Fig. 10.1
Enzymatic pathway for sapienic acid and related fatty acids Fatty acid desaturase 2 (FADS2) catalyzes two distinctly different types of desaturation reactions, depending on the tissue type. In most tissues, FADS2 is the rate-limiting enzyme that catalyzes the polyunsaturated (PUFA)-type reaction, of which there are two series of noninterconvertible PUFAs. The essential fatty acid (diet-derived) α-linolenic acid (with a double bond located three carbons from the methyl end) is the parent PUFA of the n-3 series (upper grey box), with the metabolite docosahexaenoic acid having a critical role in nervous system function. The essential fatty acid (diet-derived) linoleic acid (with a double bond located six carbons from the methyl end) is the parent PUFA of the n-6 series (lower grey box), with the metabolite arachidonic acid having a critical role in eicosanoid signaling. Under normal conditions, the sebaceous-type reaction (middle grey box) of FADS2 is only found in the human sebaceous gland, where palmitic acid, the parent saturated fatty acid, is converted to the monounsaturated fatty acid, sapienic acid. Sebaleic acid is the predominant PUFA in the human sebaceous gland, being formed by elongation of sapienic acid followed by FADS1-mediated desaturation. Note that the only difference between sebaleic acid and linoleic acid are the positions of the double bonds. Competing reactions for palmitic acid as substrate (outside of the grey boxes) are: (1) desaturation by Stearoyl-CoA desaturase (SCD) to form the monounsaturated fatty acid, palmitoleic acid, and (2) elongation to form the saturated fatty acid, stearic acid, which can then be desaturated by SCD to produce oleic acid. Note that the only difference between sapienic acid and palmitoleic acid is the position of the double bond. The green box highlights the similarity of the first 9 carbons (from the carboxyl terminal) of the FADS2 substrates with chain length of 16 or 18 carbons: the PUFAs linoleic and α-linolenic acids and the saturated fatty acid, palmitic acid. Despite the large structural differences towards the methyl end of these fatty acids (which plays a role in the higher affinity of PUFAs for FADS2), the molecular similarity toward the carboxyl end (green box) allows these fatty acids to be desaturated by a single FADS2 enzyme, in which the catalytic site (between carbon 6 and 7) is determined relative to the carboxyl terminal. All FADS2-relevant reactions and structures are indicated in red, with relevant carbons greater than 6 resulting from elongation and/or beta-oxidation. The various naming conventions for the fatty acids are indicated: common name (including the abbreviation used in this article) and/or systematic name, shorthand notation indicating chain length-/number of double bonds-/position of double bonds, and shorthand notation indicating chain length-/number of double bonds-/fatty acid series based on double bond closest to methyl end (n-3 or n-6 PUFA families; n-7, n-9, n-10 for MUFA families). All SCD-relevant reactions and structures are indicated in blue. Not all metabolites are shown for the PUFA (n-3, n-6) and MUFA (n-7, n-9, n-10) fatty acid families
Given the rare distribution of SA/6-HA in nature, and the biochemical complexity of its production (discussed below), a central question arises: “With regard to human sebum, what is the advantage of SA versus its positional isomer, POA, given that fatty acids with a double bond located at the ninth carbon from the carboxyl end (∆9-desaturated) are present in the sebum of most other species, including the vernix caseosa (embryonic human sebum)?” This is a difficult question to answer at this time, since the precise function(s) of human sebum as a whole are unclear. However, clues may be gained by the examination of related questions such as: (1) where is SA produced, (2) how is SA produced, (3) are there human disorders that correlate with altered levels of SA, and (4) what are the functions of similar MUFAs in other systems?
Occurrence of Sapienic Acid
High concentrations of naturally occurring SA/6-HA are documented in only two places in the biosphere: human sebum (Nicolaides 1974) and the seed oil of the black-eyed Susan vine, Thunbergia alata Bojer ex Sims (Cahoon et al. 1994). Up to 47 % of human sebum is free fatty acid (FFA; Kohler et al. 2009), of which SA is dominant, second only to palmitic acid (PA), the substrate for its production (Nicolaides 1974; Ge et al. 2003; Fig. 10.1). In Thunbergia alata, 6-HA comprises over 80 % of seed oil by weight (Spencer et al. 1971; Cahoon et al. 1994). That abundant SA is found in human skin and 6-HA in a plant seed suggests that other natural sources may be found, especially with development of increasingly sensitive analytical methods. Indeed, 6-HA has been detected at low levels in oceanic particulate matter (Nichols et al. 1989), mushrooms (Marekov et al. 2012), neonatal rat brain (Spence 1970), and human blood plasma (Sansone et al. 2013). In addition, the geometric isomer, trans-6-hexadecenoic acid is present, again at low levels, in some marine animals (Ackman et al. 1971; Hooper and Ackman 1971) and in human blood plasma (Sansone et al. 2013). (It is noted that the vast majority of biologically active unsaturated fatty acids (UFAs) have the cis (or Z) conformation). In contrast to the abundant sources of SA in human sebum and 6-HA in the seed oil of Thunbergia alata (where the synthetic pathways are known), the formation, and in some cases the source, of the low abundance 6-HA isomers is not defined.
Biosynthesis of Sapienic Acid
Despite the evolutionary distance between animals and plants, there are similarities with regard to the synthesis and high accumulation of SA/6-HA: (1) PA (16:0) is directly desaturated between the sixth and seventh carbons (from the carboxyl end) by a ∆6-desaturase in both human (FADS2; Fatty Acid Desaturase 2; also known as delta(∆)6-desaturase; Fig. 10.1) and plant (∆6 16:0-acyl carrier protein (ACP) desaturase, (2) triacylglycerol is the most prevalent lipid class in human sebum and T. alata seed oil and is the main lipid to which SA/6-HA is esterified, and (3) specialized biochemical pathways are required for the generation and utilization of this highly unusual fatty acid.
FADS2 and the “PUFA-Type” Reaction
FADS2 is a member of the fatty acid desaturase gene family, which in mammals includes SCD (Stearoyl-CoA Desaturase; also known as FADS5 and ∆9-desaturase), FADS1 (also known as ∆5-desaturase), and FADS3 (putative ∆13-desaturase; Guillou et al. 2010; Rioux et al. 2013). The combined activity of the human gene family is responsible for the production of (1) the endogenous MUFAs POA (16:1∆9) and OA (18:1∆9) by SCD; SA (16:1∆6) by FADS2; (2) the polyunsaturated fatty acids (PUFAs) such as arachidonic (AA, 20:4n-6), eicosapentaenoic (EPA, 20:5n-3), and docosahexaenoic (DHA, 22:6n-3) acids, via a multi-enzyme pathway that includes FADS1, FADS2, and elongases (Guillou et al. 2010; Fig. 10.1). With the exception of SA (which is mainly secreted to the skin surface), these MUFAs and PUFAs are used internally for multiple functions that include cellular structure, energy storage, and lipid signaling, as exemplified by membrane phospholipids, triacylglycerols, and eicosanoids, respectively (Nakamura and Nara 2004).
FADS2 is the rate-limiting enzyme in the formation of PUFAs, in which the essential fatty acids (EFA; obtained from diet) linoleic acid (LA; 18:2∆9,12) and α-linolenic acid (ALA; 18:3∆9,12,15) are desaturated between the sixth and seventh carbons from the carboxyl terminal to give γ-linolenic acid (18:3∆6,9,12) and stearidonic acid (18:3∆6,9,12,15), respectively (Pereira et al. 2003; Fig. 10.1). γ-linolenic acid undergoes further rounds of elongation, desaturation (by FADS1 and FADS2), and beta-oxidation to form the biologically important lipids AA (20:4n-6, 20:4∆5,8,11,14) and docosapentaenoic acid (22:5n-6, 22:5∆4,7,10,13,16). Since these PUFAs are derived from LA (in which the first double bond counting from the methyl end is at carbon 6), they are part of the n-6 series of EFAs (Pereira et al. 2003; Fig. 10.1). Stearidonic acid undergoes similar rounds of elongation, desaturation, and beta oxidation to form EPA (20:5n-3, 20:5∆5,8,11,14,17) and DHA (22:6n-3, 22:6∆4,7,10,13,16,19). These PUFAs are derived from ALA (in which the first double bond counting from the methyl end is at carbon 3) and are part of the n-3 series of EFAs (Fig. 10.1). Severe multiorgan dysfunction occurs when the diet is deficient in the essential fatty acids LA and ALA, a condition known as essential fatty acid deficiency (Gurr et al. 2002b). For example, a deficit of EFAs during critical periods of brain development can result in permanent deficits in a wide spectrum of neurological function (Richardson 2004). Similarly, the epidermal barrier requires LA, without which it becomes excessively permeable to water, resulting in life-threatening dehydration (Gurr et al. 2002b). Given the fundamental roles of the n-6 and n-3 PUFAs for membrane homeostasis and for lipid signaling, and the position of FADS2 at the beginning of these metabolic pathways, expression of FADS2 and the concomitant ∆6-desaturase activity is widespread among mammalian organs (Cho et al. 1999; Ge et al. 2003). Consistent with substrates that are PUFAs themselves, FADS2 is a member of the “front-end” subgroup of desaturases that introduce a double bond into a fatty acid already containing two or more double bonds, and does so at the “front-end” of the fatty acid, or between the carboxyl end and the first double bond closest to this end, usually located between the ninth and tenth carbons (Meesapyodsuk and Qiu 2012; Fig. 10.1). This “PUFA-type” catalytic activity is present (albeit at variable levels) in all organs, with one notable exception, the sebaceous glands of human skin. In this singular human tissue, FADS2 catalyzes a “sebaceous-type” reaction to produce high levels of naturally occurring SA, which is secreted to the skin surface as the major MUFA of sebum (Ge et al. 2003; Fig. 10.1).
FADS2 and the “Sebaceous-Type” Reaction
The discovery of FADS2 as the major desaturase of human sebaceous glands and the demonstration of SA production by cells overexpressing human FADS2 conclusively demonstrated that the same FADS2 gene that carries out the PUFA-type reaction also carries out the sebaceous-type reaction (Ge et al. 2003). However, prior to this discovery, the existence of the sebaceous-type reaction catalyzed by a ∆6-desaturase was postulated by several independent lines of investigation. Researchers characterizing sebaceous lipids were the first to identify (and name) SA, and based on the family of sebum fatty acids with the highly unusual double bond position between the sixth and seventh carbons (from the carboxyl terminal), they correctly postulated that a ∆6-desaturase activity directly desaturates PA (16:0) to form SA (16:1∆6; Nicolaides 1974; Stewart and Downing 1991). Similarly, early work characterizing the catalytic mechanism of PUFA formation in rat liver microsomes demonstrated the formation of low levels of 6-HA (16:1∆6) when SCD activity was inhibited in the same preparation by an inhibitor specific to ∆9-desaturase (Pollard et al. 1979). Without the genomic information of the FADS2 gene and transcripts, it was not possible to conclusively determine if these ∆6-desaturase activities were carried out by one or multiple FADS2 isoforms. Indeed, studies of substrate preference (18:3∆9,12,15 > 18:2∆9,12 > 18:1∆9) and competitive inhibition (18:3∆9,12,15 > 18:2∆9,12 > 18:1∆9; Brenner and Peluffo 1966) would be more consistent with different ∆6-desaturases, one acting on PUFA substrates and the other acting on saturated fatty acid (SFA) substrates. Finally, as more ∆9-desaturases were being cloned from various organisms including nematodes (Watts and Browse 2000) and mice (Zheng et al. 2001; Miyazaki et al. 2006), it was demonstrated that different ∆9-desaturated MUFAs (POA and OA) were generated by different SCD isoforms, each with a different substrate specificity. In combination, the above data was insufficient to link a single FADS2 gene to both the PUFA-type and sebaceous-type reactions, a conclusion that required cloning and characterization of the FADS2 locus (Cho et al. 1999; Marquardt et al. 2000), tissue localization of FADS2 mRNA in skin (Ge at al. 2003), and an in vitro enzyme assay using overexpressed human FADS2 enzyme and PA as a substrate (Ge et al. 2003).
Mechanism of Sapienic Acid Biosynthesis in Human Sebaceous Glands
Given the above information, how does the human sebaceous gland produce such high levels of this unusual MUFA using a desaturase whose preferred substrates are PUFAs? In this case, the biochemical objective is to enable the FADS2 sebaceous-type reaction (Fig. 10.1), which due to its inherent inefficiency, requires multiple mechanisms to modulate substrate, product, and enzyme, such that the equilibrium of the reaction is shifted to production of SA. Several factors contribute to the need for this complex tissue-specific regulation: (1) SCD and FADS2 are ubiquitously expressed due to the cellular requirement of de novo synthesis of MUFAs and biosynthesis of PUFAs, respectively, for membrane homeostasis, energy, and signaling, (2) PA (16:0) is one of two natural SFA substrates for SCD (the other being stearic acid, 18:0; Fig. 10.1), and as such is much more efficiently desaturated by SCD than by FADS2 (Guillou et al. 2003), (3) FADS2 has evolved to recognize fatty acid substrates with two or more cis double bonds (Sperling et al. 2003; Gostincar et al. 2010), thus SFAs have very low affinity, as indicated by the low ∆6-desaturation of SFAs (even in the presence of an SCD inhibitor) as compared to the high ∆6-desaturation of ALA to stearidonic acid (PUFAs) in the same microsomal preparation (Pollard et al. 1979; Fig. 10.1). The adaptations to overcome these factors include (1) removal of competing desaturase activity for PA, (2) removal of PUFA substrates that compete for binding of PA to FADS2, (3) accumulation of high levels of the substrate PA, (4) efficient sequestration of the product SA (esterification into triacylglycerols, wax esters, and cholesterol esters), and (5) high levels of FADS2 enzyme activity.
Of these various mechanisms, removal of competing SCD activity for PA (Guillou et al. 2004) is likely the most critical. Early evidence linking SA to desaturase competition between FADS2 and SCD for PA was (1) the observation that the vernix caseosa (human embryonic sebum) contains ∆9-desaturated POA (16:1∆9) which decreases in the perinatal period, and with onset of puberty, is replaced with ∆6-desaturated SA (16:1∆6) in sebum (Nicolaides et al. 1972; Nazzaro-Porro et al. 1979), and (2) using rat liver microsomes, 6-HA could be produced from PA only when an inhibitor of SCD was included in the reaction (Pollard et al. 1979). More recently, when primary sebocytes (pediatric source) undergo differentiation and lipogenesis, lipid analysis shows a high SA to POA ratio, similar to adult sebum (McNairn et al. 2013). Inhibition of SCD mRNA expression/accumulation is likely the primary means of reducing SCD competition for PA, suggested by weak/undetectable levels of SCD mRNA in sebaceous glands of human scalp hair follicles (Ge at al.2003; Fig. 10.2), and is further supported by evidence indicating that SCD activity is primarily controlled by transcription (Mauvoisin and Mounier 2011) and mRNA turnover (Ntambi 1999). Findings in other species reinforce this concept of desaturase competition. 6-HA is only detected in the preputial gland of mice with a targeted deletion of the Scd1 gene (Miyazaki et al. 2002), and transfection of recombinant rat Fads2 in COS-7 cells produced more 6-HA from PA when Fads2 was expressed alone versus when Fads2 and Scd1 were co-expressed (Guillou et al. 2003). With the lack of SCD activity in the human sebaceous gland, FADS2 is now in the unique situation of having to desaturate PA (a SFA) in the presence of its natural substrate LA (a PUFA). This is likely accomplished by removal of LA via expression of 15-lipoxygenase-2 (15-LOX-2) in human sebaceous glands. This mechanism is strongly supported by two lines of evidence: (1) localization studies of human sebaceous glands in vivo show that FADS2 (Ge et al. 2003; Fig. 10.2) and 15-LOX-2 (Shappell et al. 2001) mRNA and protein are both expressed in differentiated sebocytes and not in the undifferentiated stem cells adjacent to the basement membrane (Fig. 10.2), and (2) explant cultures of human sebaceous glands specifically oxidize and degrade LA but not SFAs or MUFAs (Pappas et al. 2002)—an activity which correlates with the ability of the explant to produce sebaceous-specific lipids such as wax esters and squalene (Nicolaides 1974). LA and AA (metabolite of LA) are the natural substrates for 15-LOX-2, which results in their oxidation and multistep conversion to their respective hydroxy fatty acids, 13-hydroxyoctadecadienoic acid (13-HODE) and 15-hydroxyeicosatetraenoic acid (15-HETE; Brash et al. 1997). Oxidized PUFAs (via oxygenases or non-enzymatic peroxidation) are termed oxylipins and are characterized by potent bioactivity, short half-lives, and tight regulation (Tourdot et al. 2014). Once formed, these bioactive lipids have several roles: signaling, induction of structural changes by provoking secondary oxygenations, and entering beta-oxidation for energy production (Feussner et al. 1997; Brash 1999). This multifunctionality could impart a dual function for 15-LOX-2 in human sebaceous glands: (1) shielding FADS2 from LA, its preferred PUFA substrate by metabolizing LA to oxylipins that are not substrates for FADS2 and/or promoting catabolism of LA by beta-oxidation, and (2) promoting sebocyte differentiation by activating peroxisome proliferator-activated receptor gamma (PPARG)-dependent transcription of lipid metabolic genes. Several independent lines of research, when considered in aggregate, suggest a link between (1) the PUFAs LA, AA, and their oxylipin metabolites generated by 15-LOX-2, (2) a differential effect of PPARγ on FADS2 and SCD, and (3) the role of PPARγ in sebaceous gland homeostasis. 13-HODE is an endogenous activator of PPARγ in macrophages following uptake of oxidized LDL (Nagy et al. 1998) and 15-HETE activates PPARγ and inhibits proliferation (promotes differentiation) in prostate cancer cells (Tang et al. 2002). Treatment of HepG2 cells with pioglitazone (PPAR-γ agonist) increases FADS2 mRNA and enzyme activity but not that of SCD (Saliani et al. 2013). Using primary sebocytes generated from explanted sebaceous glands from pediatric donors, treatment with LA induces lipogenesis that correlates with increased mRNA for PPARγ and FADS2, and lipid analysis indicates that SA (FADS2-derived) predominates over POA (SCD-derived), demonstrating a differential desaturase response, as well as remarkable lipid similarity to in vivo human sebum (McNairn et al. 2013). Cultured rat preputial sebocytes undergo differentiation and lipogenesis when treated with LA or with a PPARγ agonist (Rosenfield et al. 1999).
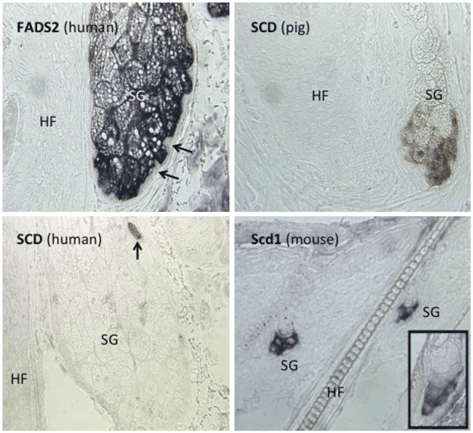
Fig. 10.2
In situ hybridization reveals expression of FADS2 and SCD1 mRNA in sebaceous glands Upper left : Fatty Acid Desaturase 2 (FADS2) is abundantly expressed in human sebaceous glands (SG), with highest level in the early differentiating sebocytes immediately centripetal to the basal stem cells (arrows), which do not express FADS2 mRNA. HF, hair follicle. Lower left: SCD mRNA is not expressed in human sebaceous glands (SG), with the exception of rare sebocytes (arrow). HF, hair follicle. Upper right: SCD mRNA is expressed in pig sebaceous glands (SG). HF, hair follicle. Lower right : Scd1 mRNA is expressed mouse sebaceous glands (SG). Inset demonstrates Scd1 expression is restricted to the lower half of the mouse sebaceous gland. The lack of expression of SCD in the majority of sebocytes in the human sebaceous gland, and the strong expression of SCD in pig and Scd1 in mouse sebaceous glands, suggest that SCD gene expression is repressed in human sebaceous glands
Driving the sebaceous-type reaction toward product formation (SA; 16:1∆6) requires efficient removal of product as well as efficient accumulation of substrate (PA; 16:0). Early work on characterization of sebaceous lipids demonstrated that sebaceous glands preferentially accumulate fatty acids with a chain length of sixteen carbons. Triacylglycerols and wax esters together account for ~ 50 % of total sebum lipids in humans (Nicolaides 1974), with PA (16:0) and SA (16:1∆6) comprising more than 80 % of the fatty acids that are esterified in these two lipid classes (Green et al. 1984; Marzouki et al. 1988). Additionally, human sebaceous gland organ cultures preferentially incorporate PA over other fatty acids during synthesis of wax esters (Pappas et al. 2002), indicating selective utilization of 16 carbon fatty acids in sebum production. It is also possible that human sebaceous glands are less efficient in elongating PA (16:0) to stearic acid (18:0), thus leading to accumulation of PA. While several Elovl (elongation of very long chain fatty acids) genes are expressed and/or function in mammalian skin [(Elovl1 (Tvrdik et al. 2000; Wang et al. 2005; Sassa et al. 2013), Elovl3 (Wang et al. 2005), Elovl4 (Umeda et al. 2003)], including the sebaceous gland [(Elovl3 (Westerberg et al. 2004) and Elovl4 (McMahon et al. 2014)], no such evidence is available for Elovl6, which is the most efficient elongase for converting PA (16:0) to stearic acid (18:0; Matsuzaka et al. 2007; Green et al. 2010; Kihara 2012). Reduced ELOVL6 in human sebaceous glands may be related to lack of SCD expression; these two genes are coordinately regulated in vivo (Matsuzaka et al. 2007; Shimano 2012). Targeted deletion of Elovl6 in mice results in decreased expression of Scd1 and a shift of from 18-carbon predominant FA to 16-carbon predominant FA (Matsuzaka et al. 2007).
In addition to substrate accumulation, maintenance of such high levels of MUFA production requires efficient sequestration of SA. Esterification into the various classes of sebaceous lipids provides the “sink” for SA, which at the same time prevents accumulation of free fatty acids which are toxic to cells (Park et al. 2014). Once PA is desaturated by FADS2 to produce SA, there are three main pathways for further metabolism: (1) esterification into triacylglycerol (TG), for which DGAT1 and DGAT2 are the rate limiting enzymes (Wakimoto et al. 2003), (2) esterification into wax esters (WE), which is catalyzed by AWAT1 and AWAT2 (Turkish et al. 2005), and (3) conversion into sebaleic acid, a PUFA that is specific to human sebaceous glands (discussed below).
Finally, since the sebaceous-type FADS2 reaction is inefficient compared to the PUFA-type reaction, increased FADS2 mRNA and protein may provide compensation. FADS2 mRNA is the most abundant transcript in a normal human skin cDNA library (NCI_CGAP_Skn3, NCBI Unigene library 8848), comprising 150 out of 7979 (1.9 %) expressed sequence tags (ESTs). For comparative purposes, α-actin and type I myosin heavy chain comprise 1.9 and 1.5 %, respectively of mRNAs from a normal skeletal muscle cDNA library (Welle et al. 1999). Myofibril transcripts are highly abundant in skeletal muscle and they represent a class of mRNAs that are “most often observed when a cell is producing an enormous quantity of a particular protein or is highly specialized or differentiated to perform a unique function” (Farrell 2005). FADS2 expression is highly restricted in human skin to a subset of sebocytes (Ge et al. 2003). Thus the abundance of FADS2 mRNA in sebocytes is even greater than the 1.9 % detected in normal human skin. This suggests that a major function of human sebaceous glands is to produce and secrete SA to the skin surface, and that extremely high levels of FADS2 mRNA and protein are required to support this “industrial scale” production.
Possible Roles of Sapienic Acid
Sapienic acid is involved in the synthesis of the other human-specific sebaceous fatty acid, sebaleic acid (18:2Δ5,8; Nicolaides 1974; Stewart et al. 1986; Fig. 10.1). This is the product of SA elongation by 2 carbons, followed by insertion of a FADS1-mediated double bond between the fifth and sixth carbon from the carboxyl end, a reaction and metabolite that occurs only in human sebaceous cells and has not been reported elsewhere. It has been demonstrated that neutrophil 5-lipoxygenase can convert sebaleic acid to 5-HODE, which can be further metabolized to 5-oxo-ODE by 5-hydroxyeicosanoid dehydrogenase, not only in neutrophils but also in keratinocytes (Cossette et al. 2008). Because of its chemoattractant properties, sebum-derived 5-oxo-ODE could be involved in neutrophil infiltration, which is relevant to inflammatory skin diseases such as acne, and atopic dermatitis, though this has not been elucidated as yet. Regarding acne, as sebum secretion increases with the onset of puberty, LA in epidermal acylceramides (required for barrier function) can be replaced with SA (Stewart et al. 1986), which could result in a compromised barrier and hyperkeratinization of the infrainfundibulum, an early event in comedogenesis (Knutson 1974; Downing et al. 1986). Additional suggestive evidence for a pathogenic role of sebum in acne, and in particular FADS2-derived SA and/or sebaleic acid, is the dramatic suppression of sebum and improvement in subjects treated with eicosatetraynoic acid (Strauss et al. 1967), an alkyne isomer of AA and an inhibitor of FADS2 (Nakamura et al. 2000).
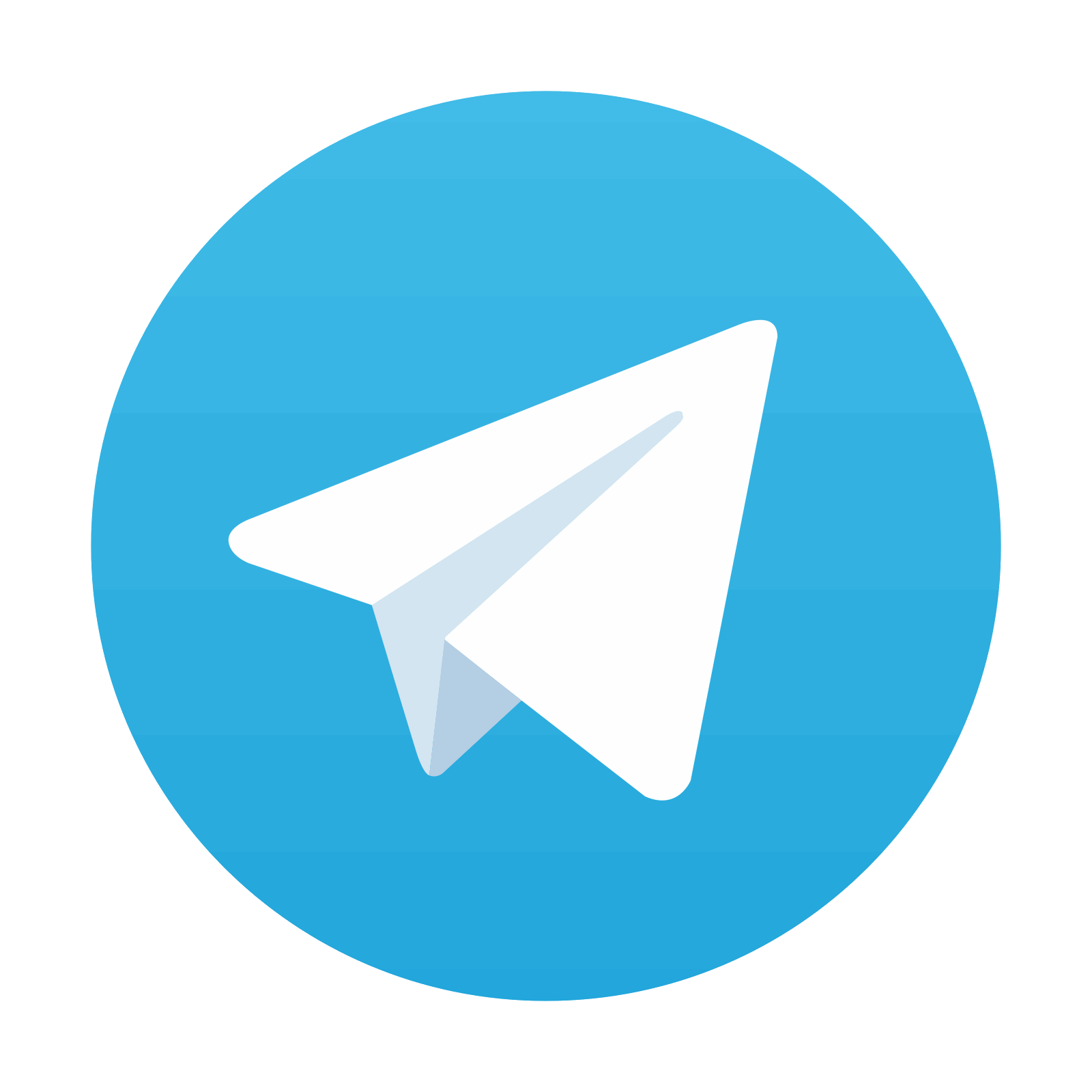
Stay updated, free articles. Join our Telegram channel

Full access? Get Clinical Tree
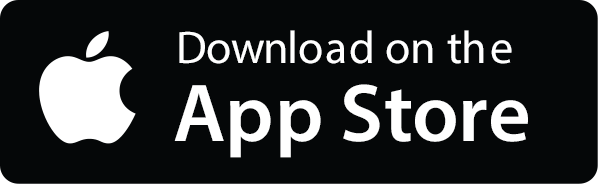
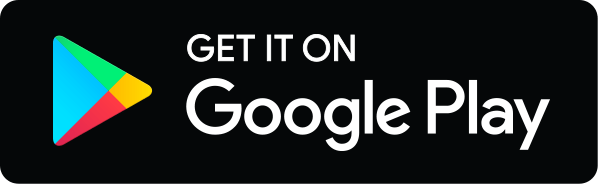