30 Dynamic posterior stabilization is now a popular form of surgical intervention employing a highly diverse range of devices. These devices are designed to relieve several different pathological conditions, including spinal stenosis and diskogenic pain, by fulfilling a range of biomechanical functions. The purpose of this chapter is not to describe individual devices in great detail or to evaluate their clinical effectiveness in different applications because this will be covered in subsequent chapters. The proposed biomechanical actions of these devices will be analyzed in generic terms so that the reader develops an understanding of the fundamental principles involved in their usage. Simplistically, dynamic posterior stabilization devices have the potential for affecting the spine in several ways: The extent to which a given device achieves any or all of the foregoing depends upon its design and mode of action and also on the mechanics of the segment that is treated. Posterior stabilization devices fall within two broad categories of design: interspinous process spacers and pedicle screw–based systems. Interspinous spacers have the potential to cause the segment to flex, distract the disk space, block extension, unload the disk, and subtly change loading and motion patterns in extension. Those that have additional tension bands, such as the Wallis Mechanical Normalization System (Abbott Spine, Inc., Austin, TX), also have the potential to limit flexion and modify flexional loading and motion patterns. Pedicle screw based–systems have the potential to modify the full range of segment behavior. The extent to which these potentials can be achieved is discussed in the remainder of this chapter. All posterior stabilization devices have the potential to increase the flexion or extension angle of the unloaded segment. The degree to which they do this, or indeed if they do it at all, depends on the size of the device relative to the separation of the pedicle screws or spinous processes. For example, if the spacer height of a Dynesys Dynamic Stabilization System (Zimmer Spine, Inc., Warsaw, IN) is greater than the separation of the pedicle screws in the neutral position, the segment will be forced into flexion, whereas if they are shorter it will be forced into extension. Tension band–only devices, such as the Graf Ligament System (Neoligaments, Leeds, U.K.), will force the segment into extension, the degree of angulation being dependent upon the tension in the ligament. This ability comes from the fact that the posteriorly positioned device can apply a bending moment to the segment. Motion segments have a highly nonlinear bending moment versus bending angle relationship where, close to the neutral position, small applied bending moments result in large, angular displacements (Fig. 30–1). It is therefore relatively easy for a device to apply a bending moment that is sufficient to alter the angulation of the segment. It should be noted that in more complex devices such as the Dynesys, the relationship between spacer length and flexion-extension angle is complicated by the application of tension through the cord, which results in postimplantation deformation of the spacer. For example, application of the standard tension to the cord of a Dynesys device will result in a 2 mm shortening of the spacer. Such deformation must be taken into account if it is wished to control the flexion-extension angle of the segment using the device. Study of Fig. 30–1 indicates why it is very difficult to use a posterior stabilization device to change the neutral position disk height of a segment (i.e., to apply distraction). Clearly it is possible to apply many devices in such a way as to apply a distractive force either to the spinous processes or the pedicles. Such a force is, however, applied posteriorly to the ICR of the segment and will therefore also apply a bending moment. This bending moment is greater for a given distractive force the more posteriorly the device is mounted. From Fig. 30–1 we can see that from the neutral position (the origin of the graph) comparatively small moments are required to cause relatively large changes of angulation. However, the intervertebral disk is very stiff (~2 kN/mm) in the axial direction. Very large forces must therefore be applied to achieve small amounts of distraction. Hence, application of distraction forces using posterior stabilization devices will result in flexion rather than distraction. The only circumstance under which this is not the case is if the stabilization device is itself very stiff to bending. It will then be able to apply an opposing bending moment to the segment that will prevent it from flexing. However, such rigid fixation devices by definition do not stabilize dynamically; they will prevent any form of bending to the segment. It is possible for a device to prevent loss of disk height, particularly in extension. Consider, for example, the X-STOP Interspinous Process Decompression system (St. Francis Medical Technologies, Inc., Alameda, CA), which provides no rigidity in flexion, but which becomes very stiff in extension when the spinous processes come into contact with the titanium spacer. In this case the ICR will move posteriorly, attracted to the point of maximum stiffness (see later for a detailed explanation). The segment will then rotate about the device (Fig. 30–2) maintaining vertebral canal width and distracting the disk. This is an example of a device with low stiffness in some situations (allowing motion) and high stiffness in others (preventing motion). Figure 30–1 A typical bending moment versus flexion angle for a motion segment. Note the neutral zone where small changes in bending moment result in large changes of angle. Control of sagittal plane bending has been the primary design criterion for the majority of dynamic stabilization devices. The degree to which they achieve this, and under what circumstances, is more fully described in the device-specific chapters. The fundamental principles by which they operate is discussed in this chapter.
Rationale for Dynamic Stabilization
Modes of Action of Dynamic Posterior Stabilization Devices
Modification of Neutral Angle and Disk Space Height
Control of Sagittal Plane Bending
Unloading of the Intervertebral Disk
Modification of Motion of the Treated Segment
Modification of the Distribution of Loads within a Segment
Control of neutral posture of the segment. Many devices aim to modify the neutral angulation of the affected segment or to distract the disk space or both.
Control of sagittal plane bending of the treated level. Most devices aim to prevent extremes of either or both flexion and extension without totally preventing such motion.
“Unloading” of the intervertebral disk of the treated level. There is a hypothesis, discussed further later in this chapter, that diskogenic pain results from an overloading of the disk that can be prevented through surgical intervention. Several devices aim to share a proportion of the compressive load, hence reducing the magnitude of disk loading.
Modification of the motion of the treated segment. The spinal motion segment is a mechanically complex structure; its response to applied bending moments arises from the sum of the effects of its component parts. Hence, any surgical intervention is going to change its behavior in bending. In particular, the effect of devices on the instantaneous center of rotation (ICR) of the segment will be discussed. More importantly, an insight into the functional/clinical relevance of the ICR will be introduced.
Modification of the distribution of loads within the segment, and in particular within the intervertebral disk. It should be remembered that for all mechanical systems deformation and load are linked. If a device modifies how the segment deforms; for example, by changing the position of the ICR, this can have dramatic effects on the internal distribution of loads.
Modes of Action of Dynamic Posterior Stabilization Devices
Modification of Neutral Angle and Disk Space Height
Control of Sagittal Plane Bending
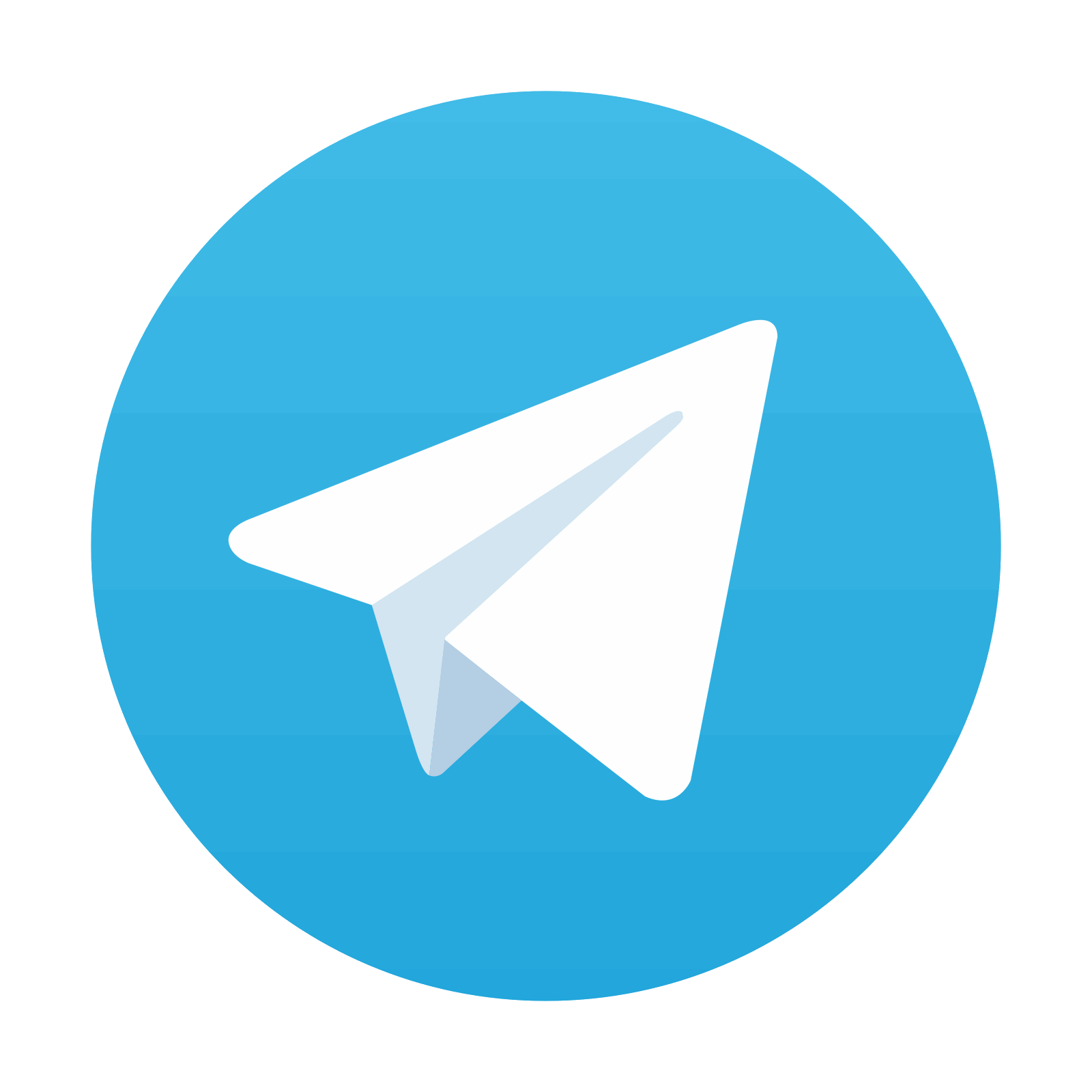
Stay updated, free articles. Join our Telegram channel

Full access? Get Clinical Tree
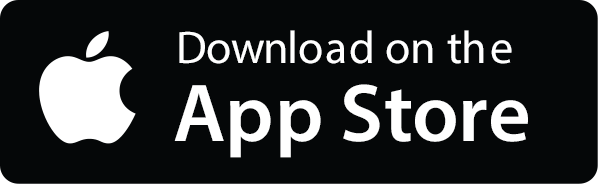
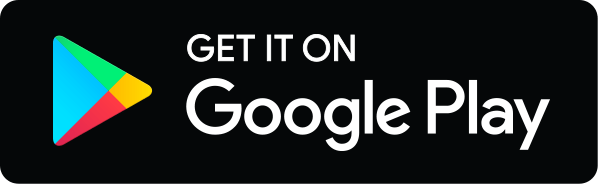