Fig. 4.1
Schematic representation of cyclooxygenase-mediated pathways leading to the biosynthesis of prostanoids from arachidonic acid. COX cyclooxygenase, PGES prostaglandin E synthase, PGDS prostaglandin D synthase, PGFS prostaglandin F synthase, PGIS prostacyclin synthase, TXAS thromboxane A synthase
PGE2 is the dominant cutaneous prostanoid and is produced by almost all cutaneous cell types (Black et al. 1978; Pentland and Needleman 1986; Ziboh 1992; Cho et al. 2005; Sugimoto et al. 2006; Rhodes et al. 2009; Gledhill et al. 2010). It is formed via the cytosolic (cPGES), and microsomal (mPGES-1 and mPGES-2) prostaglandin E synthases (Kudo and Murakami 2005; Murakami and Kudo 2006). Evidence for linked expression of the inducible mPGES-1 and COX-2 isozymes indicates the presence of an efficient system for increased PGE2 production upon stimulation (Ueno et al. 2005). PGD2 is produced via the hematopoetic-type (H-PGDS) or the lipocalin-type (L-PGDS) prostaglandin D synthases (Urade and Eguchi 2002), while its further non-enzymatic hydrolysis gives rise to anti-inflammatory cyclopentanone PGs such as PGJ2 and 15d-PGJ2 (Surh et al. 2011; Scher and Pillinger 2005). PGD2 is produced by Langerhans cells, dermal mast cells and melanocytes, and it is believed to be involved in immune and allergic responses (Shimura et al. 2010; Ujihara et al. 1988; Ikai and Imamura 1988). Interestingly, Langerhans cells and mast cells express H-PGDS while melanocytes express L-PGDS reflecting their neural crest origin (Takeda et al. 2006; Masoodi et al. 2010; Shimura et al. 2010). PGF2α can be produced via the PGH2 9,11-endoperoxide reductase (prostaglandin F synthase; PGFS) or through further metabolism of PGE2 via the enzyme PGE 9-ketoreductase. The epimeric form 9α,11β-PGF2 (11-epi-PGF2α) is formed from PGD2 via the PGD 11-ketoreductase (Watanabe 2002, 2012). PGF2α has been found in skin extracts and keratinocytes in vitro (Sugimoto et al. 2006; Ziboh et al. 1977; Rhodes et al. 2009) and has been associated to inflammatory and immune responses, pigmentation and hair growth (Colombe et al. 2007; Scott et al. 2004, 2007). Prostacyclin synthase (PGIS) forms PGI2, a very unstable prostanoid that is readily hydrolysed to the stable but inactive metabolite 6-keto-PGF1α (Cathcart et al. 2010). Finally, thromboxane A synthase (TXAS) converts PGH2 to TXA2, another unstable mediator that is quickly transformed to the stable but inert TXB2 (Cathcart et al. 2010). Both PGI2 and TXB2 have been found in whole skin extracts and cultured skin cells at low concentrations and it is possible that they may derive from infiltrating leukocytes or vascular endothelial cells and not resident skin cells (Pentland and Needleman 1986; Sugimoto et al. 2006).
Prostanoids mediate their activities via a series of G-protein coupled receptors designated EP, DP, FP, IP and TP. They are sub-divided to eight groups as defined by pharmacological studies and related signal transduction pathways, namely: EP1, EP2, EP3, EP4, DP1, FP, IP and TP. However, recent studies have showed the presence of a second PGD receptor, DP2 (also termed CRTh2), as well as a number of receptor heterodimers (Woodward et al. 2012; Breyer et al. 2001). Almost all prostanoid receptors have been identified in skin cells (Scott et al. 2005; Weber et al. 2002; Muller et al. 2000; Black et al. 2008; Andoh et al. 2007; Nakajima et al. 2010; Bryniarski et al. 2008; Konger et al. 2005; Honda et al. 2009; Satoh et al. 2006; Kanda et al. 2010).
Finally, these active autacoids are not stored in cells but produced upon request and exported via a number of PG transporters and the mulitspecific organic anion transporters (Schuster 2002). Prostaglandin levels are actively controlled through oxidation via the NAD + -dependent 15-ketoprostaglandin dehydrogenases (15-PGDH) followed by reduction via the Δ13-15-ketoprostaglandin reductases (Tai et al. 2002). These reactions catalyse the formation of metabolites such as 15-keto-PG and 13,14-dihydro-15-keto PGs that have significantly reduced bioactivities (Tai et al. 2002). 15-PGDH exhibits a rather broad substrate specificity and can deactivate a range of PGs. The PG deactivation system has been identified in epidermal keratinocytes, whole human skin and hair follicles (Rhodes et al. 2009; Judson et al. 2010; Michelet et al. 2008).
Lipoxygenase-Derived Lipid Mediators
Lipoxygenases (LOX) mediate the addition of molecular oxygen in a stereoselective manner, generating a range of hydroperoxy fatty acyls that are reduced to hydroxy fatty acids or further metabolised to leukotrienes (LT; Fig. 4.2). Although LOX isozymes are classified based on their phylogenetic profile, they are conventionally defined by their positional selectivity when oxygenating AA (Brash 1999; Kuhn and O’Donnell 2006). Human and animal skin have been shown to express 5-, 8-, 12- and 15-LOX activities that can catalyse the oxygenation of many PUFA including LA, AA, EPA, DGLA and DHA to produce and array of mono- and poly- hydroxy fatty acids (Miller et al. 1991; Lu et al. 2010; Rhodes et al. 2009; Nicolaou et al. 2012; Mayer et al. 1984).
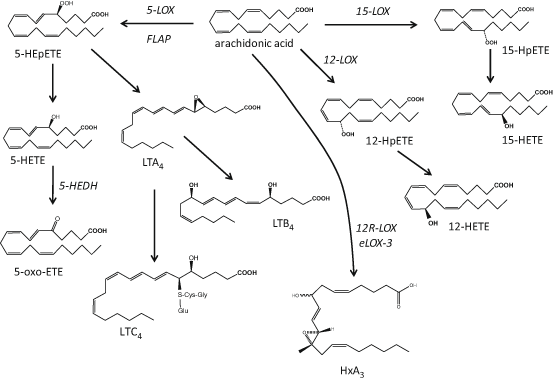
Fig. 4.2
Schematic representation of lipoxygenase-mediated pathways leading to the biosynthesis of hydroxy fatty acid species from arachidonic acid. LOX lipoxygenase, LT leukotriene, HxA 3 hepoxilin A3
The enzyme 5-LOX requires 5-lipoxygenase activating protein (FLAP) for activation; it converts AA to the unstable 5-hydroperoxy eicosatetraenoic acid (HPETE) that is subsequently reduced to 5-hydroxy eicosatetraenoic acid (HETE; Brash 1999). Oxidation of 5-HETE by the enzyme 5-hydroxyeicosanoid dehydrogenase (5-HEDH) produces 5-oxo-eicosatetraenoic acid (ETE; Grant et al. 2009), while dehydration of 5-HETE can generate LTA4 precursor to LTB4 and the cysteinyl leukotrienes LTC4, LTD4 and LTE4 (Brain and Williams 1990). Studies exploring the prevalence of 5-LOX in the skin have suggested that its activity is rather low and related primarily to epidermal keratinocytes in particular during their differentiation (Ziboh et al. 1984; Grabbe et al. 1984a; Janssen-Timmen et al. 1995), Langerhans cells (Doepping et al. 2007; Spanbroek et al. 1998) and infiltrating leukocytes (Serhan et al. 2003), while the formation of cutaneous cysteinyl-leukotrienes has been attributed to infiltrating and not resident skin cells (Church et al. 2002).
12-LOX activity is mediated by a number of isozymes: the cytosolic leukocyte-type 12-LOX and microsomal platelet-type 12-LOX are found in a number of cell types, while the 12R-LOX are unique mammalian skin cells and appear to be important in inflammatory conditions such as psoriasis (Boeglin et al. 1998; Kuhn and O’Donnell 2006). The epidermis-type lipoxygenase-3 (eLOX-3) contributes to the terminal differentiation of keratinocytes and, together with 12R-LOX, is important for the integrity of the epidermal barrier through formation of the epidermis-specific O-linoleoyl-omega-hydroxyceramides and hepoxilins, another AA-derived group of bioactive lipids (Fig. 4.2) (Epp et al. 2007; Brash et al. 2007; Nigam et al. 2007; Zheng et al. 2011).
The activity of 15-LOX is attributed to two isoforms: the reticulocyte-type 15-LOX-1 and the epidermis-type 15-LOX-2. It is noteworthy that 15-LOX-1 has high homology to the leukocyte-type 12-LOX, an isoform presenting both 12- and 15-HETE activities (referred to as 12/15-LOX; Kuhn and O’Donnell 2006). When the substrate is LA, the main product of 15-LOX-1 is 13S-HODE, a metabolite found in high abundance in the skin (Rhodes et al. 2009; Nicolaou et al. 2012). Although mammalian 12- and 15-LOX isozymes oxygenate, primarily, AA and LA, they can also utilise other PUFA including DGLA, EPA and DHA, found in either free or esterified in phospholipids and lipoproteins (Kuhn and O’Donnell 2006; Gron et al. 1993; Heitmann et al. 1995). Recent reports have revealed the presence of a large number of PUFA-derived hydroxy fatty acids in the skin confirming the presence of many cutaneous LOX isozymes, although the exact cellular origin and specific bioactivities of all these mediators are still to be explored (Nicolaou et al. 2012; Rhodes et al. 2009; Lu et al. 2010; McDaniel et al. 2011; Ziboh et al. 2000b; Pilkington et al. 2013; Shappell et al. 2001). Finally, murine epidermis is expressing 8-LOX, a homologue of the inducible 15-LOX-2 that has not yet been found in human skin (Jisaka et al. 1997; Krieg et al. 1998).
LOX reactions are also important in transcellular metabolism generating polyhydroxylated PUFA species with, principally, anti-inflammatory protective biological profiles (Sala et al. 2010; Calder 2009). These LOX-LOX or acetylated-COX-2-LOX or CYP-LOX mediated reactions generate lipoxins (LX) from AA, resolvins (Rv) and protectins (PD) from both EPA and DHA, and maresins (MaR) from DHA (Serhan et al. 2008a). To date, there are no reports on the actual formation of these species in the skin, however, the biological precursors of some of these mediators have been identified (e.g. 15-HETE for LX, 18-HEPE for RvE1 and 17-HDHA for RvD1; McDaniel et al. 2011; Nicolaou et al. 2012; Pilkington et al. 2013).
Cytochrome P450-Derived Lipid Mediators
Although the family of cytochrome P450 (CYP) monooxygenases are better known for their role in detoxification of drugs and xenobiotics, their prevalence in skin suggests potential contribution of PUFA oxygenation (Baron et al. 2008; Neis et al. 2010; Rolsted et al. 2008). CYP isozymes relevant to the formation of eicosanoid-like species are epoxygenases and hydrolases (Oliw 1994): when AA is the substrate, CYP-catalysed epoxygenation can occur in every double bond resulting in four regioisomeric cis-epoxyeicosatrienoic acids (EET), namely: 5,6-, 8,9- 11,12- and 14,15-EET, that can be formed as either R, S or S, R enantiomers. These epoxides are then transformed to biologically inactive dihydroxyeicosatetraenoic acids (DHET) by the enzyme epoxide hydrolase. Mid-chain oxidation of AA can produce a range of HETE including 5-, 8-, 9-, 11-, 12- and 15-HETE similar to the ones formed by LOX, while CYP-catalysed omega-hydroxylations can form 16-, 17-, 18-, 19- and 20-HETE (Spector 2009; Zeldin 2001; Konkel and Schunck 2011; Fig. 4.3). CYP can utilise a range of PUFA including LA, EPA and DHA, to form LA-derived epoxides such as the 9,10- and 12,13-epoxyoctadecamonoenoic acids (EpOME) and HODE, EPA-derived HEPE and five epoxyeicosatetraenoic acids (EEQ), and DHA-derived HDHA and six regioisomeric epoxydocosapentaenoic acids (EDP). Although some of these products could contribute to transcellular biosynthesis of poly-hydroxy PUFA mediators such as RvE and RvD, the presence of the latter has not yet been confirmed in skin (Serhan et al. 2008b; Konkel and Schunck 2011).
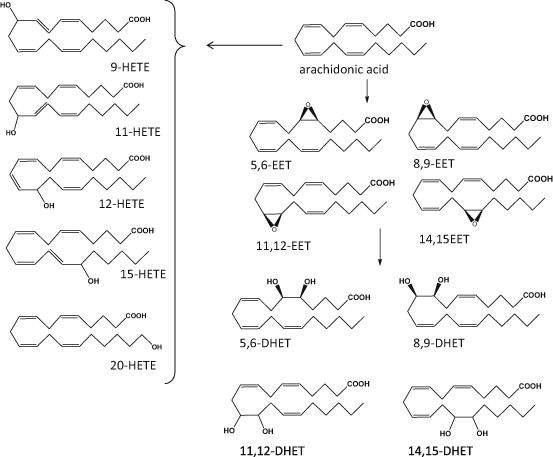
Fig. 4.3
Schematic representation of main cytochrome P450 (CYP) derived mediators of arachidonic acid. HETE hydroxy eicosatetraenoic acid, EET epoxy eicosatetraenoic acid, DHET dihydro eicosatetraenoic acid
Non-enzymatically Produced Oxidised Lipid Mediators
Free radical catalysed oxygenation of PUFA results in lipid mediators with structures similar to the ones produced via the COX-, LOX- or CYP- mediated reactions (Fig. 4.4). However, these oxidised lipids are regio- and/or stereo-isomers of the enzymatically-produced ones, exhibit different and/or less potent bioactivities or are inert, and, in many cases, their presence has been used as marker of local or systemic oxidative stress (Salomon 2005; Grundmann et al. 2004; Niki 2008). One of the most interesting families of oxidised lipids is the group of isoprostanes (isoP) that are racemic diastereoisomers of PGs (Milne et al. 2008). They are formed in situ via the oxidation of PUFA already esterified in phospholipids are released by PLA2. The AA-derived F2-isoP are chemically stable and the plasma and/or urine levels of 8-iso PGF2α are frequently used as biomarker of oxidative stress. Increased levels of this metabolite in UVR-irradiated skin microdialysates and psoriasis patients further support the role of oxidation in cutaneous inflammatory conditions (Grundmann et al. 2004; Wiswedel 2009). Recently, the formation of IsoP products from EPA (F3-isoP; Song et al. 2009) and DHA (F4-isoP or F4-neuroprostanes; Yin et al. 2005) cyclopentenone isoP (Musiek et al. 2006) has been reported, however their prevalence in human skin has not yet been explored.
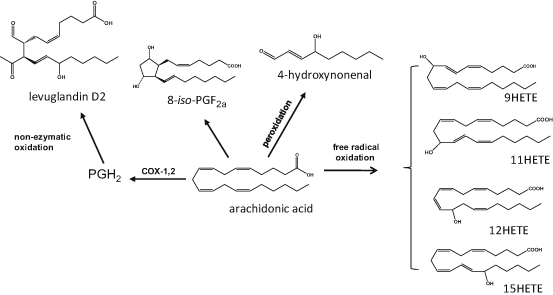
Fig. 4.4
Examples of oxygenated lipid mediators produced during the non-enzymatic oxidation of arachidonic acid. HETE eicosatetraenoic acid, iso–PG isoprostane
Other oxidised PUFA derivatives produced by non-enzymatic reactions include the levuglandins (LG) and isolevuglandins (isoLG), a group of highly reactive keto-aldehydes, which can form toxic protein and nucleic acid adducts, although, to date, there are no reports on their involvement in skin oxidation (Salomon 2005). Lipid peroxidation, a feature of many skin disorders, can also generate highly reactive toxic aldehydes, including 4-hydroxy-2-nonenal (HNE), the main metabolite formed upon peroxidation of n-6PUFA and 4-hydoxy-2-hexanal (HHE), that is produced from n-3PUFA; both HNE and HHE have been used as indices of PUFA peroxidation (Catala 2010; Briganti and Picardo 2003). Studies suggest that HNE can bind and modify proteins in aged skin fibroblasts, while keratinocytes have the ability to process and detoxify this cytotoxic aldehyde (Aldini et al. 2003; Jorgensen et al. 2014). Finally, lipid peroxidation can generate a wide range of HODE, HETE, HEPE an HDHA regio- and stereo-isomers that are formed in a non-selective random manner and may be used as markers of cutaneous disease (Baer et al. 1991; Grundmann et al. 2004).
Bioactivities of Oxidised Lipid Mediators and Role in Inflammatory Skin Disease
Bioactive lipid mediators are produced by skin cells at relatively low concentrations and are used to maintain critical functions related to physiology and homeostasis. However, when produced at higher concentrations and in response to various stimuli, lipid mediators can modulate cell proliferation, inflammation and tumorigenesis, and this is of particular interest to acute and chronic cutaneous inflammatory diseases including atopic dermatitis, psoriasis, photosensitivity disorders, photoageing, sunburn and skin cancer. Nutritional supplementation with PUFA has the potential to alter the cutaneous fatty acid profile and this has been explored as means of developing chemoprotective and therapeutic interventions (Ziboh et al. 2000a; Lee et al. 2003; Nicolaou 2013; Kendall and Nicolaou 2013; De Spirt et al. 2009). These approaches were based on the ability of the fatty acid treatment to reduce the prevalence of AA-derived pro-inflammatory HETE and increase the concentration of less inflammatory derivatives such as 15-HEPE and 15-HETrE (Calder 2006). Examples include animal and human studies using the n-3PUFA ALA, EPA and DHA that reported decreased sensitivity to sunburn and increased immune protection (Orengo et al. 1992; Rhodes et al. 1994, 2003; Pilkington et al. 2013; Takemura et al. 2002). A similar rationale has been used to investigate the role of n-3PUFA-derived mediators in wound healing where the profile of lipid chemo-attractants can influence the leukocytic infiltrate and may affect the degree of epithelization (Lu et al. 2010; McDaniel et al. 2011; Martin and Leibovich 2005; Miller et al. 1991). Furthermore, GLA, DGLA and DHA have showed promising results in improving atopic dermatitis (Gueck et al. 2004; Kawashima et al. 2008), while n-3PUFA supplementation during pregnancy results in fewer children with atopic eczema (Makrides et al. 2013). Finally, GLA, DGLA and EPA supplements, as well as local application and intravenous administration of EPA, have been considered in for the treatment of psoriasis (Zulfakar et al. 2007; Mayser et al. 2002; Ricketts et al. 2011; Miller et al. 1988).
Bioactivity of Cutaneous Prostanoids
Prostaglandins, especially the AA-derived ones, are some of the most abundant cutaneous lipid mediators. PGE2 is produced by both keratinocytes and fibroblasts, and can act as a keratinocyte chemo-attractant with possible involvement in wound healing (Parekh et al. 2009; Ruzicka 1992). Additionally, it contributes to the cross-talk and biochemical support between skin layers (Leong et al. 1996; Sato et al. 1997), while its role on keratinocyte proliferation and differentiation makes it important for the epidermal barrier (Honma et al. 2005; Konger et al. 1998; Pentland and Needleman 1986). Furthermore, PGE2 is involved in epidermal melanocyte biology mediating post-inflammatory pigmentary responses and melanocyte dendricity (Scott et al. 2004, 2007; Gledhill et al. 2010). Overall, PGE2 exhibits pro-inflammatory and vasodilatory activities, induces cellular proliferation, modulates immune suppression and is a strong tumour promoter (Harris et al. 2002; Larsson et al. 2004; Narayanan et al. 2011). Its concentration is increased following UVR exposure, following upregulation of cutaneous PLA2 and COX-2, a major event in photo-carcinogenesis (Tober et al. 2006; Kabashima et al. 2007; Rundhaug and Fischer 2008; Rhodes et al. 2009). UVR appears to also inhibit 15-PGDH in human skin allowing for a short-term increase in PGE2 levels suggesting that higher levels of this eicosanoid may be of importance in the orchestration of cutaneous response to UVR-induced injury (Judson et al. 2010). This role of PGE2 is further supported by studies showing that it can play a part in the resolution of inflammation and tissue repair (Levy et al. 2001), while continuous production of PGE2 in unresolved inflammation may contribute to the immune suppression observed in photo-carcinogenesis (Narayanan et al. 2011).
PGD2 is one of the principle prostanoids involved in atopic dermatitis, and this is linked to the involvement of Langerhans cells and mast cells, that are considered to be the main cutaneous courses of PGD2 (Sugimoto et al. 2006; Matsushima et al. 2011; Kolgen et al. 2002). The vasoactive PGI2 and immunosuppressive PGE2 have also been suggested to be actively involved in this cutaneous condition (Nakajima et al. 2010; Honda et al. 2009). Reduced levels of PGD2 have been reported in animal and human skin post UVR. This has also been linked to the UVR-mediated Langerhans cells migration out of the epidermis that is part of the solar radiation-induced immunosuppression (Ikai et al. 1989; Rhodes et al. 2009; Meunier et al. 1995). Finally, PGD2 is precursor to the anti-inflammatory cyclopentanone prostaglandins PGJ2, Δ12-PGJ2 and 15d-PGJ2 that are formed through non-enzymatic hydrolysis (Straus and Glass 2001; Scher and Pillinger 2009). Although Δ12-PGJ2, a PPARγ agonist, has been shown to exhibit anti-proliferative effects in epidermal cells in vitro, the formation of such potent electrophiles by skin cells has not yet been well documented (Ikai et al. 1995).
Bioactivity of Cutaneous Hydroxy and Epoxy Fatty Acids
Leukotrienes and 5-oxo-ETE are potent chemo-attractants that can contribute to cutaneous inflammation and allergy (Grant et al. 2009; Boyce 2007). However, although the involvement of LTB4 has been shown in animal models of atopic dermatitis (Andoh et al. 2012), it is likely that the origin of 5-LOX-derived mediators can be attributed to dermal neutrophilic infiltrates and not the resident skin cells (Church et al. 2002).
12-HETE is a potent leukocyte chemo-attractant that can also stimulate cell proliferation and enhance tumour cell survival (Dailey and Imming 1999; Honn et al. 1994). Binding sites for 12-HETE have been identified in human keratinocytes and Langerhans cells suggesting its potential involvement in wound healing and cutaneous inflammation (Arenberger et al. 1992, 1993; Ruzicka 1992). Conversely, 15-HETE is considered, primarily, as an anti-inflammatory mediator that can counteract some of the pro-inflammatory properties of 12-HETE and PGE2, and may contribute to the resolution of cutaneous inflammation (Serhan et al. 2003; Vachier et al. 2002; Rhodes et al. 2009). Interestingly, there is a degree of reciprocal regulation between dermal and epidermal 12- and 15-LOX activities and related metabolites, alluding to the complex relationship, cross-talk and biochemical interactions occurring between these skin areas (Kragballe et al. 1986b; Yoo et al. 2008). 15-LOX and its metabolites may also mediate anti-cancer and protective effects similarly to its homologous murine 8-LOX (Schweiger et al. 2007), while 15-HETE may act as metabolic precursor of the anti-inflammatory lipoxins, although the latter have not yet been identified in skin (Serhan et al. 2003). The same anti-inflammatory profile is shared by the 15-LOX-derived LA and DGLA metabolites, 13-HODE and 15-HETrE (Nicolaou et al. 2012; Ziboh et al. 2000b; Xi et al. 2000), while the DHA-derived 14,21-dihydroxy-docosahexaenoic acid (HDHA) has recently been reported to mediate wound healing (Lu et al. 2010). 13-HODE, is one of the most abundant hydroxy fatty acid in the skin and has been shown to reverse epidermal hyperproliferation (Cho and Ziboh 1994). Recent reports have shown a possible involvement of HODE and oxo-ODE in pain, although this has not yet been explored in skin (Ruparel et al. 2012; Sisignano et al. 2013).
UVR upregulates cutaneous 12- and 15-LOX, with concomitant increase of the related hydroxy fatty acids (Ziboh et al. 2000b; Yoo et al. 2008; Rhodes et al. 2009). Interestingly, LOX-derived metabolites appear to peak later than COX-derived prostanoids, suggesting that these two groups of lipids may be important for events related to different phases of the inflammatory response, including initiation and resolution (Rhodes et al. 2009). LOX-mediators are also important in psoriasis that is characterised by high levels of 12-HETE, while chiral analysis revealed the prevalence of 12R-HETE in psoriatic scales pointing at the involvement of 12R-LOX (Boeglin et al. 1998). Furthermore, increased production of PGE2 and PGF2α are less prominent in psoriatic tissue, and 15-HETE appears decreased in uninvolved psoriatic skin compared to healthy epidermis (Grabbe et al. 1984b; Ikai 1999; Kragballe et al. 1986a). Finally, 8, 9- and 11-HETE have also been detected in human skin, although their exact origin has not yet been fully elucidated and may be products of CYP-mediated reactions or non-enzymatic oxidation of AA (Rhodes et al. 2009; Nicolaou et al. 2012). The same applies to the prevalence of 13-(R,S)-HODE in psoriatic skin that is characterised by increased oxidative stress, although the contribution of R-hydroxy-fatty acid producing CYP isoforms cannot be excluded (Zhou et al. 2009; Baer et al. 1990).
Although some AA-specific mono-oxygenases have been identified in epidermal keratinocytes (Keeney et al. 1998a, 1998b), the overall contribution of CYP in cutaneous eicosanoid production remains to be explored. CYP-derived eicosanoids include various EET that are involved in vascular relaxation and angiogenesis, their biologically inactive DHET metabolites, various mid-chain HETE (e.g. 8-,9-,11-,12-,15-HETE), and the omega-hydroxylated 20-HETE, a potent vasoconstrictor (Spector 2009; Kroetz and Xu 2005; Panigrahy et al. 2010; Iliff et al. 2010). EET have been identified in mouse skin cells and 11,12- and 14,15-EET have been shown to play a part in the cornification of human and mouse keratinocytes (Du et al. 2005; Ladd et al. 2003). It has been reported that UVR upregulates CYP4A11 in human keratinocytes, however, the effect of UVR on the activity and expression of cutaneous CYP450 isoforms is not well documented (Gonzalez et al. 2001). Finally, it is of interest to note that CYP isozymes can mediate the oxidation of eicosanoids, as suggested by the co-localisation of COX-2, mPGES-1 and CYP4F8 in psoriatic lesions leading to oxidation of PGE2 (Stark et al. 2006).
Acknowledgements
Research support provided by the The Wellcome Trust (WT094028) is gratefully acknowledged. We thank Dr. Paula Urquhart for her help to compose the figures shown in the manuscript.
References
Aldini G, Granata P, Orioli M, Santaniello E, Carini M. Detoxification of 4-hydroxynonenal (HNE) in keratinocytes: characterization of conjugated metabolites by liquid chromatography/electrospray ionization tandem mass spectrometry. J Mass Spectrom. 2003;38:1160–8.PubMed
Andersen S, Sjursen W, Laegreid A, Volden G, Johansen B. Elevated expression of human nonpancreatic phospholipase A2 in psoriatic tissue. Inflammation. 1994;18:1–12.PubMed
Andoh T, Nishikawa Y, Yamaguchi-Miyamoto T, Nojima H, Narumiya S, Kuraishi Y. Thromboxane A2 induces itch-associated responses through TP receptors in the skin in mice. J Invest Dermatol. 2007;127:2042–7.PubMed
Andoh T, Haza S, Saito A, Kuraishi Y. Involvement of leukotriene B4 in spontaneous itch-related behaviour in NC mice with atopic dermatitis-like skin lesions. Exp Dermatol. 2012;20:894–8.
Arenberger P, Kemeny L, Rupec R, Bieber T, Ruzicka T. Langerhans cells of the human skin possess high-affinity 12(S)-hydroxyeicosa tetraenoic acid receptors. Eur J Immunol. 1992;22:2469–72.PubMed
Arenberger P, Kemeny L, Ruzicka T. Characterization of high-affinity 12(S)-hydroxyeicosatetraenoic acid (12(S)-HETE) binding sites on normal human keratinocytes. Epithelial Cell Biol. 1993;2:1–6.PubMed
Baer AN, Costello PB, Green FA. Free and esterified 13(R, S)-hydroxyoctadecadienoic acids: principal oxygenase products in psoriatic skin scales. J Lipid Res. 1990;31:125–30.PubMed
Baer AN, Costello PB, Green FA. Stereospecificity of the products of the fatty acid oxygenases derived from psoriatic scales. J Lipid Res. 1991;32:341–7.PubMed
Baron JM, Wiederholt T, Heise R, Merk HF, Bickers DR. Expression and function of cytochrome p450-dependent enzymes in human skin cells. Curr Med Chem. 2008;15:2258–64.PubMed
Bergers M, Van de Kerkhof PC, Happle R, Mier PD. Membrane-bound phospholipase C activity in normal and psoriatic epidermis. Acta Derm Venereol. 1990;70:57–9.PubMed
Bito T, Nishigori C. Impact of reactive oxygen species on keratinocyte signaling pathways. J Dermatol Sci. 2012;68:3–8.PubMed
Black AK, Greaves MW, Hensby CN, Plummer NA. Increased prostaglandins E2 and F2alpha in human skin at 6 and 24 h after ultraviolet B irradiation (290–320 nm). Br J Clin Pharmacol. 1978;5:431–6.PubMed
Black AT, Gray JP, Shakarjian MP, Mishin V, Laskin DL, Heck DE, Laskin JD. UVB light upregulates prostaglandin synthases and prostaglandin receptors in mouse keratinocytes. Toxicol Appl Pharmacol. 2008;232:14–24.PubMedCentralPubMed
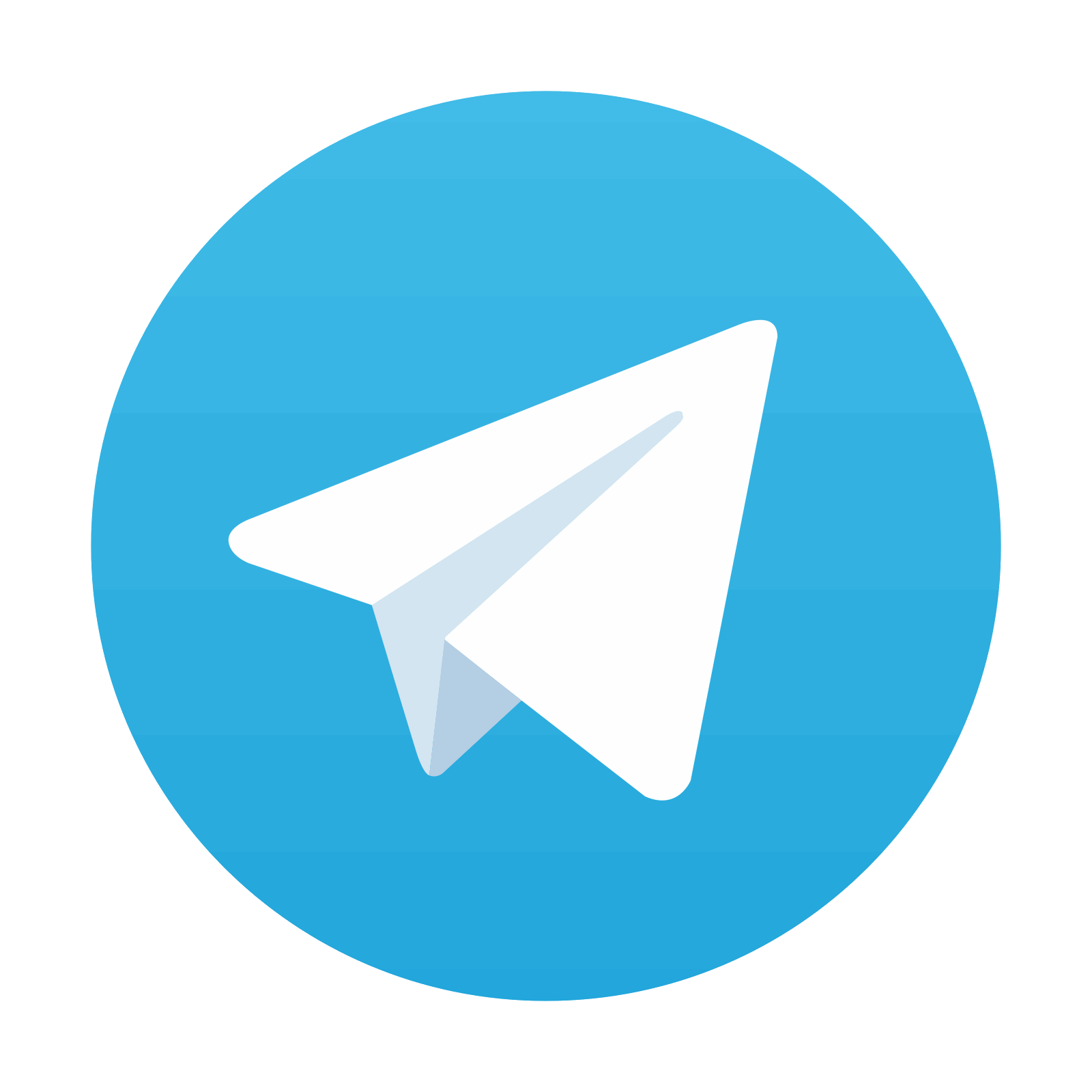
Stay updated, free articles. Join our Telegram channel

Full access? Get Clinical Tree
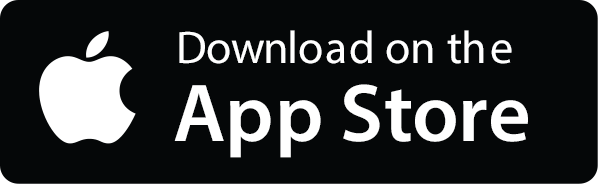
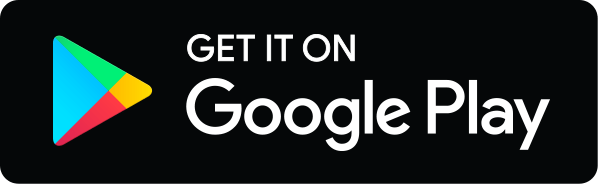