17 Biocompatibility and Biodurability Biomechanical Assessment and Fatigue and Extrusion Characteristics of the NUBAC Nucleus Implant For diskogenic back pain, fusion is no longer being considered the gold standard of treatment due to the concern of adjacent disk disease. After more than 40 years of relentless effort, disk arthroplasty is finally becoming a clinically viable option for the treatment of diskogenic back pain. Disk arthroplasty can be divided into total disk replacement and nucleus replacement. Although the goals of these two arthroplasty treatments are the same (i.e., relieving back pain through removing the pain source and restoring or maintaining disk anatomy and function), each approaches the problem from a different perspective. For total disk replacement, almost all of the disk tissue is removed and, therefore, functional restoration depends completely on the device itself. However, for a nucleus replacement, only the nucleus is removed and functional restoration relies on the device and the remaining annulus and ligaments. Although total disk technology has been narrowed to two basic designs (ball and socket and metal-elastomer-metal), there are more variations in nucleus designs, from metal ball to in situ curable elastomer. To design an effective prosthesis, it is important to understand the functions played by the original tissue and the prosthesis. Biologically, the nucleus pulposus functions as a fluid pump that facilitates fluid diffusion, carrying nutrients to and removing metabolites from the avascular disk.1 Biomechanically, although the nucleus presents as a loose gel and has little resistance to physiological loads by itself, it inflates the annulus and shares about one half to two thirds of the compressive load on the disk.2 In most nucleus replacements, because the majority of the natural nucleus is replaced with a nonbiological material, the biological function of fluid diffusion might no longer be important for the implanted disk, although conceptually it might still bring the benefit of preventing degeneration of the remaining nucleus and annulus. Therefore, for most nonbiological nucleus devices, the main objective would be to restore or maintain the disk height, sagittal balance, and bio-mechanical function of the index disk. Many past and current nucleus replacements are made of elastic materials, either preformed or in situ cured, probably due to the viscoelastic nature of the nucleus. However, there is a major limitation in using a material that attempts to mimic the exact properties of the natural nucleus for a nucleus replacement device because of the change in boundary conditions. As mentioned earlier, the mechanical function of the nucleus is largely achieved by the total encasement and resulting constraint of the annulus, just like air is encased and constrained by a tire. If there is a rupture in the annulus, the nucleus will immediately leak through this defect with a drastic reduction of intradiskal pressure which compromises its load-sharing capability. Before the technology of repairing the annulus becomes available, a nucleus prosthesis will always be used with some annular defect, which can either exist prior to the surgery or be created during the surgery. Therefore, most elastic nucleus replacement devices use materials with a modulus much higher than the natural nucleus so they will not deform easily and can be readily extruded through the annular defect. Several nonelastic nucleus devices have also been attempted. In fact, the two nucleus implants first used in humans were made of nonelastic materials. The first nucleus implant used in a human was polymethyl methacry-late (PMMA), which is a plastic material. The clinical data on this device are limited. Although the initial data on 14 patients appeared to be encouraging,3 a subsequent small randomized study using diskectomy as a control found that the PMMA nucleus device provided no obvious clinical advantages over the control.4 The second nucleus implant used in a human was the Fernström ball, a stainless steel ball bearing, which was even stiffer than PMMA. Although the Fernström ball design seems simple, the concept showed clinical evidence of being fairly sound. Based on biomechanical data that suggested the plane of intervertebral movement could be described by the movement of a contiguous vertebra over a small ball located in the nucleus pulposus recess,5 Fernström believed a ball nucleus device would best restore the articulation of the adjacent vertebrae and prevent anterior or posterior slippage of the neighboring vertebrae. The Fernström ball has been implanted in many more patients than the PMMA nucleus, and all the published clinical data on this device appeared to be fairly favorable. Fernström reported his own series of a total of 125 patients with 191 nucleus implants with a follow-up of 6 months to If one is to judge the clinical success of the Fernström Ball from the published data, the Fernström Ball should be a clinically viable device, even compared with the clinical results of many other modern disk arthroplasty devices.9–11 However, for whatever reasons, the Fernström Ball was not further commercialized beyond its initial use in the 1960s. Most of the negative criticism on the Fernström nucleus device has been focused on its subsidence. From the design of the Fernström Ball, it is obvious that some subsidence is inevitable due to the initial small or nearly point contact area, resulting in a high initial contact stress. As a matter of fact, some initial subsidence was the intention of Fernström. Fernström instructed users to utilize an implant at least 1 mm taller than the disk height.6 The initial subsidence seated the implant in the disk space and prevented the implant from extrusion in almost all cases. Most subsidence for the Fernström Ball stopped after 1 to 3 mm per end plate.6 The design of the Pioneer Surgical Technology (PST) (Marquette, MI) NUBAC nucleus device was largely based on the lessons learned from other previous nucleus devices, with the intention to capture the features that contributed to the success of previous devices and to address pitfalls that existed in the previous devices. From the clinical experience of the Fernström Ball, it is clear that a nonelastic nucleus device design works well in relieving diskogenic back pain and maintaining the index disk anatomy and function. It is more encouraging to note that the effectiveness in relieving diskogenic back pain was maintained over a long time with almost no major safety issue. The Fernström Ball forms two articulating surfaces with the adjacent vertebrae and allows the rotational motion of the index disk, which has become a key design requirement for any disk arthroplasty device. The major deficiency of the Fernström nucleus is the small initial contact area, which inevitably led to some degree of subsidence. The PST NUBAC is a two-piece implant consisting of a top and a bottom plate, with a ball and socket inner articulation joint allowing rotational motions along all three major axes within the physiological ranges. The motion of the device in axial rotation is unconstrained, whereas the motions in flexion-extension and lateral bending are semiconstrained slightly beyond the physiological ranges of motion in these directions. Unlike a total disk, the NUBAC device’s range of motion is largely dependent upon the constraints from the adjacent tissues. The device is designed to restore or maintain the load-sharing capabilities of the index disk. By selecting the proper wedge angle of the device it can restore the normal sagittal balance. There are several size configurations to ensure that individual differences in anatomy can be accommodated. Both the top and bottom plates are in the shape of a racetrack, similar to the shape of a typical nucleus cavity. This will ensure sufficient implant/cavity conformity and adequate stress distribution on the end plates. The outer surfaces of the implant are either flat or slightly convex to approximate the flat or slight concavity of the end plates (refs).12 The device is manufactured from polyetheretherketone (PEEK), an exceptionally strong engineering thermoplastic. The elastic modulus of PEEK is close to that of the underlying bone. The device is radiolucent and will not interfere with any images. It is more important for a nucleus device than a total disk device to be radiolucent so it does not interfere magnetic resonance imaging (MRI) for evaluation of potential pathology at the index disk. Tantalum markers are used to facilitate the visualization of implant location using x-ray radiography. PEEK is one of the top medical thermoplastics with a well-documented biocompatibility and biostability history, along with an excellent track record of being used as a permanent implant, including spinal implants.13 Biostability is extremely important for load-bearing disk arthroplasty devices because of the long life expectation for the device. In general, the biostability for biomaterials follows the order of metal > plastic > elastomer. Biodegradation of a biomaterial can be affected by many different factors, such as stress, biological environment, and oxidation, and may be difficult to evaluate using in vitro studies. For example, the Acroflex (DePuy-Acromed, Inc., Raynham, MA) total disk replacement device, which has a hexane-based polyolefin elastomer core, had passed vigorous in vitro fatigue tests under various compression and shear conditions.14 However, in a pilot study of 28 patients, fracture on the elastomer core was found in 10 out of 28 implants within 2 years.15 It is likely this failure was due to the biodegradation occurring in the body, which was not assessed during the fatigue test. Therefore, unless the biomaterial has an established clinical history of biostability, there will always be some uncertainties on the biostability of the material for disk arthroplasty applications, especially for elastomers. The chemical structure of PEEK ensures extreme stability against hydrolysis, even at elevated temperature. PEEK can be steam or gamma sterilized repeatedly without deterioration in its bulk mechanical properties. Figure 17–1 Schematic drawings of contact stress distribution. (A) Initial contact stress of the Fernström Ball. (B) Contact stress of the Fernström ball after some subsidence. (C) Contact stress of the NUBAC™ nucleus implant. As reported by Fernström, most of the subsidence related to the Fernström Ball stopped after 1 to 3 mm per end plate.6 This phenomenon can easily be explained with the contact stress and the ultimate compressive strength (UCS) of the end plate. If the initial contact stress is above the UCS of the end plate, the implant will experience subsidence and lead to an increase in the contact area, which in turn leads to a decrease in contact stress. The subsidence will eventually stop when the contact stress is below the UCS. Lowe et al reported that the static end plate UCS was around 17 to 20 MPa for lumbar vertebrae when compressed with a circular indenter.16 However, Tan et al, in a more recent study, found that the UCS was only ~2.7 MPa when compressed with an elliptical indenter.17 The low UCS reported by Tan et al is likely due to the much older samples (mean age of 77 years) used in this study than the samples (age range, 40–50 years) used in Lowe et al’s study. It is likely that the vertebral fatigue fracture strength will be less than the static UCS. When the Fernström Ball nucleus was first implanted, it had a near point contact with the end plates (Fig. 17–1A). This point contact leads to a contact stress much higher than the UCS of the end plate and, therefore, inevitably causes the implant subsiding into the end plate. As the Fernström ball subsides, the contact area increases and the contact stress decreases. The subsidence eventually stops as the contact area increases to a point at which the contact stress gets to below the UCS. To better understand the contact area and contact stress between the NUBAC artificial nucleus and the Fernström Ball (range, 10–16 mm diameter), the calculations in Table 17–1 and Fig. 17–1
Pioneer Surgical Technology
NUBAC Artificial Nucleus
Pioneer Surgical Technology NUBAC Nucleus Device Design Rationale
Summary of the NUBAC Preclinical Studies
NUBAC Nucleus Implant Indication and Surgical Technique
years.6 He divided his patients into two groups; group 1 with disk herniation and group 2 with only diskogenic back pain. For comparison purposes, he also analyzed 100 control patients with similar indications who were treated with diskectomy only. He found that low back pain occurred in only 12% of group 1 patients, which compared very favorably with the control group of 60%. For patients with only diskogenic back pain (group 2), low back pain occurred in 40% of patients, which also compared favorably with the 88% occurrence rate in the control group. Roentgen examination performed on 30 lumbar implanted segments every 6 months for
years revealed preservation of bending motion in the index segment. In a separate study, McKenzie implanted a total of 155 Fernström Balls in 103 patients. He also divided his patients into two groups based on the indications in the same way as Fernström. In a short-term study of 40 patients, half of whom had acute disk herniation and half of whom had disabling degenerative disk disease, he reported excellent and good results in 85% of patients.7 Many years later, McKenzie reported the long-term follow-up data with an average follow-up time of 17 years on 69 of his 103 patients.8 Results in group 1 (herniated disk) patients and group 2 (diskogenic back pain only) in the long-term series were graded excellent and good in 83% and 75%, respectively. Ninety-five percent of all patients returned to work. McKenzie’s results, even by today’s standards, would be considered a success. Like almost any clinical study, there were certainly some limitations with these studies. Foremost, it can be argued that these were retrospective, nonrandomized, controlled studies. However, it should be realized that the vast majority of clinical studies on spinal implants, especially for those started in the 1960s, were retrospective, nonrandomized, and controlled.
Pioneer Surgical Technology NUBAC Nucleus Device Design Rationale
Stay updated, free articles. Join our Telegram channel

Full access? Get Clinical Tree
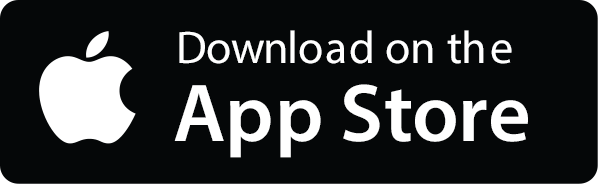
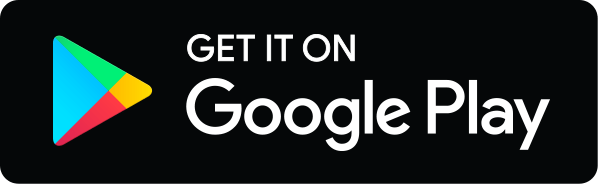