Fig. 19.1
Optical microscopy picture of an o/w Pickering emulsion of silicone oil stabilized by zinc oxide (ZnO) particles. Addition of a large concentration of sodium chloride (NaCl, 1 mol·L−1) caused the partial flocculation of ZnO particles into large aggregates that could be observed at the surface of oil droplets by optical microscopy. The oil droplets are covered by a dense coating of ZnO particles over the brown areas where large aggregates are also visible. The white areas are bare. The picture also shows free ZnO particles dispersed in the aqueous phase which are seen as aggregates in between the oil droplets
The name “Pickering emulsion” was given after their early disclosure by S.U. Pickering (1907). Actually, the adsorption of solid particles at the air-water interface has been reported earlier (Ramsden 1903). However, the merit has been given to S.U. Pickering, because his paper specifically dealt with emulsions stabilized by solid particles; he reported improved stability for these emulsions with respect to surfactant-based emulsions and provided a definite proof for the adsorption of solid particles, being the origin for such stabilization.
Pickering emulsions are essentially emulsions, so that their main properties are common with classical emulsions stabilized by surfactants (emulsifiers). Pickering emulsions are prepared using the same manufacturing processes as for classical emulsions. There might be a specific process for Pickering emulsification; however, the most often used preparation methods are the same as for classical emulsions. As a consequence, the application domains of Pickering emulsions are the same as for classical emulsions, and an application based on classical emulsions can easily be switched to Pickering emulsion. Pickering emulsions show some improved properties compared to classical emulsions. Adsorbed solid particles act as a more effective barrier against coalescence than surfactants. Such important benefit opens the possible stabilization of coarse emulsions and multiple emulsions. The “surfactant-free” character makes them attractive to applications to life sciences where surfactants often cause either irritancy or hemolysis.
Applications of Pickering emulsions in pharmaceutical and cosmetic fields rely on either their specific properties manifested in vivo (low irritancy related the surfactant-free character, specific interactions with biological interfaces) or the development of new dosage forms having improved ex vivo properties (emulsion stability, thickening with no polymeric thickener) over surfactant-based emulsions. The present chapter is dealing with percutaneous penetration-enhancing properties of Pickering emulsions and covers the former aspect where drug-loaded Pickering emulsions modify the transport of drug molecules into/through skin. This includes the classical “penetration enhancer” action similar to that of “penetration enhancer” chemicals and also any modification of the drug transport induced by the formulation in a Pickering emulsion. The behavior of Pickering emulsions is discussed with respect to a reference surfactant-based emulsion.
The physicochemical properties of Pickering emulsions are shortly reviewed in the first part of the chapter. The second part reports on the skin absorption behavior of drugs followed by a discussion of the mechanism of the effects induced by Pickering emulsions compared to surfactant-based emulsions.
19.2 Physical Chemistry of Pickering Emulsions
The origin of emulsion stabilization is the adsorption of solid particles at the surface of emulsion droplets. The mechanism of adsorption is partial wetting of the particles by oil and water, which is very different of surfactants; the solid particles are not amphiphilic. Stabilization takes place by preventing destabilization events: coagulation, coalescence, and Ostwald ripening. There are strong similarities with classical surfactant-based emulsions; and there are few differences that provide specificities to Pickering emulsions. The manufacturing processes of Pickering emulsions used so far are the same as those of classical emulsions.
19.2.1 Adsorption of Solid Particles at Interfaces and Stabilization of Emulsions
Particles adsorbed at the oil-water interface are wet both by oil and water. Wetting conditions depend on interfacial tensions of the solid-water, solid-oil, and oil-water interfaces, γ s-w, γ s-o, and γo-w. Under partial wetting conditions, the contact angle in water, θ w, is given by the Young’s law:

Partial wetting is quite common. Complete wetting by water occurs for very hydrophilic surfaces; such particles remain dispersed in the aqueous phase. This is the case of silica that cannot be used as stabilizing particle for most common oils (Frelichowska et al. 2009a). Silica is very often used however because there are commercially available grades of “hydrophobized” silica having organosilanes chemically grafted to their surface (Barthel 1995). Wetting of such silica surfaces is controlled by the grafting degree of the organosilane. Adsorption of organic molecules such as surfactants, polymers, or even small surfactant-like organic molecules, to the solid particles, allows adjusting the surface properties of the stabilizing particles so as to achieve the desired emulsion type and optimum stability (Gelot et al. 1984; Hassander et al. 1989; Midmore 1998a, b, 1999; Ghouchi Eskandar et al. 2007, 2011; Akartuna et al. 2009; Drelich et al. 2010). However, surface-active molecules that adsorb at the surface of solid particles may also adsorb at the oil-water interface and contribute to the emulsion stability in the same way as classical surfactants do. Adsorption of solid particles is very strong when partial wetting conditions are met. The strongest adsorption and the maximum stability of emulsions are reached when the contact angle is 90° (Binks and Lumsdon 2000a). Obviously, large particles having a larger area contacting oil and water show larger adsorption strength. Even small solid nanoparticles also adsorb to the oil-water interface quite strongly. Stabilizing solid particles do not show surface activity, which is a definite difference compared to surfactants (Vignati et al. 2003; Dong and Johnson 2003).

(19.1)
Stabilization of Pickering emulsions occurs because the layer of adsorbed solid particles forms a rigid coating that acts as a mechanical barrier against coalescence. Such a rigid protective coating has been compared to an eggshell. The origin of its mechanical strength is the two-dimensional aggregation of solid particles at the droplet surface by means of capillary forces. A supplementary three-dimensional aggregation takes place in some instances, building a thick solid layer of solid particles. Therefore, the stability of Pickering emulsions is very high compared to classical emulsions. It allows the preparation of either concentrated emulsions (high internal phase ratio) or coarse emulsions (emulsion with droplet size in the millimeter range) that conventional surfactants would not be able to stabilize with sufficient efficiency. Solid particles may also prevent coagulation if they cause thickening of the continuous phase. This effect is similar to the stabilizing action of polymeric thickeners that are often included in the formulation of conventional emulsions.
Numerous particles are able to stabilize Pickering emulsions. They are either inorganic or organic. Hydrophobized silica and clay (montmorillonite, laponite, kaolin) are the most common inorganic particles. Latex and carbohydrate nanocrystals (cellulose, chitin) are examples of suitable organic particles.
19.2.2 Emulsion Type, Emulsion Inversion, and Double Emulsions
The emulsion type is controlled by the wettability of the solid particles (Aveyard et al. 2003; Binks and Lumsdon 2000): hydrophilic particles stabilize o/w emulsions and hydrophobic particles stabilize w/o emulsions. Examples of hydrophilic particles are silica, clay, titanium dioxide, and zinc oxide. Examples of hydrophobic particles are “hydrophobic” silica (grafted with organic silanes), “hydrophobic bentonite” (montmorillonite coated with fatty quaternary ammonium salts) polystyrene, and polytetrafluoroethylene (PTFE, Teflon®). Too much hydrophilic (or hydrophobic) particles are totally wet by water (or oil) however; partial wetting is an absolute requirement for solid particles adsorption at the oil-water interface. This is very similar to the Bancroft rule for emulsifiers stating that a hydrophilic emulsifier (high HLB) gives rise to an o/w emulsion type, while a hydrophobic emulsifier (low HLB) gives rise to a w/o emulsion type. Accordingly, a HLB-like rule has been introduced for solid particles by Kruglyakov (2000). The relevant quantity is the contact angle in water, θ w, defined by the Young’s law (Eq. 19.1): o/w emulsions form when θ w <90°, and w/o emulsions form when θ w >90°. In contrast with the behavior of emulsifiers, the contact angle for optimum stability is close to θ w = 90°. Therefore, “balanced particles” are efficient stabilizers, whereas “balanced surfactants” behave as poor emulsifiers. As an example, the well-known lecithin is a “balanced surfactant” that cannot stabilize emulsions; it needs being mixed with either a hydrophilic surfactant in order to stabilize o/w emulsions or with a hydrophobic surfactant for o/w emulsions. In other words, optimum emulsifiers are shifted apart from the HLB of “balanced surfactants”; conversely, “balanced particles” are at optimum. As a corollary, the same type of solid particles meets the stabilization criteria of either o/w or w/o emulsion type. The Bancroft rule for solid particles is less a clear-cut rule than for emulsifiers. Phase inversion does not take place when the contact angle is close to 90°. Catastrophic phase inversion upon progressive addition of the continuous phase does not happen as readily as for surfactants. Apart from the wetting behavior, supplementary parameters such as the relative water and oil contents, the medium where the solid particles have been initially dispersed, have a definite influence on the emulsion type (Binks and Lumsdon 2000a, b, c; Binks and Rodrigues 2003). Another consequence of both o/w and w/o emulsion types being at optimum for θ w = 90° is a strong hysteresis in phase inversion experiments (Binks and Rodrigues 2003; Kruglyakov and Nushtayeva 2004).
Multiple emulsions of the w/o/w type are attractive dosage forms for encapsulation of hydrophilic drugs such as proteins and nucleic acids. The stabilization of multiple emulsions using emulsifiers is a difficult task because the stability is lost when the emulsifiers adsorbed at the surfaces of the w/o internal and w/o/w droplets mix together. The strong adsorption of solid particles to the various oil-water interfaces is an obvious benefit because particles keep retained at the right interfaces and do not mix.
A classical two-step process was used to prepare w/o/w multiple emulsions of medium-chain triglycerides: a primary w/o emulsion was stabilized by hydrophobic silica particles (coated with grafted dimethylsilyl groups at 49 % coverage); the obtained primary w/o emulsion was then dispersed in water using hydrophilic silica particles (coated with dimethylsilyl groups at 21 % coverage) as stabilizing particles, yielding a stable w/o/w emulsion (Barthel et al. 2003).
19.2.3 Control of Emulsion Properties by Formulation and Process Parameters
Once the emulsion type and the nature of the ingredients have been chosen, the droplet size and the rheological behavior are the main physicochemical properties of emulsions that matter in regard to their skin delivery. Such properties are controlled by the formulation (the type and concentration of the ingredients) and the emulsification process.
19.2.3.1 Droplet Size of Pickering Emulsions
The concentration of solid particles controls the droplet size. This meets expectations as it is similar to the effect of the concentration of surfactants. Indeed, a larger amount of stabilizing species allows a larger interfacial area to be formed, thus smaller droplets. Under conditions such that solid particles adsorb as a dense monolayer, the total oil-water interfacial area is in proportion to the amount of solid particles, and the mean droplet diameter is given by the following relationship (for an o/w emulsion) based on simple geometrical considerations (Wiley 1954; Arditty et al. 2003; Frelichowska et al. 2010):

where M(oil)/M(solid) is the mass ratio of oil (dispersed phase) to solid particles, ρ oil is the density of oil (kg m−3), and a solid is the interfacial area covered per mass of adsorbed solid particles (m2 kg−1). Such a linear relationship has often been experimentally verified (Arditty et al. 2004, 2005); but cases of departure from Eq. 19.2 have also been reported (Wang and Hobbie 2003; Binks and Whitby 2004; Frelichowska et al. 2010). Incomplete coverage by solid particles may also lead to stable Pickering emulsions; however, there are several microscopic observations of such emulsions (Fig. 19.1) (Binks and Kirkland 2002; Horozov and Binks 2006; Destribats et al. 2010).

(19.2)
The mechanisms being responsible for the droplet size in surfactant-based and Pickering emulsions are different. The reduction of droplet size by increasing surfactant concentration results from two phenomena: on one hand, the simple geometrical argument given above holds; on the other hand, fast surfactant adsorption during the emulsification process causes a decrease of the interfacial tension that makes the droplets fragmentation easier under shear. On the contrary, adsorption of solid particles does not change the interfacial tension (Vignati et al. 2003; Dong and Johnson 2003) (unless the particles contain surface-active impurities or they are associated with a surfactant).
In the case of a low amount of solid particles, a “limited coalescence” ripening phenomenon may occur: the poorly stabilized emulsion droplets undergo coalescence till the total interfacial area matches the area that the solid particles can stabilize (Wiley 1954; Arditty et al. 2004). Successful limited coalescence requires that the emulsion remains stable during the ripening process; otherwise emulsification fails as a part of the oil is released (Frelichowska et al. 2010; Avendaño Juárez and Whitby 2012). Under conditions of low particle content, large droplets can only be stabilized. A suitable emulsification process yields stable coarse emulsions having droplets within millimeter size range (Zhai and Efrima 1996; Arditty 2003). Formation of poorly stabilized Pickering emulsions is controlled by both formulation and emulsification process parameters.
On the basis of the geometrical relationship between droplet diameter and oil/solid mass ratio (Eq. 19.2), very fine emulsions might be prepared at a high content of solid particles. Such expectation is based on the hypothesis of droplet-size control by the amount of stabilizing particles. A high-power emulsification process is required for the fragmentation of droplets. In the case of lack of power, the smallest droplets that the process can create do form, and only a part of the stabilizing particle is used for reaching full coverage of the droplets. The remaining part of the stabilizing particles is not used for the stabilization; such excess particles are left free in the continuous phase.
As a summary, three distinct regimes are encountered depending on the mass ratio of solid particles to the dispersed phase (Fig. 19.2). In the first regime at low solid content, either emulsification fails or coarse emulsions are prepared provided a suitable process has been designed. In the second regime, the droplet size is controlled by the ratio M(particles)/M(oil) according to Eq. 19.2. The third regime is reached when the emulsification process is not able to create the interfacial area that the amount of solid particle might stabilize. The droplet size gets controlled by the emulsification process parameters such as homogenizer type, design of the instruments (propeller, rotor-stator, etc.), and stirring speed. The crossover from the second to the third regime depends on the power of the emulsification process.


Fig. 19.2
Scheme of the different behaviors taking place according to the mass ratio M(particles)/M(oil). In the first regime at low particle/oil ratio, either emulsification process fails or a stable coarse emulsion is formed. Full adsorption of solid particles takes place in the second regime where the droplet size is controlled by the formulation (amounts of solid particles and oil). The droplet size is controlled by the emulsification process in the third regime at high particle/oil ratio; excess solid particles is dispersed in the aqueous phase and possibly particles aggregate, forming a percolating continuous network in case of strong aggregation or large amount of excess particles
19.2.3.2 Rheology of Pickering Emulsions
The viscosity of emulsions is an important property regarding their use as formulations for topical administration. Indeed it controls the materials transfer from the emulsion droplets to the skin. When all solid stabilizing particles are adsorbed to the emulsion droplets, the rheological behavior of Pickering emulsions is the same as that of surfactant-based emulsions. Emulsions are fluid as long as the concentration of droplets remains low; strong thickening appears in concentrated emulsions when the volume fraction of droplets reaches 50–60 %. High internal phase ratio emulsions (HIPEs, gel emulsions) are viscoelastic materials found for concentrations above 60–70 %. A specific feature of Pickering emulsions is the thickening action of excess of free particles (non-adsorbed) present in the aqueous phase (of o/w emulsions). In general, the solid particles that are able to adsorb onto oil droplets also tend to self-aggregate in water because they are not hydrophilic enough to remain well dispersed. As an example, the hydrophobic fumed silica particles used for the stabilization of Pickering emulsions have been primarily made commercially available as thickening agents (Barthel 1995).
A nice example of gelation induced by solid particles in Pickering emulsions is given by Abend and Lagaly (1998, 2001). A percolating network of flocculated solid particles has been built by heterocoagulation of two types of particles. The adsorbed particles prevented coalescence; the remaining part caused thickening and prevented coagulation. Interestingly, X-ray microscopy observations (Fig. 19.3) have shown that the oil droplets coated by solid particles were stuck to the network of solid particles, so that the oil droplets were part of the gel (Neuhäusler et al. 1999; Thieme et al. 1999).


Fig. 19.3
X-ray microscopy images of o/w Pickering emulsion stabilized by mixed montmorillonite and layered double hydroxide particles that underwent heterocoagulation. A continuous network of aggregated particles is seen in the continuous phase, as well as entrapped oil droplets appearing as large black circles. Montmorillonite particles appear as platelets and layered double hydroxide appear as particles of nearly spherical shape (Thieme et al. (1999), with permission)
The flocculation of excess solid particles can be induced in a controlled manner by physicochemical parameters such as salinity of the aqueous phase. Thus, low salinity leave well-dispersed or weakly flocculated particles and the emulsion is fluid (either Newtonian or rheo-thinning fluid); high salinity induces the formation of a continuous network of solid particles in the continuous phase that causes gelation of the emulsion. The rheological properties of practical relevance, in particular the yield stress, can be adjusted by means of simple addition of electrolytes (Horozov et al. 2007; Whitby et al. 2011).
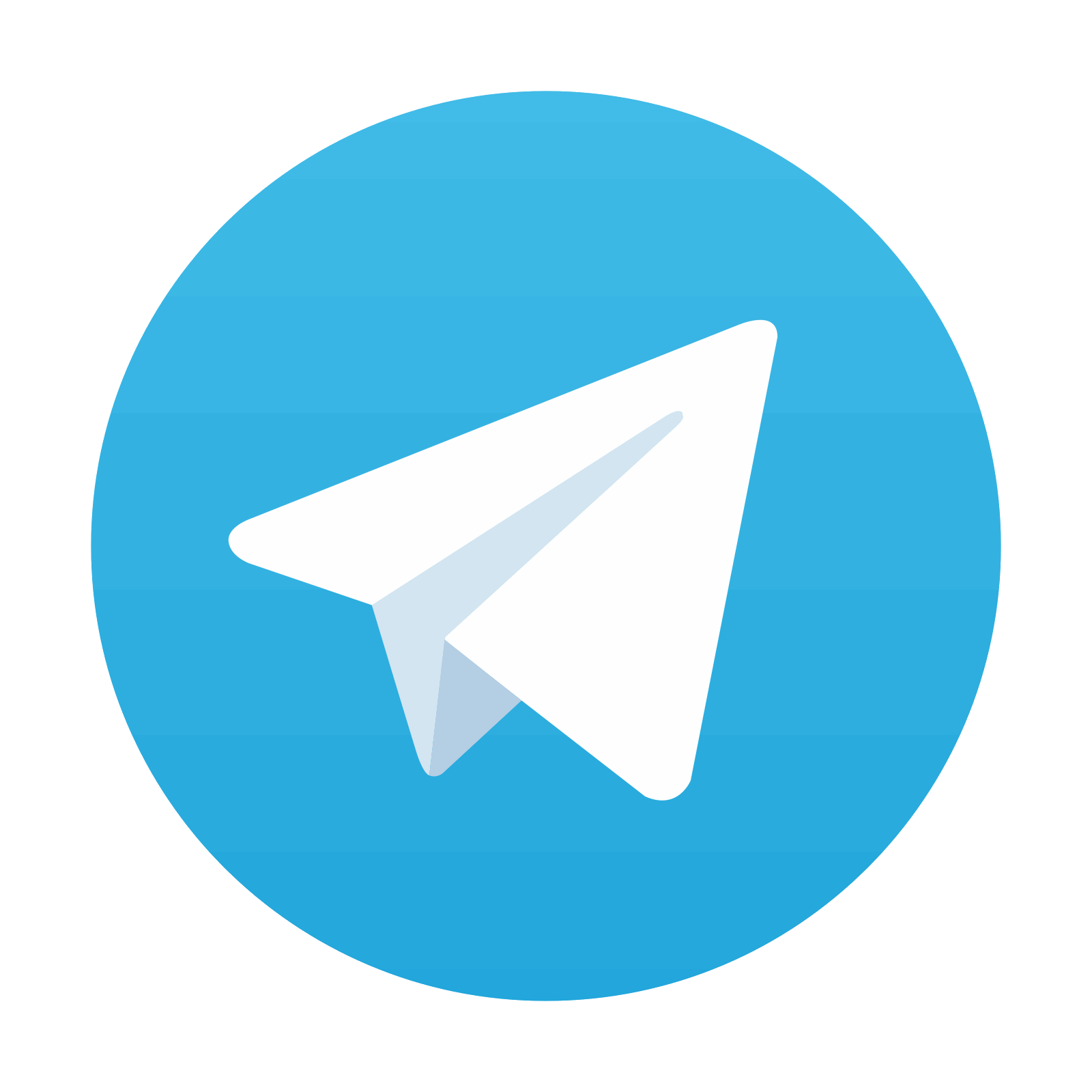
Stay updated, free articles. Join our Telegram channel

Full access? Get Clinical Tree
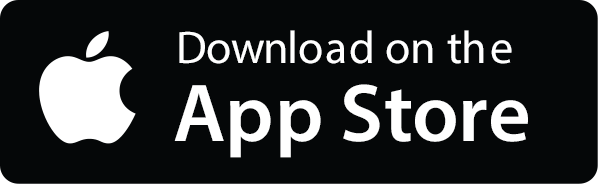
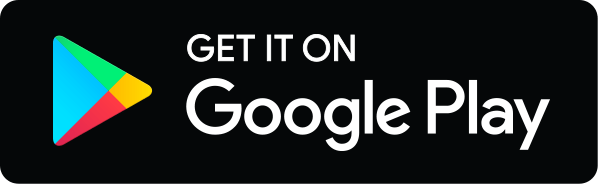