Fig. 1.1
A diagrammatic representation of the structure of human skin in cross section. The epidermis is composed of the stratum corneum and the viable epidermis. Diagram is not to scale
1.2.1.1 Stratum Corneum: The Primary Barrier
The stratum corneum is the outermost layer of the skin. It is typically 10–20 μm thick and composed of 10–15 layers of corneocytes (Agache 2004a; Williams 2003). Corneocytes are nonliving cells derived from terminally differentiated keratinocytes that have originated from the deeper layers of the epidermis. Morphologically, corneocytes are flattened and elongated, measuring about 0.2 μm thick and 40–60 μm wide (Kashibuchi et al. 2002). Corneocytes have a cornified envelope in place of a plasma membrane, which is surrounded by a lipid coat. They lack nuclei and cytoplasmic organelles but are filled with keratin filaments and are interspersed in a lipid-enriched extracellular matrix that also contains protein/peptide components (Elias 2012). This organisation of the stratum corneum is commonly referred to as the ‘brick and mortar’ model (Elias 1983; Michaels et al. 1975), where the corneocytes are likened to bricks and the extracellular matrix analogous to the mortar in a brick wall (Fig. 1.3). Corneocytes are connected by corneodesmosomes and are continuously shed from the skin surface via desquamation.
Since diffusion across the stratum corneum is considered as the major pathway of skin permeation (see Sect. 1.3), the structural properties of this layer have been extensively studied to elucidate its barrier function. Significant effort has been focused on elucidating the role of stratum corneum lipids, since the lipid-enriched extracellular matrix is the only continuous domain in the stratum corneum and thus likely to be pivotal to its barrier function (Bouwstra et al. 2002). This is supported by empirical data that demonstrate skin penetration predominantly through the extracellular matrix (Labouta et al. 2011), the marked reduction in the skin’s barrier function following stratum corneum lipid extraction (Sweeney and Downing 1970), and that chemicals that disrupt stratum corneum lipid organisation enhance skin permeation (Williams and Barry 2004).
Through freeze-fracture electron microscopy, Breathnach et al. (1973) first observed that the extracellular space in the stratum corneum was composed of a ‘laminated material’. It is now understood that stratum corneum lipids are organised into layers (lamellae) that run parallel to the flat plane of the corneocytes. According to the widely accepted Landmann model of skin barrier lipid morphogenesis (Landmann 1986), stratum corneum lipids are initially secreted by lamellar bodies found in keratinocytes and later fuse to form continuous lipid lamellae in the extracellular space. This model is supported by evidence of lipid membrane extrusion and fusion in the stratum corneum under electron microscopy (Madison et al. 1987). An alternative model, the membrane folding model, postulates that the extracellular lipid lamellae forms from single, coherent lipid structures through a non-fusogenic process (Norlén 2001a).
Ceramides, cholesterol and free fatty acids are the main constituents of the extracellular matrix, present in approximately equimolar proportions (McGrath et al. 2004). Of these, ceramides account for approximately 50 % of stratum corneum lipids by mass (Law et al. 1995). Eleven classes of ceramides, encompassing 342 individual ceramide species, have been identified in the human stratum corneum (Masukawa et al. 2008). Each ceramide molecule consists of a sphingoid moiety (sphingosine, phytosphingosine, 6-hydroxysphingosine or dihydrosphingosine) containing a polar head group and a hydrocarbon chain and another hydrocarbon chain derived from a fatty acid or fatty acid ester moiety (Fig. 1.2). The polar head groups of ceramides can form lateral hydrogen bonds when organised in lattice structures within lipid bilayers. The hydrocarbon chains are mostly saturated, with few exceptions, and exhibit chain length distribution (Bouwstra and Gooris 2010; Masukawa et al. 2008). Notably, those ceramides containing a fatty acid ester moiety have an exceptionally long hydrocarbon chain. To illustrate this, the typical ceramide contains a total of 38–54 carbon atoms, whereas fatty acid ester-containing ceramides contain a markedly larger number (66–72) of carbon atoms (Masukawa et al. 2008). Like ceramides, the free fatty acids found in the human stratum corneum are mostly saturated. Cholesterol fluidises the stratum corneum lipid bilayers at skin temperature (Zbytovská et al. 2008). There also exists cholesterol sulphate, typically at 2–5 % weight ratio of total stratum corneum lipids, which appears to facilitate the formation of the lipid lamellae and stabilises the stratum corneum by inhibiting enzymatic degradation of corneodesmosomes (Sato et al. 1998).


Fig. 1.2
Chemical structures of two stratum corneum ceramides and their possible conformations. Ceramide 2 (CER2) is shown as a typical ceramide consisting of a polar head group (dotted circle) and two hydrocarbon chains derived from a fatty acid and a sphingoid moiety, respectively. In CER2, the hydrocarbon chain derived from the fatty acid is 24 carbon atoms long, exemplifying the typical hydrocarbon chain length for this portion of ceramide molecules. Ceramide 1 (CER1) is shown to illustrate its exceptionally long fatty acid ester-derived hydrocarbon chain compared to the typical ceramide molecule (CER2). The inset shows two possible molecular conformations of ceramides, the ‘hairpin’ or ‘tuning fork’ conformation, where hydrocarbon chains point in the same direction, and the extended or ‘splayed chain’ conformation, where the hydrocarbon chains point in opposite directions
The precise molecular arrangement of stratum corneum lipids within the extracellular matrix remains a subject of intense investigation. Under electron microscopy with ruthenium tetroxide fixation, the extracellular lipid matrix displays characteristic, alternating ‘broad-narrow-broad’ lucent bands (Madison et al. 1987; Swartzendruber et al. 1989). This trilamellar motif, with a repeat distance (periodicity) of approximately 13 nm, is known as the long periodicity phase (LPP). This is in contrast with the short periodicity phase (SPP), which has a periodicity of approximately 6 nm but has not been observed in some animal species. For this reason, the LPP is considered to be more important for the skin’s barrier function and thus has been the focus of most investigations into stratum corneum lipid organisation. X-ray diffraction analysis has revealed that the extracellular lipids are packed into hexagonal or orthorhombic lattices along the plane parallel with the lamellae, orthorhombic packing being denser than hexagonal. Based on these and other supporting observations, a number of molecular models have been proposed to elucidate stratum corneum lipid organisation:
The model proposed by Swartzendruber et al. (1989) describes a repeat unit (Landmann unit) of the extracellular lipid lamellae comprising two lipid bilayers. Ceramides in each apposing bilayer assume the ‘splayed-chain’ conformation (Fig. 1.2) and, in so doing, contribute hydrocarbon chains to form a lipid monolayer between the bilayers that holds the two bilayers together.
The domain mosaic model (Forslind 1994) describes an intracellular lipid matrix where polar lipids are segregated in crystalline domains surrounded by liquid crystalline ‘grain borders’. It is postulated that the liquid crystalline ‘grain borders’ provide a diffusion pathway for hydrophobic molecules to penetrate the skin.
The ‘sandwich’ model (Bouwstra et al. 2000, 2001, 2002) describes alternating crystalline and liquid phases within the LPP. The repeating unit in this model comprises three lipid layers, namely, a middle, narrow liquid phase sandwiched between two adjacent, broad crystalline phases. Ceramides are packed into a crystalline lattice within the broader phases, but the long linoleate moieties in ceramide 1 and ceramide 4 protrude beyond the thickness of the crystalline phases into the space between the crystalline phases to form the narrow liquid phase with cholesterol. It is postulated that the liquid phase represents the main permeation pathway within the LPP. In this model, ceramides are typically depicted in the ‘hairpin’ (or ‘tuning fork’) conformation (Fig. 1.2).
The single gel phase model (Norlén 2001b) describes the extracellular lipid matrix as a single and coherent lamellar gel phase, with no phase separation.
The model proposed by Hill and Wertz (2003) describes three lipid layers of equal thickness within the LPP. According to this model, the broad-narrow-broad motif observed under electron microscopy with ruthenium tetroxide fixation is an artefact. It is suggested that unsaturated linoleate or sphingosine moieties are asymmetrically distributed in the central lamellae but not the outer lamellae, resulting in the reduction of a greater amount of the fixative agent and hence an apparently narrower central band.
The model presented by McIntosh (2003) has lamellae composed of twin lipid bilayers, with an asymmetric distribution of cholesterol and ceramide 1 in apposing monolayers of each lipid bilayer.
The model of Schröter et al. (2009) suggested that the SPP is formed from lipid bilayers composed of short-chain ceramides, with the long-chain ceramide 1 spanning multiple lipid bilayers. Cholesterol is distributed homogenously within the lipid bilayers.
More recent work has shown that ceramides are fully extended in the stratum corneum, with the two hydrocarbon tails of each ceramide molecule pointing in opposite directions centred on the polar head of the sphingoid moiety (Iwai et al. 2012).
1.2.1.2 Viable Epidermis
Excluding the stratum corneum, the rest of the epidermis is composed of nucleated cells and therefore collectively referred to as the viable epidermis. The viable epidermis is typically 50–100 μm thick (Gentilhomme and Neveux 2004) and devoid of blood capillaries and sensory nerve endings. It is composed primarily of keratinocytes (95 %), with the remainder being Langerhans cells, melanocytes and Merkel cells. Keratinocytes arise from the stratum basale and undergo progressive differentiation whilst migrating towards the stratum corneum. Keratinocyte differentiation is characterised by increasing keratinisation (formation of intracellular networks of keratin fibres), the formation of the lamellar bodies that secrete stratum corneum lipids and the loss of intracellular organelles and nuclei. The process culminates in the formation of corneocytes in the stratum corneum. Keratinocyte differentiation serves to maintain the stratum corneum by replenishing stratum corneum lipids and corneocytes lost via desquamation.
1.2.1.3 Dermis
The dermis, typically ≥1 mm thick (Agache 2004b; Williams 2003), comprises the bulk of the skin and is responsible for its elasticity and strength. It is composed principally of fibroblasts in an extracellular matrix of structural proteins, mainly collagen and elastin. It also contains a range of immune cells including macrophages and dermal dendritic cells. The dermis can be subdivided into the upper papillary dermis and the lower reticular dermis, which can be distinguished microscopically from each other by the thinner and looser packing of collagen fibres in the papillary dermis. The papillary dermis contains papillae that interdigitate with the basal layer of the epidermis at the dermo-epidermal junction. The dermis contains hair follicles, sweat glands, sebaceous glands, sensory nerve endings, lymphatic vessels and blood capillaries which extend to the dermal side of the dermo-epidermal junction. This allows nutrient and oxygen delivery to, as well as waste removal from, the avascular epidermis to occur by diffusion across the dermo-epidermal junction.
1.2.1.4 Hypodermis
The hypodermis is the innermost layer of the skin. However, its absence is notable in some lean skin, such as that on the eyelid. The hypodermis is composed mainly of subcutaneous fat. Embedded in this skin layer are larger lymphatic and blood vessels.
1.2.2 Functions of the Skin
The primary function of the skin is to separate the internal physiological environment of the body from the external non-physiological environment. To put it plainly, it serves to ‘keep the insides in, and the outsides out’ (Williams 2003). The skin barrier is physical, chemical and immunological in nature. The physical barrier is provided primarily by the stratum corneum, that is to say, traversing the stratum corneum is usually the rate-limiting step in substance exchange between the body and the environment via the skin. This physical barrier is responsible for regulating not only the ingress of exogenous materials but also preventing excessive water loss from the body. The chemical barrier is known as the ‘acid mantle’. The skin owes its chemical barrier function to the acidic (pH 4–6) nature of the skin surface which protects the body in two ways. Firstly, it confers selective antimicrobial properties to the skin by maintaining the natural skin microflora, which live optimally in an acidic environment, whilst arresting the growth of pathogenic microorganisms which thrive in alkaline environments. Secondly, it helps maintain the integrity of the stratum corneum barrier since many skin enzymes pivotal to stratum corneum lipid homeostasis (e.g. β-glucocerebrosidase and sphingomyelinase) have a pH optima within this pH range (Bowser and Gray 1978; Takagi et al. 1999). Sebaceous glands in the skin, which secrete sebum, perform a similar function. Following its secretion to the skin surface, sebum forms a greasy film on the skin, which waterproofs the skin to maintain hydration and suppleness. Sebum also contains antimicrobial constituents.
The skin is also an immune-competent organ. A range of immune cells including Langerhans cells, dermal dendritic cells and macrophages are found in the skin (Zaba et al. 2008). These cells conduct immune surveillance and defend the body against invading microorganisms. They are antigen-presenting cells capable of priming naïve T lymphocytes to elicit a primary immune response against newly encountered antigens. This is an important role of the skin considering a compromised skin barrier is a common route of pathogen entry into the body. There is also an increasing body of evidence that supports a role for some skin dendritic cell subsets in inducing immune tolerance (Romani et al. 2012), which is equally important for maintaining immune homeostasis.
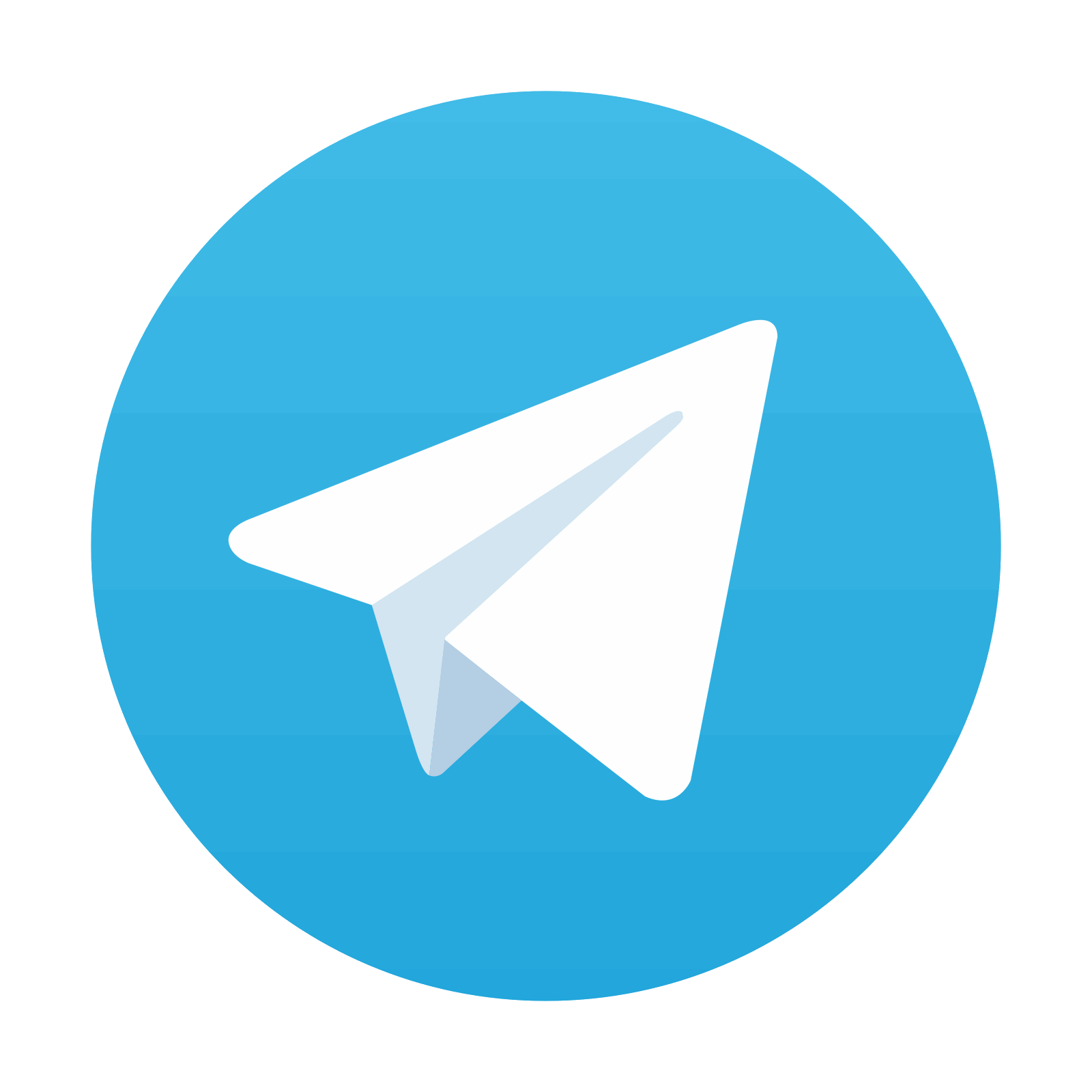
Stay updated, free articles. Join our Telegram channel

Full access? Get Clinical Tree
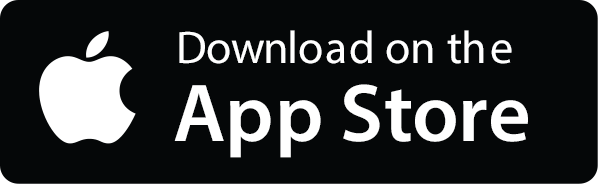
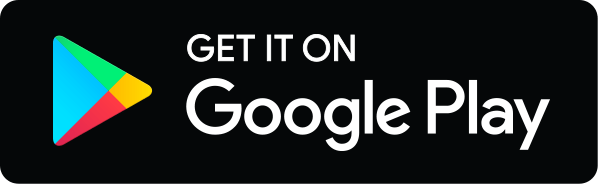