(1)
Department of Dermatology and Allergology Biederstein, Technische Universitaet Muenchen (TUM), Munich, Bavaria, Germany
(2)
Christine Kuehne Center for Allergy Research and Education (CK-CARE), Hochgebirgsklinik (High Altitude Hospital), Davos, Switzerland
3.1 Genetics
3.1.1 Classical Genetics of Atopic Dermatitis
Since the introduction of the term “atopy” by Coca and Cooke in 1923 and the inclusion of the correspondent skin disease atopic dermatitis or atopic eczema by Wise and Sulzberger in 1933, the familiar occurrence of these diseases is common knowledge in textbooks (Schnyder 1960). Classical genetics examine the occurrence of diseases or symptoms of certain phenotypes within family trees. Twin studies allow the clear-cut estimation of genetic factors versus environmental influences when monozygous and dizygous twin populations are compared (Schultz-Larsen 1985) (Fig. 3.1). Since Gregor Mendel, various hereditary models can be determined.
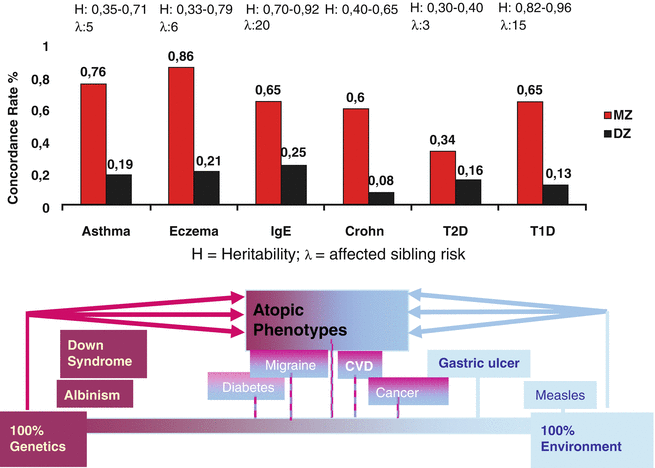
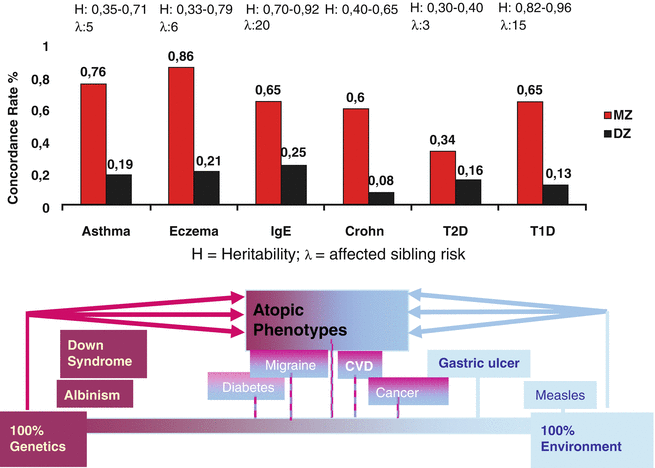
Fig. 3.1
Atopic eczema as genetically determined disease. MZ monozygous, DZ dizygous
When the determination of the phenotype is not exactly precise, difficulties may arise, e.g., history with the definition of family with regard to positive family history or with regard to the degree of relationship, the attribution of paternal versus maternal hereditary factors (“mater semper, pater numquam constat”), the overexaggeration of ascendant (retrospective) versus descendant (looking into the future) family history, as well as unprecise definitions of atopy versus atopic disease (Moffatt and Cookson 1998; Uehara and Kimura 1993).
The first detailed investigations with regard to family history and genetic risks of atopic diseases were done by Urs Schnyder in Zurich, where he found that the risk of a child to develop atopic eczema is around 15 % when both parents are healthy while it is 25–30 % when one parent is atopic and 66 % when both parents are atopic (Schnyder and Klunker 1957; Schnyder 1960). Twin studies showed a concordance rate of 80 % in monozygous twins compared to 25–30 % in heterozygous ones (Blumenthal and Jaffe 1933; Schultz Larsen and Holm 1985; Wüthrich 1975).
Unfortunately there are only few similar studies with regard to the phenotype “atopic dermatitis” alone. Family studies with long-term observation from Linköping (Kjellmann 1977) have shown that the atopy risk for children of atopic parents is then highest when both parents suffer from the same atopic manifestation (e.g., both parents suffer from atopic eczema) (Table 3.1). Apart from the “tendency to IgE formation,” also the organ manifestation is somehow inherited.
Table 3.1
Atopy prevalence in children and parental atopy (Kjellmann 1977) (percent in the 12th year of life)
Parents | Atopyprevalence (%) |
---|---|
No atopy | 10–15 |
One parent is atopic | 20–30 |
Two parents are atopic(different manifestation) | 30–40 |
Two parents are atopic(same manifestation) | 60–80 |
Atopic eczema as a complex disease does not follow the simple Mendel heredity, but shows a polygenic inheritance under multifactorial influence and partly genomic imprinting (maternal influences stronger than paternal ones). This maternal dominance can be explained by epigenetic effects. There are critical opinions with regard to the methods and data analysis of family history. When parents are asked for early childhood diseases (e.g., cradle cap or infantile eczema) in their own personal life, it may be that women have a better memory since they may be more somatically oriented than boys already in youth and spend more time to external appearance while boys and men probably remember sports or traffic accidents better. Therefore studies using descendant family history are interesting as they have been performed by Uehara in Japan who compared parents with atopic eczema and asked them about the diseases of their children (Uehara and Kimura 1993). In 270 parents with a total of 529 children, the rate of atopic eczema was 60 % with absolute equality regarding gender (59 % boys, 60 % girls). In subclassification of adults with regard to the atopic diathesis in the spouse, the highest eczema rates were found in children from parents in whom both father and mother were suffering from atopic eczema (Table 3.2). There was no difference in eczema prevalence between parents in whom one partner was non-atopic or suffered from respiratory atopy. In another study, Uehara compared the paternal and maternal effects and found that for 285 adults with atopic eczema who were married to a non-atopic partner and had at least one child, there was no difference in the prevalence of atopic eczema in their children between families in which the father was suffering from eczema compared to families in which the mother was affected. Totally 57 % of children suffered from atopic eczema (Uehara et al. 1999).
Table 3.2
Incidence of atopic eczema in children from families with atopic eczema in one or both parents (Uehara and Kimura 1993)
Partners of patients with atopic eczema | N (families) | N (children) | Children with atopic eczema |
---|---|---|---|
Parents of partners non-atopic | 164 | 321 | 180 (56 %) |
Atopic eczema | 26 | 59 | 48 (81 %) |
Respiratory atopy | 80 | 149 | 88 (59 %) |
Total | 270 | 529 | 316 (60 %) |
Another problem of classical genetics can be seen in the decreasing number of children in many countries of the world; it is obvious that the frequency of a positive family history is higher in larger families than in one-child families.
In so-called “pure” eczema patients who are not affected by respiratory atopy neither in the personal nor in the family history, lower IgE levels and a higher percentage of “intrinsic” eczema can be found. It might be interesting to study specially this patient group with regard to molecular genetics. Schultz-Larsen found in a Danish twin study similarly high concordance rates for intrinsic atopic dermatitis as for IgE-associated cases (77 % monozygous versus 15 % dizygous) (Schultz Larsen and Holm 1985).
3.1.1.1 Summary
Atopic diseases are strongly genetically influenced. This is evident from twin studies where monozygous twins show a concordance of 80 % of atopic eczema while dizygous twins only show ca. 20 %. The risk to develop atopic eczema is 40 % for children with one atopic parent. When both parents are affected by the same manifestation of atopy (e.g., father and mother with atopic eczema), the risk is between 70 and 80 %. Similar high concordance rates can be found for extrinsic (IgE-associated) and intrinsic (non-IgE-associated) atopic dermatitis.
3.1.2 Molecular Genetics
Since it became clear that atopic dermatitis as a complex disease cannot show monogenous hereditary traits following Mendel’s laws, there was the hope to define an atopy gene (Cookson and Moffatt 2002; Cookson et al. 1992; Marsh et al. 1981). In the meantime we know that a multitude of gene loci on various chromosomes are involved in the manifestation of the many phenotypes of atopic diseases. The modern methods of molecular genetics use naturally occurring DNA sequences which are distributed as polymorphous markers (microsatellites) over the whole genome and allow the determination of gene loci. In various studies, coupling analyses were performed when genetic markers and defined clinical signs and symptoms cosegregate within families (e.g. affected peer), while association studies use larger populations of affected persons and compare them with non-affected individuals with regard to allele distribution patterns of polymorphous gene markers. Principally there are two strategies for investigations:
Candidate gene analyses
Genome-wide association studies (GWAS)
In candidate gene analyses, genes are selected on the basis of their known function in encoding pathophysiologically relevant products for the disease investigated. It is thought that changes in the function of certain substances determine the susceptibility of an organism to the occurrence of a certain disease.
The best suitable model here is the Family Trio study using the transmission disequilibrium test (TDT). Here the transfer of a sign from heterozygous parents to an affected child is investigated; a disease-relevant allele should be more common in the affected child than a non-relevant gene.
3.1.2.1 Genome-Wide Association Studies
Using the microsatellite markers, the whole genome of many affected individuals can be screened and compared to non-affected controls. Associations are determined with certain disease-specific phenotypes. With this approach, totally new until now unknown genes with unknown functions can be detected which then can be further investigated.
Table 3.3 shows a review over the known gene loci with association to markers of atopy or atopic eczema. The progress in this area is so rapid that almost monthly new results are published (Kabesch et al. 2003, 2006; Moffatt et al. 1994, 2007; Vercelli 2008; Bussmann et al. 2011).
Table 3.3
Atopy and atopic eczema: associated Candida genes
Region | Candidate gene | Associated phenotypes |
---|---|---|
1q31-32 | ? | Psoriasis |
1q21 | EDC: filaggrin | Ichthyosis vulgaris, atopic eczema, asthma? |
3p24 | Chemokine RANTES | – |
3q21 | COL 29A1 | Atopic eczema |
4q35.1 | IRF-2 | – |
5q31 | SPINK5 | Netherton syndrome |
Common loci with IBD | IL-4 cluster | Asthma, rhinitis, SPT, total IgE |
Common loci with IBD | IL-13 | Asthma, BHR, SPT, total IgE |
Common loci with IBD | CD14 | Asthma, rhinitis, SPT, total IgE, specific IgE |
11q13 | FCER1B, FCER1A | Atopy, asthma, total and specific IgE, atopic eczema |
13q12-14? | – | – |
14q11.2 | Mast cell chymase | – |
16p11.2-12.1 | IL-4-receptor A | Asthma, BHR, rhinitis, total IgE |
17qcen-q11 | NOD 2a | Asthma, SPT |
17q11-q12 | RANTES | Asthma, eosinophils |
– | MCP1 | Asthma, eosinophils |
17q21 | Eotaxin | Asthma, BHR |
19b13.3 | TX2 | Total IgE |
19q13.1 | TGFB1 | Asthma, SPT, total and specific IgE |
The critical parameter for successful molecular genetic analysis is the exact characterization of the phenotype investigated; here the experienced clinician and exact observation and determination of morphology are crucial.
In the following, some gene loci will be shortly discussed. In the GWAS it became apparent that overlaps of relevant gene loci between atopic eczema and asthma but also between atopic eczema and psoriasis have been observed (Lee et al. 2000) and also to Morbus Crohn. This lets us speculate that there are common risk factors for inflammatory diseases of barrier organs like skin and gut. The most exciting results can be seen in the discovery of polymorphisms and deficiency mutations of the filaggrin gene as causal factor for ichthyosis vulgaris by the group of McLean and Irvine which also showed highly significant associations with atopic eczema (Palmer et al. 2006; Smith et al. 2006; Weidinger et al. 2007, 2008a) (see Sect. 3.2).
Another important factor in the evaluation of genetic effects is interactions between environmental influences and genetic factors: So certain genetic alterations only become clinically relevant after exposure to certain environmental factors such as allergens or pollutants (tobacco smoke) acting together.
3.1.3 Genes of Skin Barrier Function
Among the many genes associated with markers of atopy or atopic dermatitis, specially interesting associations were found with genes on chromosome 1 in the area of the so-called epidermal differentiation complex (EDC) (Fig. 3.2).
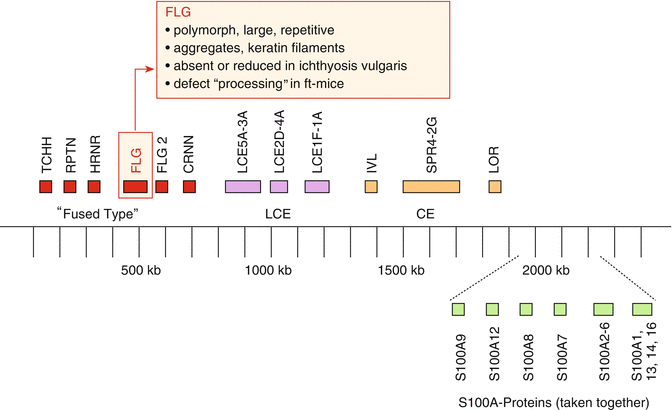
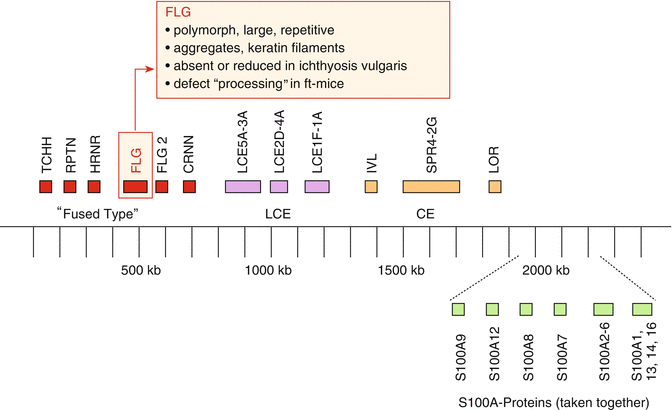
Fig. 3.2
Epidermal differentiation complex (EDC) on chromosome 1, part 1p.21, with various gene loci relevant for epidermal barrier function (Modified after Weidinger (2009))
3.1.4 Filaggrin
Apart from many proteins of the so-called S100 group, filaggrin is crucial in the development of a normal skin barrier. This protein is formed from profilaggrin within the keratohyalin granules by proteolysis and plays a role in the aggregation of keratin filaments in the upper epidermis, keeping lipids and proteins together in between the dead keratinocytes of the stratum corneum (Fig. 3.3).
When a filaggrin loss of function mutation had been shown to be causal for the autosomal dominant variant of ichthyosis vulgaris, the most common genetic keratinization disorder, it was logical to study this mutation also in patients with atopic eczema. Indeed, and numerous studies have shown this, it was found that also over ethnic groups, Caucasians and Asians, highly significant associations have been found between filaggrin loss of function mutation and atopic dermatitis with high significance rates (Baurecht et al. 2007; Rodriguez et al. 2009).
In Caucasians, two most common filaggrin variations (R501X, 2282de14) as well as 18 less common variants have been described in European patients and controls. In addition, there are many mutations most commonly seen in Asian patients, but all these variations lead to loss of function mutations in the filaggrin molecule with consecutive disturbance of skin barrier function.
While filaggrin mutations have been found in almost all ethnic populations studied worldwide, there was a lack of information with regard to Africa (George 1989). Recently a study was performed in Ethiopia in 103 patients with clear-cut AD and seven patients with ichthyosis vulgaris compared to controls. No carriers of filaggrin mutations of the four most common FLG mutations were found; only in one patient with eczema, a new heterozygous mutation was detected. The authors concluded that filaggrin loss of function variants are less common in the Ethiopian population and maybe other factors are more important in this group of patients (Winge et al. 2011).
In a meta-analysis of 24 studies in a total of 6448 patients with atopic eczema and 26,787 controls in 1993 as well as 17 studies with 3138 cases of asthma, there was a high evidence for the role of filaggrin in the development of atopic eczema (Baurecht et al. 2007).
It is of interest that filaggrin mutations also are associated with the marker “increased IgE” as well as with “eczema plus asthma.” There is no association for asthma alone.
The prevalence of filaggrin mutations in Europe in heterozygous carriers is 15 %; homozygous carriers of the filaggrin mutations develop ichthyosis vulgaris; heterozygous carriers have a three- to fourfold increased risk for atopic eczema.
It remains to be stated that there are patients with atopic eczema without filaggrin loss of function mutation; therefore, filaggrin cannot be regarded as a definite and single causal factor. Furthermore, there are many (around 7 %) filaggrin mutation carriers without any skin problems.
While at the beginning of the filaggrin mutation discovery one had hoped to find a positive marker for the diagnosis of intrinsic (non-IgE-associated) atopic eczema, this was not the case; especially the extrinsic variant is associated with filaggrin mutations (Weidinger et al. 2008a). Exposure to cat allergen in early life increases the risk of atopic eczema in children, especially in a subgroup carrying the filaggrin mutation (Bisgaard et al. 2008). Therefore, it has been speculated that the filaggrin mutation leading to a disturbed skin barrier facilitates the penetrance of exogenous noxious substances or allergens, then inducing IgE responses.
Filaggrin concentrations are decreased in patients with atopic eczema. There are clear-cut relations between the severity of eczema and filaggrin mutations (Nemoto-Hasebe et al. 2009) which are controversially discussed (Hubiche et al. 2007).
Filaggrin is further degraded in the epidermis into amino acids and thus represents a large part of so-called natural moisturizing factors contributing to skin barrier and smoothness.
Right next to the filaggrin locus within the EDC, the S100 calcium-binding protein S100A7 (= psoriasin) is encoded which is highly significantly associated with psoriasis (Gläser et al. 2009a).
Filaggrin seems to be expressed particularly in the epidermis. With regard to mucosal surfaces, it can be found in the oral mucosa, but neither in the airway nor in the esophagus (De Benedetto et al. 2008).
Filaggrin mutations not only confer the most significant risk for atopic eczema, but also associations with allergic rhinitis and asthma have been found in several studies. However, in large-scale studies it has been found that asthma per se and allergic rhinitis per se are not connected with FLG mutations, yet such loss of function mutations increase the risk to develop asthma plus eczema (Weidinger et al. 2008a, b).
In animal experiments with knockout mice for filaggrin, these showed very dry and scaly skin, but normal stratum corneum hydration and transepidermal water loss. Antigen penetration was found to be increased, so it might be that the increased TEWL only occurs secondary to skin inflammation (Kabashima et al. 2013). Similarly, in the spontaneous mouse mutant model of the flaky tail mice (ft), it could be shown that topical penetration of allergens is significantly increased, resulting in cutaneous inflammation (Vallon et al. 2009).
The loss of function of filaggrin not only leads to dry skin and increased sensitization, but also seems to be involved in the development of photosensitivity. This was studied in a three-dimensional skin model in vitro by the group of Tschachler using small interfering RNA (siRNA) to specifically suppress filaggrin formation at different levels.
Indeed, there were keratohyalin granules reduced in number and size and disturbed lamellar body formation. However, the absence of filaggrin did not affect the susceptibility to urea extraction; however, a reduced concentration of urocanic acid in the epidermis was observed, rendering the skin more susceptible to UVB-induced apoptosis (Mildner et al. 2010).
In a large epidemiological trial, it was interesting to find significantly elevated vitamin D concentrations in serum to be associated with filaggrin mutation status, possibly due to increased UV effects (Thyssen et al. 2010).
With regard to food allergy, filaggrin loss of function mutation did not predict clinical food allergies; however, it was associated with an increased risk of food sensitization in the first year of life, making it likely that food sensitization may occur through impaired skin barrier function (Tan et al. 2012).
Filaggrin loss of function mutations are associated not only with an increased risk of eczema, but also of fissured skin on the hands, as has been shown in a large cross-sectional population study in Denmark (Thyssen et al. 2012) as well as the severity of occupational irritant contact dermatitis of the hands. These authors found that in the presence of atopy, filaggrin mutations may be a marker for more severe manifestation of the clinical course of irritant dermatitis.
Also allergic contact sensitization to nickel has been found to be associated with filaggrin mutations (Novak 2009; Thyssen et al. 2010). However, compared to other causal factors, the filaggrin mutation was a weaker contributing factor than the well-known effect of early ear piercing. Also chronic hand eczema was studied in a German cohort and found to be significantly associated with heterozygosity for filaggrin mutations (Molin et al. 2009).
The connection between ichthyosis vulgaris, filaggrin and allergic sensitization was studied in a group of 26 patients with ichthyosis vulgaris, where a high presence of CD1a-positive cells in the epidermis was found in a subgroup of IV plus atopic sensitization or atopic eczema and found to be significantly increased compared to ichthyosis vulgaris patients without atopy (Oji et al. 2010).
Filaggrin mutations are the strongest genetic determinants of atopic dermatitis and may help to define risk profiles of children as an early prognostic marker. Unsurprisingly, there was no association with filaggrin mutations when patients with ophthalmic complications such as keratoconus were investigated which was even below the expected frequency (Droitcourt et al. 2011).
Equally, filaggrin loss of function mutations have been found not to be protective against acne vulgaris as might have been speculated with regard to the dichotomy of dry versus greasy skin.
3.1.5 Protease Inhibitors
On the basis of the protease inhibitor defect in Netherton syndrome (SPINK5), which encodes an important serine protease inhibitor LEKTI, corresponding investigations have been performed in atopic dermatitis (Hubiche et al. 2007). There were decreased levels of kallikrein (KLK)-dependent peptidases. In a transgenic mouse model with overexpression of KLK7 with chymotryptase activity, skin changes similar to atopic dermatitis developed (Cork et al. 2009). In a candidate gene analysis, however, no significant association of KLK7 mutations to atopic eczema was found in humans (Weidinger et al. 2008a, b).
On the other hand, associations of polymorphisms of mast cell chymase have been shown with infantile atopic eczema (Mao et al. 1996).
Epidermal serine proteases act via signal transduction of protease-activated receptor (PAR)2 G-protein-coupled receptor which plays a role in innate immunity, but also in mediation of itch sensation (Steinhoff et al. 2003). Activation of PAR2 leads to inhibition of “lamellar” bodies and stratum corneum formation. The change in potassium gradients with concomitant activation of PAR2 receptors leads to a rapid transformation of the external keratinocytes in the stratum granulosum to terminally differentiated corneocytes (Hachem et al. 2003).
The thickness of epidermis, the thickness of stratum corneum, and the size of the corneocytes also influence barrier function. Hölzle and Plewig found smaller corneocytes in atopic dermatitis compared to healthy controls (1977). The smallest corneocytes were found in the retroauricular area and on the forehead, sites commonly affected by atopic eczema.
Other studies found an association of SPINK5 gene mutations to atopic eczema (Cork et al. 2009; Weidinger et al. 2008a, b).
In addition to the SPINK5 locus, a mutation in cysteine protease inhibitor cystatin A (CSTA and chromosome 3q21) was described which shows association to atopic dermatitis (Lee et al. 2000).
3.1.6 Genes of Innate Immunity
The innate immune system is the oldest active defense system in the evolution and protects the organism against a variety of exogenous intruders with a rather rapid reaction which somehow is able to recognize and differentiate pathogenic structures on microbes or other “danger” signals (Matzinger 1994; Janeway and Medzhitov 2002). Apart from the physicochemical barrier at the surface, various cellular and secretory elements are involved which are activated after stimulation of so-called pathogen-related receptors (PRR) (Akira 2006); all of them may be altered in a sense of being “defective” or “less efficient” in atopic dermatitis such as the response of the following PRRs:
Toll-like receptors (TLRs)
Nucleotide-binding oligomerization domain (NOD)
Leucine-rich repeat-containing proteins (NLR) as well the endotoxin receptor CD14
Some soluble PRRs (De Benedetto et al. 2009)
Thus major cells of the innate immune response like polymorphonuclear leukocytes, natural killer cells, and dendritic cells, especially pDC, have been found to show reduced functions (De Benedetto et al. 2009). Also the production of antimicrobial peptides (AMPs) from keratinocytes is reduced as well as the secretion of CCL20/MIP-3-α or CXCL8/interleukin-8.
Functioning innate immune responses are the basis of the establishment of a specific adaptive immune response. When the innate immunity is weaker, dysregulation of T-cell reaction patterns may occur. Toll-like receptor activation after epicutaneous allergen sensitization plays an important protective role in allergy development (Haapakoski et al. 2013). Impaired innate immune reactions can give rise to Th2-dominated reaction patterns (Mrabet-Dahbi and Maurer 2010).
These findings may lead to a new understanding of the mechanisms of the so-called “hygiene” hypothesis with regard to allergy and eczema development (Frei et al. 2012; Kemeny and Szabo 2013).
An association of polymorphisms of TLR2 gene with severe atopic eczema and common bacterial infections has been found (Ahmad-Nejad et al. 2004). There was no association between TLR4 and TLR6 (Weidinger et al. 2008a, b); however, there was significant association to a polymorphism in TLR9 (Novak et al. 2007) similar to the NOD1 gene, a molecule of signal transduction in innate immunity against bacteria (Weidinger et al. 2005).
3.1.6.1 Antimicrobial Peptides
An important part of innate immunity in the skin is antimicrobial peptides in the epidermis, such as defensin or cathelicidin. A study from Korea found significant polymorphisms for Defensin Beta1 (DFB1) in atopic eczema (rs5743399 and C haplotype) which are especially common in the extrinsic variant of atopic eczema (Kim et al. 2009).
3.1.7 Genes of Adaptive Immunity
In the beginning of the era of molecular genetics, associations between atopic disease and genes on chromosome 5 were already discovered, where the so-called cytokine cluster is located between 5q31 and 5q33 and encoding for a variety of candidate genes such as interleukin-4, interleukin-13, interleukin-9, and interleukin-5, but also for CD14 (receptor for endotoxin) (Marsh et al. 1994; Rosenwasser et al. 1995). Many gene associations in this cluster were found for atopy in general, IgE, but also respiratory atopic diseases; for atopic dermatitis, they have to be specially considered and only associations for certain subtypes were found (Novak 2009).
Similar findings have been observed for the STAT6 signal transduction marker (signal transport and activation in T cells) (Weidinger et al. 2004a).
The genes encoding for the chemokine RANTES or the transcription factor GATA equally were found to be associated with atopic eczema (Lee et al. 2000).
Another interesting association between polymorphisms of the high-affinity IgE receptor FcεR1 (Cookson and Moffatt 2002; Weidinger et al. 2008b) has been found. Obviously mutations in the alpha chain of the high-affinity IgE receptor have a special importance with regard to IgE production (Weidinger et al. 2008b, 2010).
3.1.8 Gene Loci with Yet Unknown Function
In one of the first GWAS, Lee et al. observed a highly significant association between atopic eczema and a region on chromosome 3q21 where the proteins CD80 and CD86 as costimulation molecules in T-cell activation are encoded (Lee et al. 2000).
On chromosome 11, recently a gene variant was identified as significantly associated with a risk for atopic eczema. Here a protein named EMSY is encoded which is present in various tissues, however, especially in the skin and in immune cells. There was a highly significant association also to inflammatory bowel disease (Esparza-Gordillo et al. 2009).
A meta-analysis of meanwhile numerous GWAS with a total of approx. 40,000 individuals, among them close to 10,000 patients with atopic eczema and 30,000 controls, first studied the ten most significant associations and then in a second set tried to reproduce these in other cohorts. Apart from filaggrin, which was significant in all studies, three loci were found to be consistently and reproducibly associated with increased risk of eczema, namely, OVOL1, KIF1A, and ACTL9 (Paternoster et al. 2012).
In a genome-wide association study (GWAS) combining Caucasian and Asian patients in a total of over 20,000 patients, altogether 11 susceptibility loci for atopic dermatitis have been described, many of them without known function at present (Ellinghaus et al. 2013 neu). In a GWAS in China, also previously undescribed loci with high association to atopic dermatitis have been identified (Sun et al. 2011 neu).
It has become clear that the future of molecular genetic research will be in close conjunction with precise clinical phenotyping and functional analysis of gene environment interactions. Now we know that in the manifestation of symptoms, a multitude of genes is involved.
Animal models with knockout mice or transgenic mice with overexpressed genes may help to elucidate the pathophysiological processes (e.g., flaky tale mouse with a combined defect in filaggrin and hornerin and eczema-like symptoms).
3.1.9 Summary
With the new methods of molecular genetics, it was possible to identify certain loci on various chromosomes associated with specific phenotypic markers of atopic eczema. Some of these genes already have been positionally cloned. Genes of the epidermal differentiation complex (EDC) on chromosome 1 seem to have special importance (such as filaggrin and possibly hornerin) which are highly significantly associated with increased risk for atopic eczema. Homozygous carriers of filaggrin mutations develop ichthyosis vulgaris; heterozygotes have a three- to fourfold increased risk for atopic eczema. Also genes of epidermal proteases or protease inhibitors seem to play a role, like SPINK5 in the Netherton syndrome, a monogenic disease. Also genes of innate and adaptive immunity are involved in the pathophysiology of atopic dermatitis. Genome-wide association studies (GWAS) help to discover new gene associations whose function is not yet clear, such as on chromosome 11q, but also mutations in the area of the alpha chain of the high-affinity IgE receptor. It is clear by now that there is not only one atopy gene, but that in the development of the phenotype of an atopic disease, a multitude of genes on several chromosomes have to act together with environmental influences.
3.2 Disturbance of Skin Functions: “Sensitive” Skin, “Dry” Skin, and Disturbed Barrier Function
3.2.1 Structure and Function of the Skin
In order to better understand the processes in the pathophysiology of atopic dermatitis, it is important to understand the structure and function of normal skin. The skin with a size of 1.5–2 m2 is the largest organ of the body. Many are astonished that the skin is called an “organ”; they feel it is more of a “cover” for the other “true” organs.
3.2.2 The Skin in Evolution
When the species Homo sapiens is regarded with respect to particularly advantageous development of certain organs over the millions of years of evolution, normally the research focuses on the nervous system and the development of the brain. Few studies take the development of the human skin with regard to evolution into account. Therefore, a short reflection of this aspect will be given. The earliest correlate of a “skin” can be found in the Metazoa (e.g., Porifera [sponges]) which have not yet really separated their internal body from the external aqueous environment densely. A structure called pinacoderm represents a very thin one-cell layer which is often disrupted to allow water flow. The Coelenterata (e.g., hydra) already show real epithelium closing the internal part of the body with a one-layered ectodermis overlaying an endodermis.
Further specialization with the development of a mesoderm or entomesoderm between the two other layers can be found in helminths. Arthropods have an important feature, namely, an exoskeleton consisting of chitin and proteins as cuticula connected to the underlying epidermis via hemidesmosomes. This cuticula becomes even more pronounced in Echinodermata (e.g., sea urchin).
Fish also produce an exoskeleton with a multilayered epithelium and the development of scales in the upper dermis (placoid scales). The major change occurred in Amphibia when they as the first animals conquered the land and had to cope with a dry environment. Here cornification of the most upper layers of the epidermis occurs; the secretory glands migrate down to the dermis. Cornification is more pronounced in Reptilia with a dry epidermis which periodically is shaded off sometimes within minutes as in the gecko species.
In birds, the skin developed feathers important for the temperature equilibrium and for movement in the air. The avian epidermis only consists of two layers: the stratum germinativum and the stratum corneum. There are no sweat or sebaceous glands. Feathers develop from a protrusion of epidermis with a central dermal pulpa. Finally they consist completely of keratin, only showing connective tissue rests in the papilla.
Mammalians finally have a wide variability from reptile-like corneal scales via dense furs until the relatively thin and reduced skin of humans. Homo sapiens, among all members, has the most reduced type of skin with regard to thickness, scales, or adnexal tissues, except for innervation which is rather well represented with many sensory cells and free nerve endings over the whole skin surface.
3.2.3 Functions
However, the skin is much more than a bag which keeps the human being together so that he does not break apart similar to a sausage—as primitively suspected. The skin is a highly complex system with a multitude of functions (Table 3.4).
Table 3.4
Functions of the skin
Frontier |
Barrier (physical, chemical, biological) |
Metabolic organ |
Immune organ |
Organ to express psychologic changes |
Aesthetic function |
The skin protects us from wind, cold and sun, noxious substances, and parasites. It ensures that the temperature in the body is kept in a way that the necessary metabolic processes can take place.
Only via the skin we can get into direct contact with our environment. Sometimes this contact is painful. The skin is a sensory organ and is the organ where psychologic processes are expressed as is seen, e.g., in erythema e pudore (to become red for emotional reasons which can be embarrassing). Poets have named the skin “mirror of the soul.” There are many proverbial sentences of everyday use involving the skin like “to save one’s skin,” “this gets under my skin,” “to jump out of one’s skin,” etc.
The skin is also an immune organ; all cells and cytokines necessary for the establishment of an immune response are contained in the skin. Similarly to the gut (gut-associated lymphoid tissue (GALT)), one can speak of a skin-associated lymphoid tissue (SALT) (Bergstresser and Takashima 2001; Granstein and Askari 1986).
3.2.4 Structure
Anatomically one can classify the skin from outside to inside into epidermis, dermis or corium, and subcutis (Fig. 3.5).
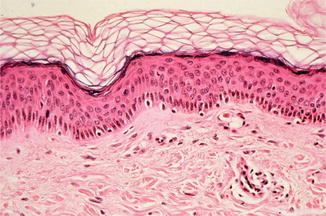
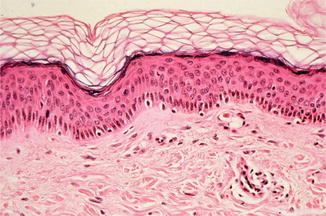
Fig. 3.5
Histological structure of normal skin with dermis and epidermis (hematoxylin-eosin staining) (With friendly permission from Volker Steinkraus)
The epidermis is not of the same thickness on all sites of the body. Dependent upon the localization, the thickness of the epidermis varies between 0.014 and 1.5 mm. The epidermis is formed from inside to outside in four layers:
Stratum basale or stratum germinativum (basal layer)
Stratum spinosum (spinal layer)
Stratum granulosum (granular layer)
Stratum corneum (corneal layer)
Within the epidermis, fine dendritic cells can be found (Langerhans cells) which represent the most external sentinels of the immune system. They act as antigen-presenting cells together with dendritic cells in the dermis which take up foreign substances and present them after migrating to lymphocytes in lymph nodes.
The multitude of cells in the epidermis are formed in the basal layer as keratinocytes producing the most important protein of the epidermis, keratin, but also other proteins (see below). Also keratinocytes are immunologically active and able to produce important cytokines such as interleukin-1 and -6 as well as defensins and other antimicrobial peptides.
The corium, or dermis, consists of connective tissue with collagen and an extracellular matrix produced by fibroblasts. Here also lymphocytes, nerves, and blood vessels are embedded. In the corium reaching into the subcutis are the adnexal structures of sweat glands and sebaceous glands plus hair follicles.
The adipose tissue forms the mass of the subcutis above the fascia of the muscle.
3.2.5 Barrier Function and Stratum Corneum
The barrier function of the skin is important to form a frontier between the organism and the environment. Physiologically it protects the body from pathogenic noxes and is anatomically realized mostly in the stratum corneum. This barrier is not totally impermeable but has permeability to a degree to allow transport between the internal milieu of the organism and the outside. The corneal layer is not “dead skin” but is the end product of a highly differentiated epidermal process where skin cells transform into corneocytes from inside to outside with increasing numbers of keratin filaments which, together with lipids, form the stratum corneum according to the model of “bricks and mortar” (Elias and Menon 1991; Piérard 1989; Seyfarth et al. 2011).
The structural integrity of the stratum corneum is guaranteed by so-called modified desmosomes (corneodesmosomes) which bind corneocytes together and thus build a defense against tangential forces and mechanical trauma. In the model of Peter Elias, corneocytes can be compared to bricks, while the lipid lamellar layers can be compared to mortar. Corneal desmosomes could be regarded as iron rods (Cork 1997; Cork et al. 2005) which supply resistance against mechanical stress (Proksch et al. 2003).
3.2.6 Corneocytes
Corneocytes are flat cells in the terminal differentiation of epidermal keratinocytes which takes place in the stratum granulosum. Here the cells lose their nuclei as well as intracytoplasmic organelles and are connected densely with keratin fibrils. The human stratum corneum consists of approximately 20 layers of corneocytes, each 30 μm in diameter. The keratinocytes of the stratum granulosum release the contents of the keratohyalin granules which then together with keratin filaments form the lipid lamellar matrix of the mortar. The lipid layer consists of cholesterol, ceramides, fatty acids, and cholesterol esters which represent as a coherent lamellar layer in the stratum corneum (Fartasch et al. 1989). In the terminal differentiation of keratinocytes, the cell membrane changes into an insoluble protein matrix, called cornified envelope, to which the lipid layer binds. The cornified envelope consists mainly of structural proteins such as loricrin, involucrin, filaggrin, and smaller proline-rich proteins which are bound together by transglutaminases.
3.2.7 Filaggrin
Filaggrin, which is formed from profillagrin, plays a special role in the aggregation of keratin filaments in the cellular cytoskeleton. Finally filaggrin is further degraded to small peptides and free amino acids which form part of the well-known natural moisturizing factors (NMF) together with lactic acid, sodium pyrrolidone, urocanic acid, urea, and others. The natural moisturizing factors play the decisive role in the binding of moisture in the stratum corneum by binding water to corneocytes. Sodium pyrrolidone and lactic acid are specially hygroscopic and absorb water and thus prevent the formation of fissures between corneocytes (Cork 1997).
Probably the most important causal factor in explaining disturbed skin barrier function in atopic dermatitis may be seen in loss of function mutations in the structural protein filaggrin, which leads to also diminished hydration of the stratum corneum and adhesion of corneocytes. This allows an increased penetration of noxious substances, be it microbial, chemical, or allergenic in nature (Fig. 3.6) (see also Sect. 3.1.4).
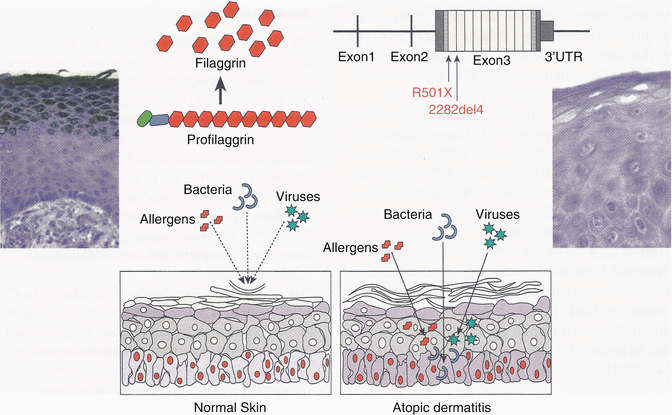
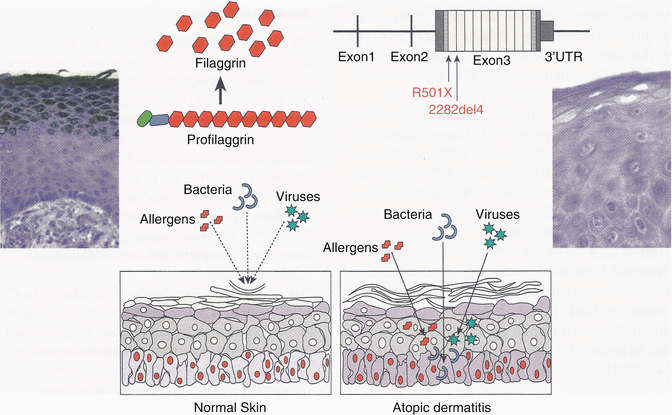
Fig. 3.6
“Loss of function” mutations in the filaggrin gene predispose to atopic dermatitis. With inefficient function of filaggrin, noxious substances make penetration more easily through the disturbed barrier of the epidermis (With friendly permission from S. Weidinger)
Besides filaggrin, other proteins may be involved, such as desmoglein, desmoplakin, but also proteins of the “tight junctions” like claudin-1 and claudin-23 as part of the corneodesmosomes (Fujita et al. 2011).
Furthermore, disturbances of protease and protease inhibitor metabolism have been described in atopic eczema especially based on the gene defect in SPINK5 with diminished LEKTI known from Netherton syndrome.
3.2.8 Corneodesmosomes
The corneodesmosomes are part of corneocyte envelopes and consist mainly of proteins of the cadherin family, a group of extracellular transmembrane glycoproteins. Corneodesmosin is a 52kD protein specially expressed in cornifying epithelia, which, after its secretion in the extracellular space between stratum granulosum and stratum corneum, is integrated into the desmosomes in a way that corneodesmosomes develop.
A very rare disturbance of cornification and skin barrier function has been described as “peeling skin disease” which shares some features with Netherton syndrome, but without “bamboo hair” and SPINK5 mutation. In a family with this rare autosomal recessive ichthyosiform erythroderma, a defect in corneodesmosin has been found to be relevant and another very important factor for epidermal barrier integrity (Oji et al. 2010).
3.2.9 Desquamation
A natural decay of superficial corneocytes leads to a continuous process of desquamation in an equilibrium between keratinocyte proliferation in the stratum basale and desquamation on the surface. The process of desquamation depends upon a net of active proteases and protease inhibitors which degrade extracellular corneodesmosomal structures and thus allow the desquamation of superficial corneocytes. In this process, kallikrein-associated peptidases such as stratum corneum chymotryptic enzyme (SCCE) KLK7 as well as stratum corneum tryptic enzyme (SCTE) KLK5 are involved (Egelrud 1993). Apart from serine proteases, also cysteine proteases like cathepsin L2 (SC thiol protease) and aspartate protease cathepsin D with a pH optimum in the acid range are active. KLK-related serine proteases have their activity optimum in the mild alkaline pH.
All the activities of these proteases are regulated by a mixture of protease inhibitors such as serine leukoprotease inhibitors, but also cystatin protease inhibitors. Cystatin A is formed in sweat and forms a protective film against exogenic proteases from microbes or parasites (S. aureus or house dust mite) on the skin surface. The lymphoepithelial Kazal-type 5 protease inhibitor (LEKTI), which is encoded via the serine protease inhibitor Kazal-type 5 gene (SPINK5), is a special pH-dependent regulator of desquamation. LEKTI is formed in the stratum granulosum and secreted into the intracellular space between stratum granulosum and stratum corneum (lamellar bodies) where it is found colocalized with the above-mentioned kallikrein peptidases in the neutral pH. The more acid the pH to the outside, the weaker the inhibitory potential of LEKTI, thus allowing a normal desquamation in the most superficial layers.
3.2.10 Acid Mantle
Since the germinal work of Marchionini in the 1930s, the concept of the acid mantle of the skin explains a major part of skin barrier function (Marchionini and Hausknecht 1938). The mildly acidic skin surface pH develops together with stratum corneum in the neonatal period with acidification of the stratum corneum that goes along with normal functioning lipid-processing enzymes which are acidic pH-dependent such as the beta-glucocerebrosidase (Behne et al. 2003).
It is a well-known fact that seasonal changes in atmospheric conditions can affect the epidermis and trigger some skin diseases. In hairless mice, various environmental humidity conditions were studied with regard to skin surface hydration and amino acid generation which was drastically decreased in environmental dry air (Katagiri et al. 2008). While the formation of the acid mantle in earlier work was mainly regarded to be due to exogenous substances from colonizing bacteria on the skin surface or free fatty acids from sebaceous glands and eccrine glands, it is known today that also by-products of keratinization together with free fatty acids and phospholipids through phospholipase A2 additionally contribute to the acid mantle.
The acidic pH of the skin surface exerts strong antimicrobial effects (Leyden et al. 1975😉 and improves the adhesion of nonpathogenic bacteria. Furthermore, it plays a role in influencing the above-mentioned proteases and protease inhibitors in the process of desquamation (Schmid-Wendtner and Korting 2007).
3.2.11 Alteration in Epidermal Lipids
Alteration in epidermal lipids has long been regarded as a crucial characteristic of skin barrier dysfunction in dry skin or skin of patients with atopic dermatitis. Apart from lipids from sebaceous glands which have been studied intensively (Gloor et al. 1991) and were also found to be decreased in some patients, it is the intraepidermal ceramides which constitute the majority of epidermal lipids and are important in providing the skin barrier in the stratum corneum (Imokawa 2009). They represent 50 % of stratum corneum lipids. Alterations in ceramide concentrations and patterns have been described (Imokawa et al. 1991).
In this context, possible defects of sphingolipid-metabolizing (SM) enzymes like SM deacylase can contribute to ceramide deficiency in eczema, preferably SM glucosylceramide deacylase, which is an enzyme that cleaves the N-acyl linkage of sphingomyelin and glucosylceramide (Imokawa 2009).
Other lipids include cholesterol; a factor of possible importance for lipid homeostasis could be apolipoprotein C1 (APOC1). In a mouse model transgenic for human APOC1, development of dermatitis, disturbed skin barrier function, and pruritus was observed (Nagelkerken et al. 2007; Proksch et al. 2006).
Long-chain fatty acids contribute to the skin barrier, and fatty acid-binding proteins seem to play a role. In specifically deficient mice, the basal transepidermal water loss was increased with overexpression of fatty acid-binding proteins, and there was a decreased transepidermal water loss and a better repair after acetone treatment (Owada et al. 2002).
3.2.12 Disturbance of Skin Barrier Function in Atopic Eczema
The complexity in the formation of a normal skin barrier function makes it understandable that disturbances at various levels may lead to clinical consequences. Therefore, it is not surprising that many authors studying skin barrier functions observed quite different findings in atopic eczema such as disturbance of the acid mantle with increased pH on the skin surface (Eberlein-König et al. 2000; Seidenari et al. 1996), but also disturbances of lipids and lipid-synthesizing enzymes (Hara et al. 2000; Imokawa et al. 1991; Jungersted et al. 2010; Mustakallio et al. 1967) as well as alterations in protease or protease inhibitors, and structural proteins.
The variations and distribution of several subgroups among ceramides were studied in healthy volunteers and correlated to skin barrier function as measured by transepidermal water loss and a subjective questionnaire. There was no difference between young and old skin with regard to ceramide subgroups or ceramide/cholesterol ratios, although there was a higher ceramide/cholesterol ratio in males compared to females.
3.2.13 How to Measure Skin Function
In studies of skin physiology, a variety of methods are used in order to study the most important functions of the skin. Unfortunately, many of these techniques are only good for scientific investigations under very controlled conditions and not useful for daily clinical practice. Thus the clinically obvious parameter of “sensitive skin” cannot be measured in routine in the same way as bronchial hyperreactivity in respiratory airway disease.
The following methodologies are used to measure skin function:
With the profilometry, the roughness of the skin can be measured. It is increased in atopic dermatitis (Eberlein-König et al. 1996).
The water content of the stratum corneum can be measured with the corneometer and has been shown to only show small or little changes in atopic dermatitis (Eberlein-König et al. 2000)
Secretion of sebaceous glands can be measured with lipid analyses of the skin surface with the sebumeter; some authors found decreased sebaceous secretion (Gloor 1991) which, however, is not consistently measurable.
The best method to measure a functioning skin barrier is the estimation of the transepidermal water loss which determines the liquid water leaving the stratum corneum by passive diffusion as well as gaseous water vapor as a result of sweating. Normally measurements are done below the sweat thresholds so that there should be no eccrine sweat. With disturbed skin barrier, the TEWL increases, which can be measured by different devices, mostly open-chamber systems (e.g., Tewameter, VapoMeter, Germany, VapoMeter, Finland). Recently a new methodology has been described using a condenser chamber where a closed chamber is cooled below the freezing point just as a sink for water vapor; no recovery time is necessary (Farahmand et al. 2009). In patients with atopic dermatitis, also in uninvolved skin, but more marked in eczematous skin lesions, dramatic increases of TEWL can be measured (Gfesser et al. 1997).
Of special interest are studies who measure the repair activity of regenerative capacity of the epidermis after certain stimuli, such as tape stripping of stratum corneum or application of irritants, like sodium lauryl sulfate or dimethyl sulfoxide (DMSO). In many studies, abnormal reaction patterns have been found in patients with atopic dermatitis, sometimes also in patients with respiratory atopy, although the latter is discussed controversially (Cork et al. 2005).
3.2.14 Exposure Studies
In exposure studies measuring effects of indoor air chemicals or pollutants (e.g., formaldehyde or volatile organic compounds (VOCs)), we have found significant impairment of epidermal barrier function in patients with atopic eczema (Eberlein-König et al. 1998; Huss-Marp et al. 2007). Patients were exposed under conditions of normal indoor air with concentrations of the above-mentioned substances below toxic levels in a climate chamber.
Concomitantly epicutaneous application of house dust mite allergen was performed in the atopy patch test (APT): There was a significant increase in intensity of APT reactions after exposure to VOCs, without any clinical dysesthesias from the patients’ side; the patients did not feel any discomfort (Huss-Marp et al. 2007). Tobacco smoke has been identified as the most common indoor air pollutant and can increase the risk of atopic eczema in genetically predisposed individuals, as has been shown in a study in preschool children with actual determination of cotinine in the urine (Krämer et al. 2004).
3.2.15 Clinical Relevance
It can be concluded that the obvious disturbance of skin barrier function already in subclinical levels is of pathophysiological relevance and may give rise to an enhanced penetrance of noxious substances in these patients. Patients with very sensitive skin often in their history tell the following symptoms:
Itch when sweating
Wool incompatibility
Incompatibility with solvents
Incompatibility with UV light
3.2.16 Skin Hygiene and Cleaning Habits
It is an open question whether the increasing hygiene procedures in the Western world have led to an increase in prevalence of atopic dermatitis. Cork et al. have found a significant increase of soap use from 67 million pounds in 1981 to 453 million pounds in 2001 in the UK (Cork et al. 2005). In the same time, also the personal use of water has increased from 11 L per person and day in 1961 to 51 L per person and day in 2001. Furthermore, the degree of hardness of water may influence directly, by irritation and increased soap use, the clinical course of atopic eczema (McNally et al. 1998; Miyake et al. 2004).
3.2.17 Summary
In order to guarantee adequate barrier function of the skin, a physiological exchange between organism and environment has to be possible. This is guaranteed by a complex interplay of proteins and lipids in the stratum corneum. In atopic dermatitis, several abnormalities have been observed; the most important one probably is a loss of function mutation in the structural protein filaggrin, which gives rise to a three- to fourfold increased risk for atopic eczema in affected individuals (see also Sect. 3.1.2). Disturbances in protease and protease inhibitor metabolism also may play a role.
The clinically measurable phenotype of “dry skin” or “sensitive skin” can best be explained as roughness of the skin or as increased transepidermal water loss (TEWL). The increased susceptibility to irritants has practical relevance. Environmental pollutants such as formaldehyde or volatile organic compounds in patients with atopic dermatitis can lead to increased eczematous reactions as has been shown in the atopy patch test with house dust mites. They also can lead to an increased transepidermal water loss.
However, until today there is no simple routinely available test to measure skin barrier function in the daily office such as that for measuring bronchial hyperreactivity in patients with asthma.
3.3 Immunodeviation in the Pathophysiology of Atopic Eczema
In order to better understand the complex mechanisms in the pathophysiology of atopic dermatitis, it makes sense to briefly recall the physiology of normal immune reactivity with the small round lymphocytes in the center; it is only 50 years that their fundamental importance as carrier of specific immunity has been discovered (see Bergmann and Ring 2014).
3.3.1 B and T Cells
In the process of maturation of lymphocytes from stem cells in the bone marrow, the first step of differentiation is the development of the large group of B and T cells. Both arise from undifferentiated stem cells (Fig. 3.7). T cells, which mature within the thymus, are the carriers of cellular immunity (e.g., cytotoxic cells) and immune regulation, while B cells differentiate into antibody-secreting plasma cells.
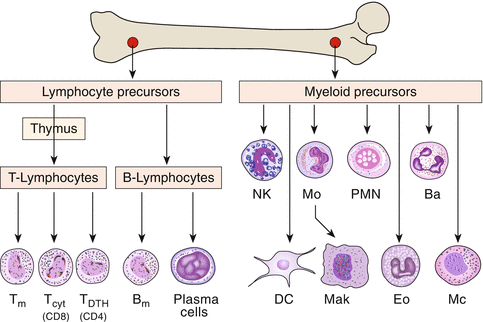
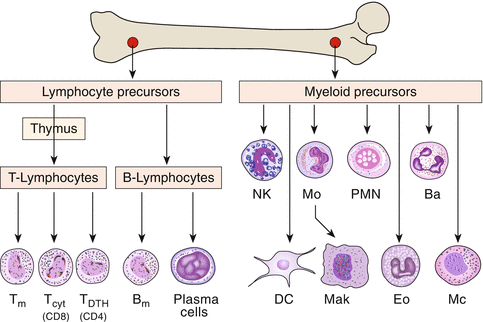
Fig. 3.7
Development of various lines of immune cells from the bone marrow. T m T-memory cells, T cyt cytotoxic T cells, T DTH T cells involved in delayed-type hypersensitivity reactions, B m B-memory cells, NK natural killer cells, Mo monocytes, PMN polymorphonuclear neutrophils, Ba basophils, DC dendritic cells, Mak macrophages, Eo eosinophils, Mc mast cells
T cells mature in the thymus, hence the name. The thymic epithelium provides the immunological identity of cells which helps to establish tolerance against autologous antigens. The precursors of B cells mature in the bone marrow, in birds in a special organ, Bursa fabricii, hence the name.
Mature T and B cells come into the blood and reach so-called secondary lymphatic organs (e.g., lymph nodes) where they come into contact with antigens via antigen-presenting cells, thus starting further steps of differentiation.
Both T and B cells, when specifically simulated, can finally transform into long-living memory cells which only become reactivated after renewed antigen simulation.
3.3.2 Antibodies
Antibodies are proteins—also called immunoglobulins—which are secreted by specially differentiated plasma cells from B-cell precursors. According to their primary structure, five different immunoglobulin classes (isotypes) are distinguished which can be defined according to the characteristics of their heavy chains as G, M, A, D, and E (Fig. 3.8).
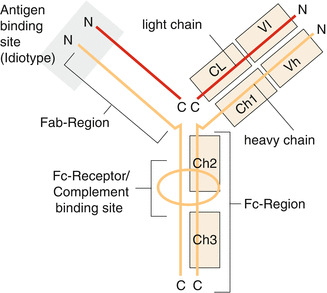
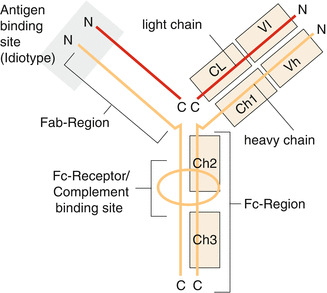
Fig. 3.8
Schematic structure of an immunoglobulin molecule with constant (Fc) and antigen-binding regions (Fab)
IgE antibodies differ from IgG by a fifth domain; they are larger (190,000 kD compared to 150,000 for IgG) and can bind to specific receptors on the surface of mast cells and basophil leukocytes via high-affinity specific receptors (FcεR1) (Fig. 3.9).
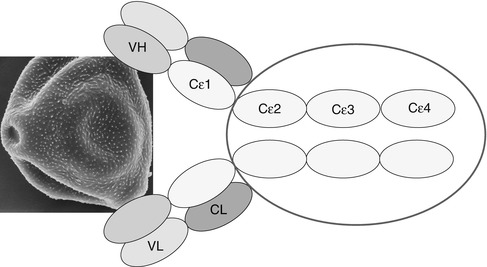
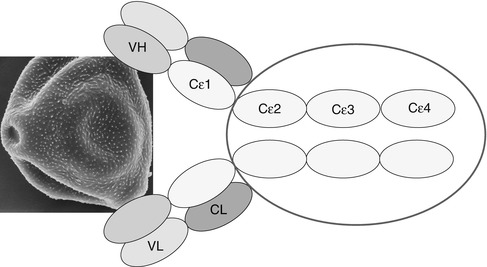
Fig. 3.9
Schematic structure of immunoglobulin E. VH variable heavy chain, VL variable light chain, CL constant light chain
3.3.3 IgE and Atopic Dermatitis
While the pathophysiological processes in other allergic diseases like hay fever or bronchial asthma are quite well understood, the etiopathophysiological concepts in atopic dermatitis still differ with regard to various dysregulations at several levels leading to a complex interplay between genetic predisposition and environmental influences in the development of the eczematous skin lesions.
As illustrated by the multitude of names for this disease (see Sect. 1.2.2), different hypothetical concepts are supported by different research groups—probably also by their subjective special focus (Table 3.5).
Table 3.5
Atopic dermatitis: etiopathophysiological concepts
Genetic predisposition |
Dry skin—disturbed skin barrier function |
Microbial colonization |
Vitamin D deficiency? |
Dysregulation in autonomic nervous system |
Psychosocial factors (stress) |
Inflammation: |
Non-immune (irritative-toxic) |
Allergic DTHa (Th1) |
Allergic atopic (Th2, IgE) |
Autoimmune |
One of the most characteristic features of atopic diseases and especially of atopic dermatitis is the increased tendency towards IgE production. While in the normal organism only little amounts of IgE antibodies are formed (in the range of few ng/mL serum), atopic diseases are characterized by much higher serum concentrations of this immunoglobulin.
In the development of this “immune deviation,” a complex interplay of several cells and cytokines is involved; the major players in this concert will briefly be introduced. It should be stressed that never one cell or subpopulation or mediator alone is sufficient, but it is the total interaction with target and receptors (Metzger et al. 1984) which practically determines the outcome of the immune response in the organism.
3.3.4 Dendritic Cells
Dendritic cells (DC) in the tissue are, next to monocytes in the blood, the major antigen-presenting cells in lymphoid and peripheral tissue; these are the cells which allow the first contact with a foreign substance (antigen) and, by processing, uptake, and presentation of specific structures to lymphocytes, initiate a specific immune response (Lanzavecchia and Sallusto 2001). Without adequate stimulation by dendritic cells, no specific effector T cells can develop.
There are various dendritic cells (Schaekel et al. 2002) which can be classified according to the topography in the skin where we can distinguish between epidermal and dermal DCs.
According to their function, dendritic cells can be classified into the following:
Plasmacytoid dendritic cells (pDC)
Myeloid dendritic cells (mDC)
which in the epidermis can be further classified into the following:
Langerhans cells
Inflammatory dendritic epidermal cells (IDEC)
Dendritic cells can be regarded as sentinels of the immune system and form part of the so-called innate immune response; they express specific receptors for danger-associated molecular patterns (pathogen-associated molecular patterns (PAMP)) as, e.g., the strongly conserved Toll-like receptors (TLR) in the evolution (Terhoorst et al. 2010). Stimulated by danger signals—as mechanic trauma, scratching, heat, etc.—dendritic cells may be activated (Matzinger 1994). Via the production of various cytokines, they influence the differentiation and polarization of T cells in the direction of specifically functional subpopulations.
3.3.5 Langerhans Cells
Langerhans cells have been described as the first group of dendritic cells in the normal human epidermis, originally by the medical student Paul Langerhans (1847–1888) in his doctoral thesis with Rudolf Virchow; because of their dendritic appearance, he believed them to be nerve cells (Langerhans 1868). Only in 1961 was the specific characteristic of Langerhans cells discovered in electron microscopy, the so-called Birbeck granule, which allows the morphological identification of Langerhans cells and can be immunologically detected by the expression of langerin (Silberberg et al. 1974).
Langerhans cells express on their surface markers of major histocompatibility complex (MHC) class II as well as CD1a. They originate from stem cells in the bone marrow via precursors (CD34-positive cells) and differentiate under the influence of certain growth factors such as granulocyte-monocyte colony-stimulating factor (GM-CSF) or TGF-beta (transforming growth factor beta). Langerhans cells can stay over longer time periods (weeks to months) in the epidermis before they migrate to lymph nodes where they present antigens to lymphocytes.
Langerhans cells often are regarded as the outermost sentinels of the immune system in the organism with the function of antigen recognition, uptake, and presentation (Enk and Katz 1992; Jakob et al. 2001; Stingl et al. 1977, 1978). Recently through modern techniques, it has become clear that for the initiation of an immune response, dermal dendritic cells play the crucial role while epidermal Langerhans cells have a more important role in regulatory aspects and tolerance induction (Von Buboff et al. 2004).
3.3.6 Inflammatory Dendritic Epidermal Cells (IDEC)
The second population of dendritic cells in the epidermis is the so-called inflammatory dendritic epidermal cells (IDEC) which do not contain Birbeck granules, but express CD1a and MHCII molecules on the surface and can be detected especially in inflamed skin as, e.g., in atopic dermatitis, psoriasis, and allergic contact dermatitis, but also in mycosis fungoides and other inflammatory skin diseases (Wollenberg et al. 1996).
In the kinetics of the development of an atopic inflammation—using the model of the atopy patch test (see below)—we could show that IDEC can be detected very early in the development of an eczematous skin lesion in the epidermis, both in extrinsic (IgE-associated) and intrinsic (non-IgE-associated) atopic dermatitis (Kerschenlohr et al. 2003a).
3.3.7 Role of Epidermal Dendritic Cells for Atopic Dermatitis
The first note that Langerhans cells may play a role in the pathophysiology of atopic dermatitis came through the detection of IgE molecules on the surface of LC by Bruijnzeel-Koomen (1989) and the detection of the high-affinity IgE receptor (FcεR1) on the surface by the groups of T. Bieber and G. Stingl (Bieber et al. 1992, 1995; Maurer et al. 1994; Wang et al. 1992) (Fig. 3.10). At this time the high-affinity IgE receptor was only recognized to be present on the surface of mast cells and basophil granulocytes, while lymphoid or dendritic cells only could express the low-affinity IgE receptor CD23.
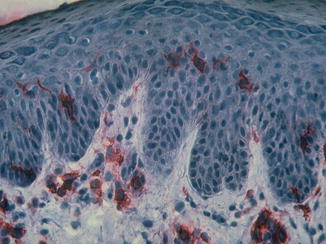
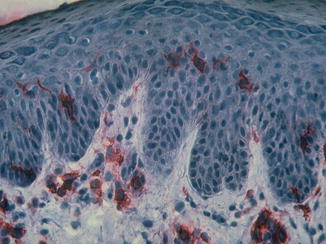
Fig. 3.10
Immunohistochemistry of immunoglobulin on epidermal Langerhans cells (With friendly permission from Thomas Bieber)
Further investigations showed that there are differences in the expression of this receptor: Langerhans cells express the FcεR1 mostly intracellularly, while IDEC show the complete membrane expression of the high-affinity receptor. It is speculated that the membrane-bound IgE also plays a role in the antigen recognition.
A Japanese group showed in immunohistochemical analysis the concomitant presence of IgE antibodies and house dust mite allergen on the surface of epidermal Langerhans cells (Tanaka et al. 1990).
3.3.8 Plasmacytoid Dendritic Cells
The role of plasmacytoid dendritic cells is less well established; after activation, they mediate antiviral responses via production of pDC interferon and are present in peripheral blood of patients with atopic dermatitis. They also carry the three chains of the Fcγ receptor on their surface. They usually are not in the epidermis and seem to be rarer in the dermis of patients with atopic dermatitis, which might play a role in the decreased immune reactivity against viral infections such as eczema herpeticum (Wollenberg et al. 2003a, b).
3.3.9 T Cells and T-Cell Subpopulations
In the immunohistochemical analysis of eczematoid skin lesions, an inflammatory infiltrate can be found, consisting predominantly of T lymphocytes (Werfel 2000) and especially T helper cells (CD4 positive). The role of CD8-positive cells in atopic dermatitis is controversial; however, they can be detected and may play a role in the development of spongiosis (Trautmann et al. 2000). A delayed maturation of T cells in atopy has been discussed as a possible feature (Holt et al. 1992; Prescott et al. 1999, 2003).
The thymic function was studied in atopic dermatitis and psoriasis, using the detection of T-cell receptor excision circles (TREC) which are produced during the rearrangement of the T-cell receptor and represent a possible marker for recent thymic immigrants (RTI), cells freshly migrating from the thymus. In psoriasis, TREC levels were significantly reduced in atopic dermatitis and showed significantly greater variation compared to healthy controls, especially within the CD8 T subpopulation. This suggests that eczema patients have a higher capacity to develop compensatory mechanisms, especially involving CD8 cells, compared to psoriasis patients (Just et al. 2008).
3.3.10 CD4-Positive Cells
Major progress in the understanding of the atopic immune response came through the recognition of highly differentiated subpopulations of T cells, especially T helper cells, starting with the concept of Th1 and Th2 cells (Mossmann and Saat 1996; Coffmann and von der Weid 1997). Table 3.6 lists some of the most important T helper cell populations.
Table 3.6
T helper cell subpopulations
Type | Cytokines secreted | Function |
---|---|---|
Th1 | Interferon-y Interleukin-2 TNF-α | Delayed-type hypersensitivity (DTH) Allergic contact dermatitis Tuberculin reaction Chronic atopic eczema |
Th2 | Interleukin-4 Interleukin-13 Interleukin-5 | IgE formation Parasite defense Atopic eczema initiation |
Treg | Interleukin-10 TGF-β | Tolerance, inhibition of Th1 and Th2 responses |
Th17 | Interleukin-17 |