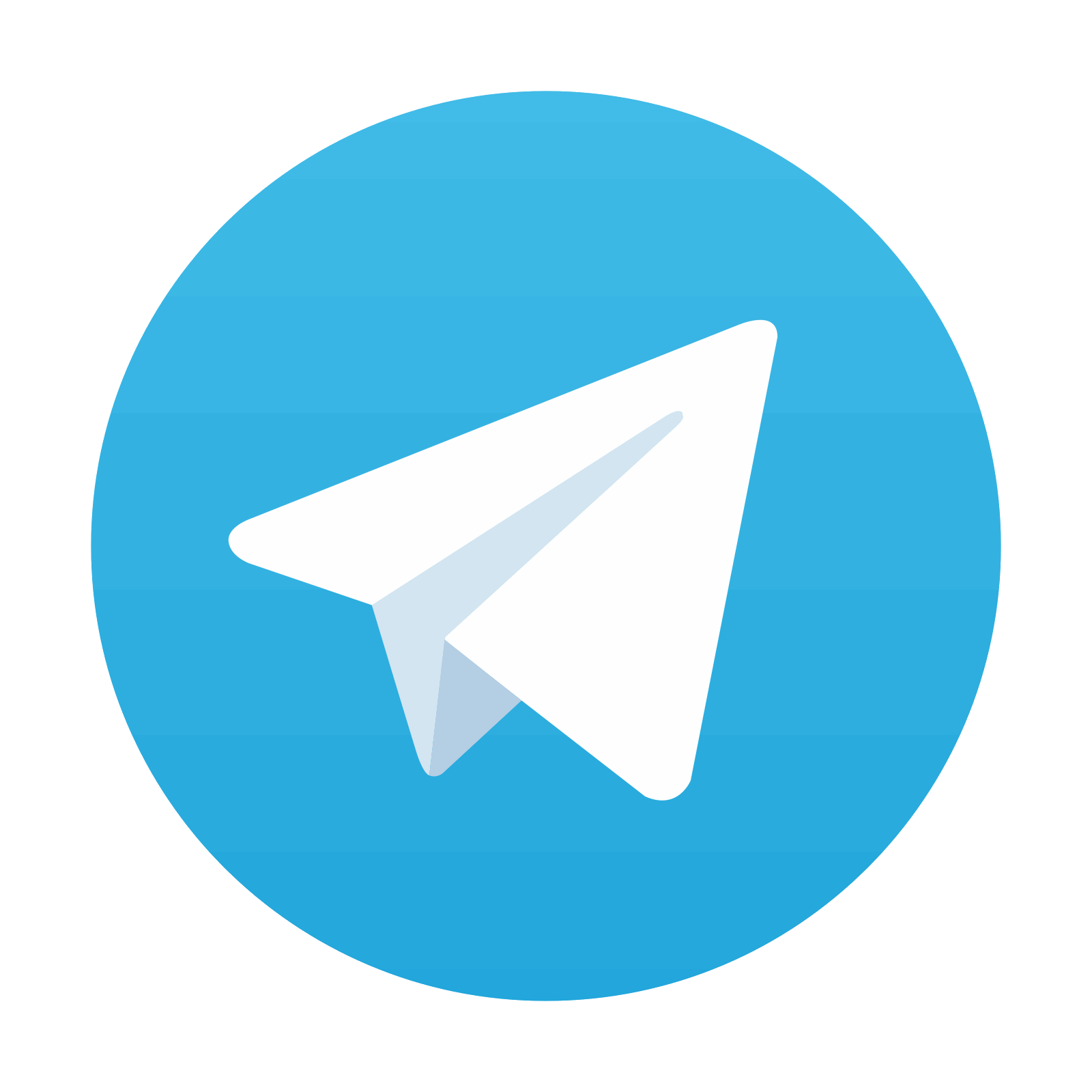
Stay updated, free articles. Join our Telegram channel

Full access? Get Clinical Tree
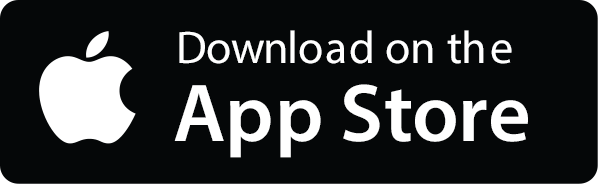
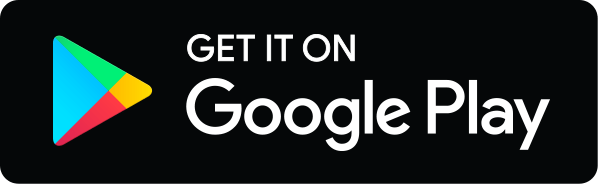