Fig. 14.1
Histopathology of chronic radiodermatitis (hematoxilin and eosin). Hair follicles and sebaceous glands are missing. The connective tissue is relatively homogeneous; many blood vessels are dilated (40×)
The dermis is sclerotic, especially in late lesions [42]. Fibrocytes may have abnormal nuclei and stellate cytoplasm. Giant multinucleate fibrocytes are often observed [42].
Hair follicles and sebaceous glands are absent [41] (Fig. 14.1) unless relatively low doses were applied. Erector pili muscles often remain. There is usually some atrophy of eccrine sweat glands; they may be completely destroyed [41].
Blood vessels are often occluded by thromboses [42]. Telangiectases may be observed in the upper dermis, especially in late lesions [42]. The number of capillaries is often diminished [41]. Endothelial cells may be hyperchromatic [42].
14.4 Radiogenic Ulcers
14.4.1 Definition
The most serious dermatologic complication of radiation therapy is ulceration. This may develop months to years after apparent complete healing of the primary radiation reaction or ulceration can persist after the end of radiotherapy. My colleagues and I have used the term “radiogenic ulcer” for any defect in the irradiated field, which is present at any time more than 8 weeks after the end of radiotherapy and which is not caused by a recurrence of a malignant tumor [5].
14.4.2 Mechanism of Development
Radiogenic ulcers are usually combined injuries [43]. The profound changes in connective tissues and blood vessels caused by ionizing radiation [44] result in a reduced supply of nutrients and oxygen in chronic radiodermatitis. Coexisting diseases, like diabetes mellitus and hypertension, may further reduce this supply. If the demand for nutrients and oxygen is then increased – for example, by inflammation due to excessive sunlight exposure, infection, allergic reaction, or an insect sting – the supply may not be sufficient any longer. Tissue necrosis may then develop.
14.4.3 Incidence
Fortunately radiogenic ulcers after irradiation for a malignant cutaneous tumor are relatively uncommon. This complication has been observed in 3.0 % [31], 5.0 % [24], 5.5 % [20], 6.3 % [5], and 9.4 % [45] of fields in selected series. Radiogenic ulcers were more frequently observed after larger total doses [1, 45]; in larger treatment fields [5, 20]; in older patients [45]; on the scalp [5], lip [5], ear [5, 46], forehead, and temples [5]; and after treatment with harder X-rays (higher half-dose depths) [5]. Slower healing of irradiated fields should be expected on the trunk and limbs [47]. A radionecrosis rate of 9.2 % has been observed on the lower limbs [48]. Large individual doses increase the risk of developing a radiogenic ulcer: Traenkle et al. [49] compared 322 patients who were irradiated with 4 × 10 Gy in 8–10 days with 816 patients treated with either 9 × 5 Gy in 12 days or 13 × 4 Gy in 18 days or 18 × 3 Gy in 25 days. Patients who had received 10 Gy fractions developed radiogenic ulcers in 13.9 % of fields (cumulative probability), while radiogenic ulcers were observed in only 2.9 % of fields following 3–5 Gy fractions. Radiogenic ulcers sometimes develop many years after therapy (Table 14.1). Long follow-up periods are therefore necessary to assess the true incidence of these radiation sequelae.
Table 14.1
Ulcers in the irradiated field after radiotherapy of 1,267 basal and squamous cell carcinomas [5]
Time after irradiation | Ulcers |
---|---|
2 months to 3 years | 50 (63 %) |
>3–6 years | 20 (25 %) |
>6–9 years | 7 (9 %) |
>9–15 years | 3 (3 %) |
Total | 80 (100 %) |
14.4.4 Treatment
Sixty two of the 80 (77.5 %) ulcerations in our series healed permanently with conservative management based on ointments and moist compresses [5]; two lesions (2.5 %) were cured by surgery under local anesthesia. The conservative therapy takes into account the pathogenesis of radiogenic ulcers: moist compresses have an anti-inflammatory effect but do not reduce proliferative activity as corticosteroids do. In addition, local infection has to be controlled by appropriate antibacterial or, if necessary, antifungal ointments. My colleagues and I have used ointments containing gentamycin in most cases. If need be, allergic reactions should be excluded by epicutaneous testing. Traenkle and Dattatreya [49] reported that 90 % of 55 cases of radionecrosis healed with simple topical applications, 80 % of them within 3 months. Spontaneous involution was observed as long as 7 and 8 months after the onset of breakdown. Problematic radiogenic ulcers that require surgery under general anesthesia, that recur, or that cannot be cured at all are rare. In our series, they developed in only 0.9 % of the originally treated fields [5].
14.5 Molecular Basis of the Observed Changes
14.5.1 Biochemical Changes Indicate Cellular Activities
Radiation-induced skin alterations are not solely caused by cell damage with the consequence that the cell dies immediately or later when it tries to divide. Active biochemical processes are involved in these changes [50].
14.5.2 Ceramide
Ionizing radiation increases intracellular ceramide which by several pathways can induce apoptosis of endothelial cells. This mechanism is active after single doses of >5–10 Gy, while after doses of 3 Gy, the release of ceramide is inhibited and anti-apoptotic signaling systems predominate [51].
14.5.3 Collagen Synthesis
Connective tissue changes are mainly caused by an overproduction and deposition of extracellular matrix. An increase of newly synthesized collagen, one of the substances of the extracellular matrix, was found in the skin of irradiated mice as early as 1 week after irradiation [52]. This increase was observed for almost 1 year. Cultured fibroblasts from the skin of irradiated mice produced more collagen as well [52].
14.5.4 Transforming Growth Factor-β1 (TGF-β1)
The increased production of extracellular matrix is mediated by cytokines, particularly TGF-β1 [53, 54]. This cytokine, one of three mammalian isoforms of TGF-β [51, 53, 55], is a homodimeric peptide with a molecular mass of 25 kDa, first purified from human platelets [56], that produces its effects by activating plasma membrane serine/threonine kinase receptors [53]. Cells usually secrete TGF-β1 as a latent complex consisting of the TGF-β1 homodimer, a latency associated peptide, and another high-molecular-weight protein, the latent TGF-β1 binding protein, which binds to the extracellular matrix by disulfide bonds [51, 53]. Ionizing radiation induces enhanced synthesis of TGF-β1. The initial step may be activation of matrix bound latent TGF-β1 by proteolytic enzymes in the presence of reactive oxygen species. Activated TGF-β1 binds to the TGF-β1 receptor type 2 on the membrane of fibroblasts, platelets, macrophages, lymphocytes, epithelial, or endothelial cells. The receptor complex is formed by binding the receptor type 1. The receptor complex starts the TGF-β signaling pathway by activation of kinases [51]. This results in enhanced gene expression and production of TGF-β1 in an autocrine fashion [51]. TGF-β1 signaling is mediated by SMAD proteins, especially SMAD3 [57]. The name “SMAD” genes has been created by combining the abbreviations for related genes in invertebrates [58]: the “small” mutant (SMA) of a gene essential for the male tail ray development of Caenorhabditis elegans and the “mothers against decapentaplegic” gene (MAD) of Drosophila. The activities of TGF-β1 and the importance of the SMAD signaling pathway have been shown in SMAD3-deficient knockout mice: 6 weeks after irradiation less fibrosis, a lower incidence of ulceration and fewer dermal inflammatory cells were observed in irradiated fields of these mice compared to normal controls. Irradiated sites contained less TGF-β1 in cells and extracellular matrix [55]. TGFβ-1 does not only enhance the synthesis of extracellular matrix, but it also induces inhibitors of matrix degrading enzymes [51]. Some SMAD proteins, for instance, SMAD7, can inhibit the TGF-β1 signaling.
The activity of TGF-β1 is similar in normal wound healing and in radiation induced fibrosis. However, in normal wound healing, TGF-β1-related cellular activities are later downregulated, while in irradiated tissue extracellular matrix proteins and TGF-β1 are continuously produced at high level [53].
Blood vessels may be altered by ionizing radiation through a mechanism resembling that for connective tissue changes: irradiation causes the production of TGF-β1 in endothelial cells. TGF-β1 induces the fibrogenic phenotype of vascular smooth muscle cells. Radiation-induced vascular hypertrophy and thickening of the intima are associated with elevated levels of TGF-β1 and SMAD proteins [59].
TGF-β1 inhibits the growth of epithelial, endothelial, and hematopoetic cells [53] and therefore appears at least partially responsible for the epidermal atrophy often found in chronic radiodermatitis.
14.5.5 Other Cytokines
The chronic radiation reaction is not mediated by TGF-β1 alone. Other cytokines are involved including platelet-derived growth factor, interleukin 1, insulin-like growth factor, connective tissue growth factor, and tumor necrosis factor alpha. However, TGF-β1 plays the key role in the fibrotic process because it can modify the activity and expression of most genes coding for growth factors and their receptors [53].
14.6 Standardized Evaluation of Late Radiation Sequelae
To evaluate a specific treatment for a malignant tumor, one has to consider both the percentage of cases cured and the side effects. Results from different studies cannot be compared appropriately if uniform criteria are not used for the assessment of acute and late side effects. In 1995, the Radiation Therapy Oncology Group and the European Organization for Research and Treatment of Cancer have published the LENT-SOMA scoring system [67–72]. The name comprises two acronyms: LENT = late effects normal tissue and SOMA = subjective, objective, management, and analytic. “Late” in this context means more than 90 days after the onset of therapy. The four SOMA criteria are defined as follows: subjective, these are perceptions and problems reported by the patients like pain and pruritus; objective, this is evaluated by the physician through physical examination including conventional radiographs or images and/or results of laboratory procedures; management, this describes the measures necessary to treat the side effects, i.e., medical or surgical intervention; and analytic, this documents the potential deficits that can only be evaluated by more sophisticated methods like computer tomographs or special laboratory tests. LENT SOMA scales were devised for 38 anatomic sites [67], including skin/subcutaneous tissue. The LENT SOMA system is suitable for the documentation of late side effects after radiotherapy for malignant tumors of the skin. Late side effects after treatment(s) can be caused by the tumor and/or its involution. They are not exclusively due to the irradiation of normal tissue. The LENT SOMA system was used in recently published studies [73, 74] for the documentation of late adverse effects in different organs including the skin.
14.7 Radiogenic Skin Cancer
14.7.1 Cancer Risk After Radiotherapy for Benign Dermatoses
A significantly increased risk to develop a basal cell carcinoma of the head and neck could be assessed in two studies, one from Israel and one from New York, after radiotherapy for tinea capitis (ringworm of the scalp) at age <1–15 years [75–80]. The relatively small incidence rates of basal cell carcinomas differed between both studies, but the relative increase was similar (Table 14.2). Follow-up periods and number of patients were large in both studies. No significantly increased risk of other skin cancers or melanoma was found.
Table 14.2
Tumors after radiotherapy for tinea capitis (ringworm of the scalp)
Israeli study | New York study | |
---|---|---|
Number of irradiated patients | 10,834 | 2,224 |
Number of controls | 16,226 | 1,380 |
Age at exposure | <1–15 years | <1–15 years |
Average total dose | 6.8 Gy | 4.8 Gy |
Follow-up | Mean: 24.5 years | Median: 39 years |
Basal cell carcinomasa | ||
Number in irradiated patients | 41 (0.4 %) s b | 127 (5.7 %) s b |
Number in controls | 13 (0.1 %) | 21 (1.5 %) |
Increase in irradiated patients | 4.7-fold | 3.8-fold |
Excess relative risk per Gy | 0.7 | 0.6 |
Other skin cancersa | ||
Number in irradiated patients | 1 (0.009 %) | 11 (0.5 %) |
Number in controls | 2 (0.01 %) | 0 |
Melanomasa | ||
Number in irradiated patients | 2 (0.02 %) | 0 |
Number in controls | 1 (0.006 %) | 0 |
Thyroid cancer | ||
Number in irradiated patients | 103 (1.0 %) s b | 2 (0.09 %) |
Number in controls | 56 (0.3 %) | 0 |
Neural tumorsa | Intracranial tumors | |
Number in irradiated patients | 56 (0.5 %) s b | 16 (0.7 %) s b |
Number in controls | 10 (0.1 %) | 1 (0.07 %) |
Leukemia | ||
Number in irradiated patients | Not evaluated | 8 (0.4 %) |
Number in controls | Not evaluated | 1 (0.07 %) |
Several co-factors influence the carcinogenic risk: (1) the cumulative risk of radiation-induced skin cancer increases with time from exposure [77, 79]. The results of the tinea capitis study from New York [79] with very long follow-up support the belief that an increased skin cancer risk from irradiation continues for a lifetime. (2) The carcinogenic risk of radiotherapy depends on the applied total dose [77]. (3) Ultraviolet light is an important cofactor for the development of X-ray-induced skin cancer in humans. In the study from New York [79], 25 % of both the irradiated and the control patients were African-Americans who are protected by their black skin color against the carcinogenic potential of ultraviolet light: only three basal cell carcinomas occurred in the irradiated patients from this ethnic group, compared to 124 among the irradiated Caucasians. No skin cancer was found among the African-Americans of the control group. The incidence of skin cancer among the Caucasians was significantly lower on the relatively ultraviolet-shielded scalp compared to the margins of the scalp. Significant predictors of skin cancer risk were North European ethnicity, a history of painful sunburns with blisters, and light skin color. In the Israeli study [81], sunbathing in summer was found to be a cofactor for the development of skin cancer.
More neural tumors were found at the head and neck in the Israeli study [76] and intracranial in the New York study (Table 14.2) [80]. An increased frequency of thyroid cancers was observed in the Israeli study only [78]. This is probably due to genetic differences between the patients of both studies [78].
The two large studies after radiotherapy of children and adolescents for tinea capitis unequivocally demonstrate the carcinogenic potential of therapeutic ionizing radiation, but they presently do not have any practical importance because ringworm of the scalp is no longer treated by X-rays. The carcinogenic risk of modern radiotherapy with small doses as used for benign dermatoses is low: Most studies have failed to assess a significant increase of skin cancer [82–86]. Two groups report a significantly elevated likelihood only for individuals irradiated below 20 years [87] or 40 years of age [88] respectively. Patients with basal cell nevus syndrome [85, 89] have a higher risk. Usually only small doses of radiation reach internal organs during radiotherapy of dermatoses with modern techniques [9, 90]. That this treatment induces a malignant tumor in internal organs is therefore highly unlikely [91].
14.7.2 Cancer Risk After Higher-Dose Radiotherapy for a Malignant Cutaneous Neoplasm
The risk of developing a radiation-induced cutaneous malignant tumor following radiotherapy of a malignant neoplasm of the skin has not been evaluated in a controlled study. We assume that this risk is very low in part because (1) radiation fields are usually small [92] and the amount of irradiated skin is an important factor for risk [85]; (2) most patients who are irradiated for a cutaneous malignancy are relatively old and may therefore not experience a radiation-induced skin tumor because their life expectancy is shorter than the long latency period [77, 93–96] for the induction of a neoplasm, and (3) benign dermatoses have often been treated in the past – not in published controlled studies [12, 77, 79, 97] – with repeated courses of fractionated radiotherapy resulting in high cumulative total doses. These treatments cause mutations and thereby more radiation induced skin tumors. Malignant tumors, most importantly, are irradiated with higher dose, single courses in a short time interval resulting in cell death [8, 85]. Dead cells clearly cannot become neoplastic. In animal experiments, the incidence of radiation-induced skin tumors was higher if the same total dose was applied in 64 fractions (a biologically smaller dose), as compared to 16 fractions (a biologically greater dose) [98
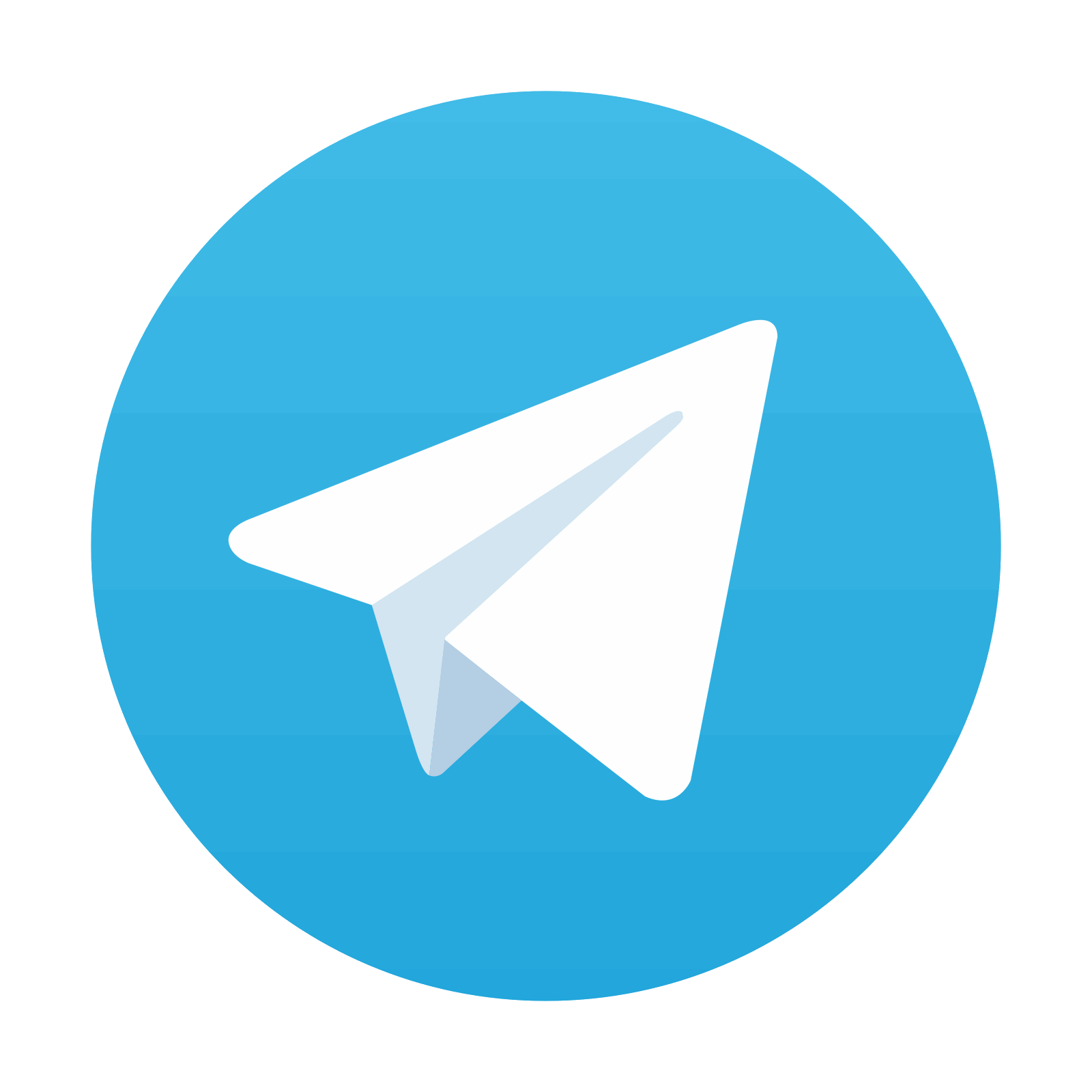
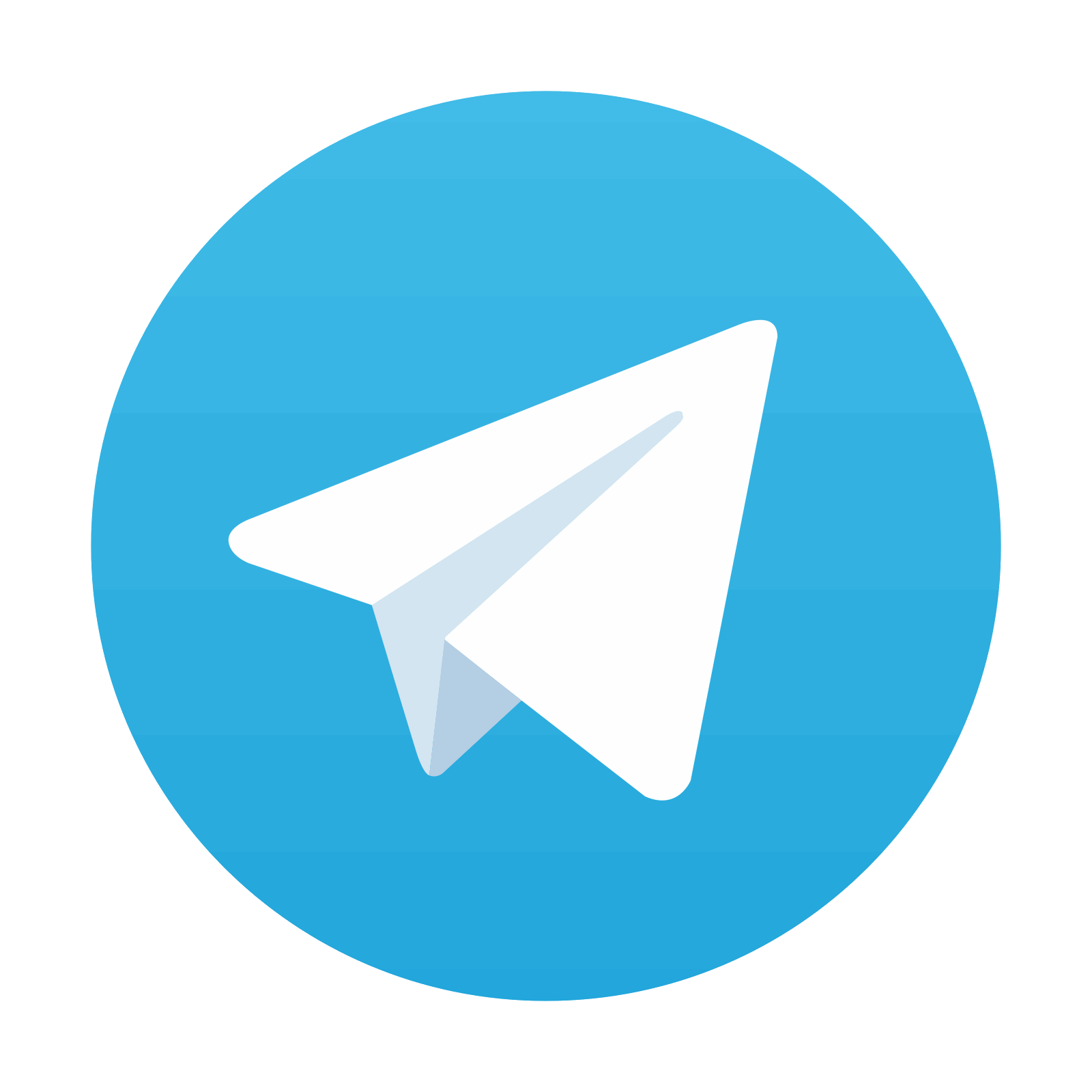
Stay updated, free articles. Join our Telegram channel

Full access? Get Clinical Tree
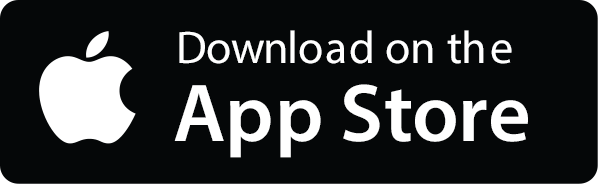
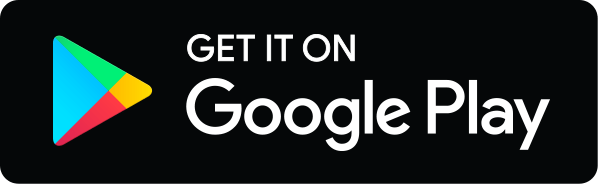