6 Static and Dynamic Characterization The spine can succumb to degenerative changes due to skeletal aging or a genetic predisposition. In time, the direct vascular supply to the vertebrae and disks decreases, and both undergo the accumulated effects of axial loading. The rate at which skeletal degenerative changes occur differs on an individual basis, depending in part upon the forces absorbed by the spine throughout an individual’s life. The resulting decreases in water and oxygen content, and metabolic efficiency lead to a disk that is more compressed, less elastic, and more prone to tear and rupture. The polysaccharide gel in the nucleus may deteriorate and lead to redistribution of pressure loads. This redistribution may stress the annulus, leading to disk material protrusion or herniation. As the degeneration progresses, the collagen in the disk is altered and the stress-bearing ability of the disk is reduced along with the disk space height.1 As a result, the biomechanical burden is shifted to the adjacent vertebral structures. At each vertebral level, there are three distinct motion segments—two at the facet joint complex and one at the disk space. Changes in any part of this motion segment are ultimately compensated by the adjoining parts of the functional spine unit. Ironically, degenerative changes in biological tissues are usually the body’s attempt to heal. If damage exceeds the local healing process, a hypermobile spinal segment could lead to further instability. The body’s response to instability would be to further immobilize the motion segments of the vertebral elements. This results in osteophyte or spur formation along the ligaments, joint capsule, and peridiskal area. This cascade of degenerative changes was first described by Kirkaldy-Willis and Yong-Hing and can be subdivided into three stages: dysfunction, instability, and restabilization.2 The stage of dysfunction may involve one or more of the following changes: outer annular tears and separation of the end plate, synovial reaction, and cartilage destruction. The clinical and radiographic findings are minimal or absent. The instability stage involves disk resorption and loss of disk space height. Further, capsular laxity in the facets may develop, leading to subluxation. Clinical and radiographic findings are more apparent. In the restabilization stage, the severe degenerative changes lead to osteophyte formation and stenosis. As a result of the body’s reactive changes, areas around the degenerated motion segment of the vertebral column enlarge with new reactive tissue and bony formation. Unfortunately, these reactive changes, such as formation of osteophytes, and ligamentous and facet hypertrophy, can sometimes lead to neural compression requiring surgical intervention. Furthermore, multiple levels of disk degeneration may lead to the reversal of normal cervical curvature. All of these changes can result in symptoms of pain and weakness, and, ultimately, debilitation. Degenerative disk disease thus describes a normal process of aging, and thus the term disease is perhaps a misnomer. Typically, it represents a natural aging process, rather than a true disease state, which nevertheless can be a painful and debilitating process. Pain arising from this process may be divided into neurocompressive or mechanical on the basis of the anatomical pain generators. The important step in the initial evaluation of patients with neck pain is determining the pain generator and the presence of radiculopathy, myelopathy, or both. Total lumbar disk replacements have been used in Europe since the 1980s, with reported clinical success similar to interbody fusions despite the lack of long-term prospective randomized clinical trials.3,4 The SB Charité (DePuy Spine, Raynham, MA, a Johnson & Johnson Company) is currently the most widely implanted total disk replacement. In 2005, the SB Charité was approved for commercialization by the U.S. Food and Drug Administration (FDA). In addition, there are other prospective randomized studies ongoing in the United States for lumbar artificial disks. Enthusiasm for cervical arthroplasty came about in the late 1990s. The first human trial involving a cervical prosthesis was reported in 1998.5 The Prestige (Medtronic Sofamor Danek, Memphis, TN), formerly known as the Bristol disk, is a two-piece stainless steel metal-on-metal design. A British trial comparing the Bristol disk versus autologous graft fusion demonstrated increase in adjacent-level movement in the fusion group as compared with the Bristol disk group at 1-year follow-up.6 Medtronic’s Bryan Cervical Disc, another investigational device, consists of a polyurethane nucleus that is situated between two end plates. As with the lumbar artificial disks, there are multiple, prospective, randomized cervical disk trials ongoing in the United States. The Spinal Kinetics Cervical Disc (Spinal Kinetics, Inc., Redwood City, CA) is a novel artificial disk that is intuitively designed to replicate the natural disk (Fig. 6–1
Spinal Kinetics Cervical Disc
Clinical Use of Artificial Disks
Design of the Spinal Kinetics Cervical Disc
Clinical Use of Artificial Disks
Design of the Spinal Kinetics Cervical Disc
Stay updated, free articles. Join our Telegram channel

Full access? Get Clinical Tree
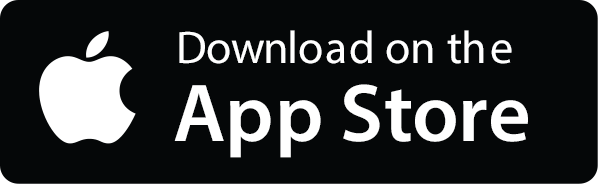
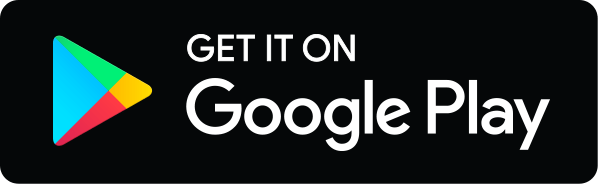