In addition to the free ceramides, it was recognized that ω-hydroxyceramides become covalently attached to the outer surface of the cornified envelope (Wertz and Downing 1986, 1987; Swartzendruber et al. 1987). In addition to ceramide OS, the covalently bound lipid in porcine stratum corneum generally included small proportions of ω-hydroxyacid and normal fatty acid.
In humans, there are additionally covalently bound ceramides OP and OH (Wertz et al. 1989; Robson et al.1994; Hill et al. 2006). The ω-hydroxyacids and ω-hydroxyceramides are ester-linked to acidic groups on the outer surface of the cornified envelope through the ω-hydroxyl group (Stewart and Downing 2001).
Biosynthetic Pathways
The rate limiting enzyme in sphingolipids biosynthesis is serine palmitoyl transferase (Radin 1984). This enzyme condenses serine with palmitoyl CoA in an NADPH-dependent reaction with loss of a CO2 and formation of 3-ketodihydrosphingosine. An NADPH-requiring reductase then reduces the ketone to produce dihydrosphingosine, and N-acylation then produces a simple ceramide. Various modifications of the base component of this initial ceramide include hydroxylations on carbon-4 of the base component to produce phytosphingosine, introduction of a trans double bond between carbon-4 and carbon-5 to produce sphingosine, and introduction of the double bond followed by hydroxylation on carbon-6 to produce 6-hydroxysphingosine. The fatty acid component of the initial ceramide can be hydroxylated on either carbon-2 to produce an α-hydroxyacid or on the terminal carbon to produce an ω-hydroxyacid. At least some of the hydroxylation reactions require vitamin C (Ponec et al. 1997). Ceramides are produced in the viable epidermis and are almost immediately converted to glucosylceramides. The sequence of hydroxylation reactions, double bond introduction, and glycosylation is not established. Most of the glucosylceramides are incorporated into lamellar granules. The lamellar granules also contain glucocerebrosidase (Freinkel and Traczyk 1985; Grayson et al. 1985; Madison et al. 1998), and at the time when lamellar granule contents are extruded into the intercellular spaces, this enzyme converts the glucosylceramides to ceramides. This lipid processing appears to be necessary for formation of mature intercellular lamellae (Holleran et al. 1993).
A special case that should be noted involves the linoleate-containing glucosylceramides and their analogous linoleate-containing ceramides. Some of the linoleate-containing acylglucosylceramide is thought to be associated with the lamellar structures within the granules (Wertz and Downing 1982), whereas most of the lamellar granule-associated acylglucosylceramides are in the bounding membrane of the granule with the glucosyl moiety on the inside (Wertz 2008). The acylglucosylceramide inside the lamellar granules is extruded into the intercellular space, after which it is deglycosylated to yield ceramide EOS. The acylglucosylceramide in the bounding membrane of the lamellar granule is the precursor of ω-hydroxyceramides that become covalently attached to the outer surface of the cornified envelope (Wertz 2000). The acylglucosylceramide is moved into position when the bounding membrane of the lamellar granule fuses into the cell plasma membrane. The process of attachment involves two stereoselective lipoxygenase attacks on the linoleate (Zheng et al. 2011) followed by possible transglutaminase-mediated formation of the ester linkage (Nemes et al. 1999) coupled with or following removal of the oxidized linoleate. This process leads to attachment of glucosyl-ω-hydroxyceramides (Doering et al. 1999). A glucocerebrosidase then removes the glucosyl moiety.
One or more ceramidases may act upon the covalently bound ω-hydroxyceramidases to release the long-chain base component, leaving behind covalently bound ω-hydroxyacid. The free long-chain bases are potent antimicrobials, and this may be an important part of the innate immune system of the skin (Drake et al. 2008).
Structures, Proportions, and Properties
As previously mentioned, the first complete set of epidermal ceramide structures was that from pig (Wertz and Downing 1983). It contained six chromatographically distinct fractions corresponding to ceramides EOS (7.7 wt.%), NS (42.4 wt.%), NP (10.2 wt.%), AS long (12.1 wt.%), AS short (10.5 wt.%), and AP (17.2 wt.%). The ω-hydroxyacids in ceramide EOS were 30- to 34-carbons long, and the sphingoid bases were 16- to 22-carbons long and included sphingosines and dihydrosphingosines. The fatty acids in ceramide NS were mainly saturated entities 24- to 28-carbons in length, and the bases were similar to those found in ceramide EOS. Ceramide NP contained fatty acid components similar to ceramide NS, but the phytosphingosines ranged from 16- to 24-carbons in length. Ceramides AS long and AS short bothcontain long-chain bases similar to those in ceramides EOS and NS. They differ in that ceramide AS long contains mainly 24- to 28-carbon α-hydroxyacids, while ceramide AS short contains almost exclusively α-hydroxypalmitic acid. Finally, ceramide AP contains phytosphingosines similar to those in ceramide NP and α-hydroxyacids similar to those in ceramide AS long.
In humans, the situation is more complicated. There are three ceramides that contain 6-hydroxysphingosine, which is not present in pig, and there is a linoleate-containing ceramide EOP, which is not present in pig (Ponec et al. 2003). The nine ceramide types found in humans are EOS (8.3 wt.%), NS (20.6 wt.%), EOP (6.4 wt.%), NP (18.1 wt.%), EOH (5.0 wt.%), AS(4.4 wt.%), NH (15.4 wt.%), AP (8.7 wt.%), and AH (13.0 wt.%). Chain-length distributions were generally similar to those found in the pig ceramides.
In accord with the finding of three linoleate-containing acylceramides in human epidermis, there are three corresponding covalently bound ω-hydroxyceramides (OS, OP, and OH) in human stratum corneum (Wertz et al. 1989; Robson et al. 1994; Hill et al. 2006).
When isolated, the natural epidermal ceramides are white amorphous solids. Synthetic ceramides, where there is no chain length variation, can be crystalline. It is noteworthy that since ceramides do not have bulky polar headgroups, such as those found in phospholipids, they are cylindrical in shape. This makes them ideal for formation of highly ordered, and thereby impermeable, membranes. In addition, except for the linoleate in the acylceramides, the epidermal ceramides contain very few cis double bonds. This is favorable for resistance of oxidative damage on exposure to air.
Catabolism
Once ceramides reach the stratum corneum, they are relatively stable. There are, however, several ceramidases that can hydrolyze ceramides to release fatty acid and long-chain base. The extent of this hydrolysis under normal circumstances in minimal, probably due to the scarcity of free water in the intercellular spaces. It is, nevertheless, thought that the release of free long-chain bases is biologically significant in that the long-chain bases are potent antimicrobials and may function in the innate immunity of the skin (Drake et al. 2008). There is some evidence that free long-chain bases may also be anti-inflammatory.
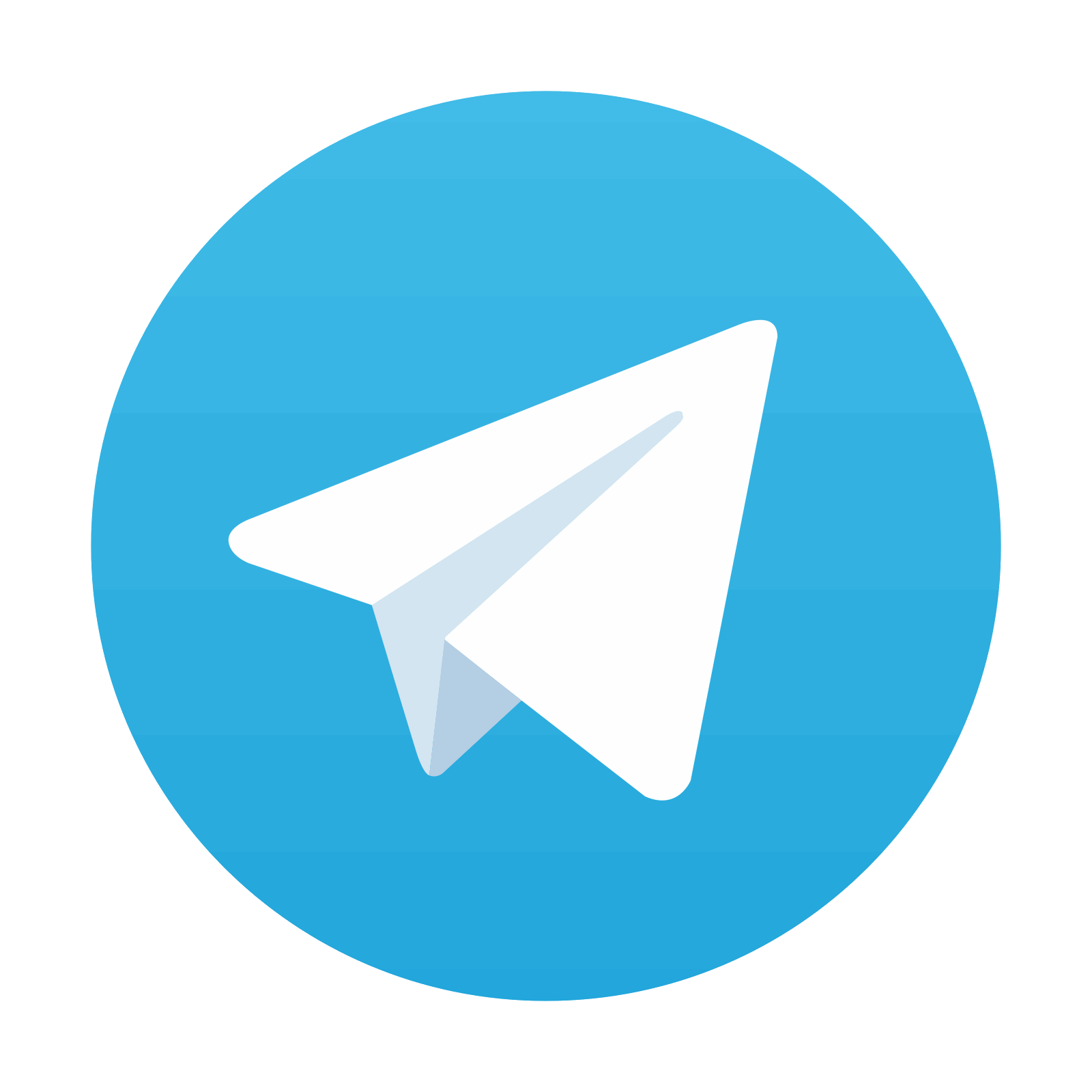
Stay updated, free articles. Join our Telegram channel

Full access? Get Clinical Tree
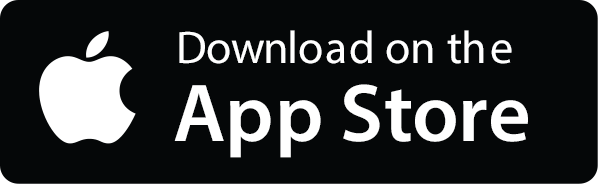
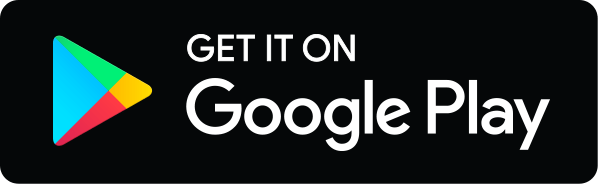