Failure of Anterior Cruciate Ligament Reconstruction
Keywords
• Anterior cruciate ligament • Graft failure • Loss of motion • Extensor mechanism dysfunction • Osteoarthritis • Infection • Anatomic ACL reconstruction • Extensor mechanism dysfunction
Key Points
Injuries to the Anterior Cruciate Ligament (ACL) are becoming more frequent. Correspondingly there has been an increase in the number of ACL reconstructions performed, with more than 150,000 procedures documented annually in the United States.1 This increase in primary ACL reconstructions almost certainly will lead to an increase in the number of revision procedures. A successful outcome after revision ACL reconstruction depends on many factors, including a comprehensive knowledge of the etiology of failure.
The University of Pittsburgh has created a classification system that combines objective and subjective factors to further elucidate the reason for failure.2 Although multifactorial and subject to significant overlap, recurrent instability can further be categorized into early (<6 months from surgery) or late (>6 months from surgery) failure. Early failures are usually secondary to poor operative technique, failure of graft incorporation, premature return to high-demand activities, or aggressive rehabilitation. Late failures may also be due to poor operative technique or graft malposition as well as repeat trauma to the graft; associated pathology, such as malalignment or collateral ligament injury; and generalized factors, such as ligamentous laxity. Incomplete biologic incorporation may also contribute to late failures.
Graft failure
The true incidence of graft failure is unknown at present. Although graft failure is often defined as a combination of pathologic laxity and clinical instability due to graft rupture or elongation, it is well documented that objective laxity or graft rupture on MRI scan does not always correlate to subjective symptoms of instability.3 Also the degree of laxity that defines graft failure is not universally accepted, with some studies allowing 3 mm of anterior translation (side-to-side difference using an instrumented laxity device)4–7 whereas others accept 5 mm.8–11 In addition, many studies only record failures as those requiring a revision procedure, not including those patients who decline further surgery or have no instability symptoms. Failure rate analysis should include all graft failures from the study institutions that occur during the defined study period, including those reviewed before subsequent graft failure; that were not reviewed because of graft failure; or that were not included because of a failure to satisfy study inclusion criteria.
Although failure rates as high as 24% have been reported,12 most studies have reported graft failure rates in the range of 0.7% to 14%.5,13–22 Several recent systematic reviews by Spindler and colleagues,23 Lewis and colleagues,24 and Wright and colleagues,25 have reported failure rates of 3.6%, 4%, and 5.8%, respectively.
There has been a recent focus on anatomic ACL reconstruction, which has been defined as the “functional restoration of the ACL to its native dimensions, collagen orientation and insertion sites,” independent of whether a single-bundle or double-bundle technique is used.26 Several studies have demonstrated that anatomic procedures better control laxity and knee kinematics.27–30 Most studies, however, comparing single-bundle and double-bundle techniques have not demonstrated a difference in failure rates.31–37 Perhaps surprisingly, the failure rate after anatomic ACL reconstruction has not been shown significantly lower than the failure rate of nonanatomic procedures. A recent study by van Eck and colleagues38 reported a failure rate for the double-bundle technique of 13%, which is at the upper border of failure rates published in the literature. The same study reported an anatomic single-bundle failure rate of 11%, although a possible confounding variable was the use of allograft material. A similar failure rate (12.3%) after double-bundle reconstructions using autograft hamstrings has been reported in a separate study.39 Potential reasons for these findings are discussed later; however, this area requires additional research.
The pattern of ACL graft rupture is different for double-bundle and single-bundle techniques. In a recent study by van Eck and colleagues40 that examined the pattern of rupture for double-bundle grafts, the anteromedial (AM) bundle was ruptured in all cases. The most common patterns were either midsubstance rupture of both AM and posterolateral (PL) bundles or midsubstance AM bundle rupture with elongation of the PL bundle. The same investigators in a separate study examined the pattern of rupture after single-bundle reconstruction and demonstrated the most common pattern in 58% of cases was graft elongation.41 Both differ from the rupture pattern of the native ACL, which tends to demonstrate a proximal AM bundle rupture in combination with a proximal or midsubstance PL bundle injury.42 These studies also showed, however, that with increasing time from surgery to reinjury, the pattern of graft rupture became more proximal or similar to the native ACL rupture pattern.40,41 This is suggestive that the observed injury pattern may closely correlate with the degree of graft healing and ligamentization, which may be enhanced with time.
It is also possible for a graft to be intact but nonfunctional or detrimental to the function of the knee. Nonfunctional intact grafts may allow excessive laxity, particularly in the case of a poorly positioned vertical graft that is able to control anterior translation but not tibiofemoral rotation. Graft malposition may also lead to restriction of knee motion, especially terminal extension, which has been associated with increased rates of long-term osteoarthritis.43
Although multifactorial, the following factors are important to consider when investigating the cause of graft failure (Fig. 1):
Patient Factors
Certain factors inherent to patients, such as age, gender, activity profile, and generalized ligamentous laxity, have been associated with increased failure rates after ACL reconstruction.12,44–51 Other factors involving the initial mechanism of injury47 and preoperative condition of the knee have also been investigated.52,53
Age at the time of failure
Several investigators have observed higher graft failure rates in younger patients. Barrett and colleagues,45 in a study that evaluated both autograft and allograft reconstructions, reported a failure rate of 16.5% in patients under 25 years of age compared with 8.3% in those over 25. Shelbourne and colleagues54 reported similar results after BTB autograft reconstructions. Although the reasons are unclear, it is possible that return to a higher level of activity in the younger age group may be partly responsible. Hewett and Myer55 have postulated a theory proposing a mismatch between neuromuscular adaptation and skeletal growth, which may contribute to ACL injury. These factors when present may persist post–ACL reconstruction in this younger population.
Gender
Although it has been well established that women are 2 to 8 times more likely to suffer an ACL injury than men,56–58 a similar gender difference has not been clearly demonstrated for ACL graft ruptures. In a study performed by Noojin and colleagues,46 women had a clinical failure rate of 23% compared with 4% in men after ACL reconstruction using hamstring tendons. The investigators did not control, however, for confounding variables, such as meniscal or chondral pathology, and included as failures those with a grade 1+ pivot shift (pivot glide). When only patients with at least a grade 2 pivot shift were analyzed, the failure rate in women was reduced to 10%. This compared favorably with the results reported by Salmon and colleagues,48 which excluded those with significant meniscal and chondral pathology and demonstrated no gender difference in graft failure rates (10% in women and 11% in men), a finding supported by several other published studies.54,59,60
Activity profile
Return to a high activity level after ACL reconstruction has been established as an independent risk factor for ACL graft failure. Borchers and colleagues44 demonstrated a higher failure rate with an odds ratio of 5.53 for those patients who returned to a high activity level. In a study by Salmon and colleagues,47 return to competitive sidestepping, pivoting, or jumping sports was associated with an increased risk of ACL injury.
Generalized ligamentous laxity
Generalized joint laxity and specific knee hyperlaxity have been positively correlated with noncontact ACL injuries.50,61–63 It has been hypothesized that excessive knee joint laxity after ACL reconstruction may lead to an increased risk of ACL failure in these patients. Although the exact mechanism is unknown, it has been postulated that hyperlax connective tissues (secondary restraints) and excessive knee hyperextension with secondary graft impingement may contribute to graft failure.
Other factors
Meniscal injury and subsequent meniscectomy at the time of initial ACL surgery have been associated with increased postoperative laxity and, in a cadaveric study, increased in situ graft forces.53,64 Theoretically, this should lead to increased graft failure rates, although this has not been documented in the literature. A contact mechanism of injury at the time of the original ACL disruption was found a risk factor for graft failure in a study of BTB and hamstring autograft ACL reconstructions.47 Finally, higher body weight was associated with graft failure in a prospective analysis of failure rate after anatomic ACL reconstruction using allograft.38
Surgical Technique
Several studies have identified errors in surgical technique as the most common cause of graft failure, with between 50% and 88% of failures believed secondary to technical issues.9,65–68 A recent study, however, from the Multicenter ACL Revision Study (MARS) Group attributed only 24% cases of graft failure requiring revision to technical error alone69 (see Fig. 1).This difference may be attributed to a recently acquired increase in awareness of ACL anatomy and tunnel position, because all patients were enrolled in the MARS study after 2006. This may have reduced the impact of tunnel malposition as a cause of graft failure (see Fig. 1).
Tunnel malposition
Nonanatomic graft placement may lead to excessive length changes of the graft as the knee moves throughout a range of motion.68,70 This in turn may produce secondary graft elongation or rupture, with likely resultant pathologic laxity and instability. If plastic deformation, however, of the graft does not occur, a nonanatomic graft may result in loss of knee motion or excessive compressive forces through articular cartilage and menisci.43,71
Most errors in tunnel position occur on the femoral side. A recent study reported femoral tunnel malposition in 80% of cases where surgical error was believed the primary cause of failure.69 Because the femoral insertion of the ACL is closer to the axis of rotation of the knee, small changes in the femoral ACL attachment may have a significant effect on knee biomechanics. The most common surgical error is an excessively anterior or central tunnel position. An anterior femoral tunnel causes excessive graft tension with increasing knee flexion resulting in loss of flexion, stress at the graft fixation site or eventual plastic deformation of the graft, and pathologic laxity. A central femoral tunnel positioned along the intercondylar roof produces a vertical graft that may be able to control anterior tibial translation but not tibiofemoral rotation. This vertical graft position is also prone to graft impingement against the anterior aspect of the intercondylar notch, which may lead to loss of terminal extension and eventual attritional graft failure (Fig. 2).72,73
Despite less commonly recognized as a cause of failure, accurate placement of the tibial tunnel is critical. A recent study from the MARS Group reported tibial tunnel malposition in 37% of cases where surgical error was believed the primary cause of failure leading to a revision procedure.69 An excessively anterior or lateral placement may lead to notch or lateral condyle impingement as the knee approaches terminal extension.74 To avoid impingement, many investigators previously advocated a posterior tibial tunnel position.72,74,75 This has been shown to cause excessive graft strain in extension as well as posterior cruciate ligament impingement with increasing degrees of flexion, particularly when coupled with a central femoral tunnel position.71 A loss of flexion or progressive graft elongation and failure may result. Although graft obliquity in the sagittal plane largely depends largely on femoral tunnel position, it also depends on the position of the tibial tunnel. A recent study by Bedi and colleagues76 demonstrated that a posterior tibial tunnel produced a vertical graft angle despite being coupled with an anatomic femoral tunnel, which led to an inability to adequately control anterior tibial translation and knee rotation.
Double-bundle procedures are technically demanding and subject to an increased risk of tunnel malposition, because 3 or 4 tunnels are created instead of 2. Although the AM and PL bundles of the ACL function synergistically throughout a range of motion to control anterior tibial translation and tibiofemoral rotation, under loaded conditions both bundles are stretched at low flexion angles.77–79 A recent study by Zantop and colleagues70 demonstrated that a nonanatomic femoral PL graft position resulted in rotatory instability. Several studies have reported increasing in situ forces in the PL bundle or graft as the knee moves from 30° to full extension (Fig. 3A).80–82 Therefore, even small errors of femoral or tibial graft placement may lead to PL bundle overload and failure. Commonly the tibial AM bundle is positioned to avoid anterior impingement, which may influence the creation of a malpositioned posterior tibial PL bundle, which reflects the need to allow an adequate bone bridge. This posterior tibial PL tunnel may produce increased forces within the PL bundle as the knee moves into extension but also hyperflexion, as the length of the PL bundle increases with high degrees of flexion.83 Zantop and colleagues79 demonstrated that PL bundle failure may lead to excessive anterior tibial translation and increased strain on the remaining AM bundle, with overall graft failure a possible outcome (see Fig. 3B).
Graft impingement
ACL grafts may impinge against either soft tissue or bone. The most common soft tissue impingement occurs when a vertically orientated graft with a posteriorly positioned tibial tunnel impinges against the posterior cruciate ligament (described previously). An ACL graft may also impinge against the anterior aspect of the intercondylar notch or the medial aspect of the lateral femoral condyle, particularly as the knee approaches terminal extension. This impingement may lead to focal areas of graft necrosis, inadequate biologic incorporation, and subsequent attritional graft failure.74,84
Most current graft materials have a greater cross-sectional area in their midsection than the original ACL. The native ACL is narrowest in this region, with insertion site areas more than 3.5 times greater than the middle section of the ligament.85–87 This confers a long hourglass configuration to the native ACL, which allows physiologic impingement to occur where the ACL fibers course distally around the anterior aspect of the intercondylar notch. Current graft materials, however, are uniform in shape and often cylindrical. Therefore, impingement may occur when these grafts are positioned too close to the perimeter of the anatomic insertion sites.88 An excessively large tibial tunnel positioned within the anterior aspect of the AM insertion site is the most commonly reported error, which may lead to pathologic graft impingement against the anterior aspect of the intercondylar notch.76 Recent studies have also highlighted the important role of an anatomic femoral tunnel position in preventing graft impingement. This anatomic position produces a more oblique graft orientation in both coronal and sagittal planes, which in turn may reduce the risk and magnitude of graft impingement.89
Improper graft tensioning
The optimal amount of tension that should be applied to an ACL graft has not been identified. It depends on several factors, including the type and size of graft material used, graft placement, type of graft fixation, and the degree of knee flexion at the time of graft tensioning.90–92 The aim of graft tensioning should be to eliminate pathologic laxity without increasing joint contact pressures or producing excessive in situ graft forces, while allowing adequate biologic incorporation of the graft. Yoshiya and colleagues93 in a canine model, reported that highly tensioned patellar tendon grafts demonstrated areas of focal myxoid degeneration with loss of the normal parallel collagen fiber architecture. These grafts also showed areas of poor vascularity.
Other investigators have demonstrated that overtensioning may be associated with overconstraint of the joint, subsequent loss of joint motion, and increased joint contact pressures.94,95 The ideal amount of tension required for a single-bundle graft has not been established, although the degree of tension may be graft type and size dependent.96 Values ranging from 20 N to 80 N have been recommended in published literature.91,97 The most appropriate angle of knee flexion for graft tensioning has also not been established; however, most studies recommend somewhere between 0° and 30°.98 In situ ACL and graft forces, however, particularly within the PL fibers, increase significantly as the knee moves from 30° to terminal extension. More anatomically positioned single-bundle grafts may, therefore, be subjected to excessive graft force if tensioned at 30°, which has led several investigators to recommend tensioning in extension.26,98
The most appropriate graft tensioning technique for double-bundle procedures is controversial. Each technique should recognize and respect the individual tension pattern of the AM and PL bundle as the knee moves through a range of motion. In a recent literature review, van Eck and colleagues99 reported that most investigators (57%) implemented a different tension pattern for the AM and PL graft with the AM bundle tensioned between 30° and 90° and the PL bundle between 0° and 30° flexion. When both bundles were tensioned at the same angle of flexion (27%), the angle ranged from 15° to 60°. A recent cadaveric study by Vercillo and colleagues100 examined various outcomes after the tensioning of the AM and PL bundles at different knee flexion angles. The safest combination was a fixation protocol with the AM bundle tensioned at 45° and the PL bundle at 15°. This combination provided excellent restoration of knee kinematics with graft in situ forces approximating the intact knee. A comprehensive surgical technique article by Karlsson and colleagues98 recommended a similar tensioning technique with the AM bundle tensioned at 45° and the PL bundle in full extension.
Inadequate graft fixation
The fixation should be strong enough to allow immediate weight bearing and range of motion as well as participation in an appropriate rehabilitation program. Bone-to-bone healing is usually complete by 8 weeks and tendon-to-bone healing should be completed by 8 to 12 weeks.101 Failure to achieve this may lead to pathologic laxity and graft failure. Available fixation devices can be divided into extracortical, crosspin, or intratunnel (interference screw) fixation.102,103
Tibial fixation has long been considered more problematic than femoral fixation partly due to the bone quality of the tibial metaphysis but also the effect of parallel forces acting along the ACL graft and tibial tunnel.104,105 The aim of tibial fixation should be to avoid early graft creep and slippage, and several factors, including length of screw, type of screw composition and design, concentric or eccentric screw placement, tunnel dilation, additional extracortical fixation, and graft preparation (whip stitch) have been investigated.103,106,107 Pinczewski and colleagues108 reported that female patients after hamstring reconstruction secured on the tibial side with an interference screw demonstrated greater anterior tibial translation than male subjects. This difference was eliminated with the addition of an extracortical staple, suggesting perhaps a gender differences in bone density and, therefore, fixation strength.109
Lehmann and colleagues110 investigated the impact of the width of the femoral bridge between tunnels had on the structural properties of the graft-tunnel complex after double-bundle reconstruction in cadaveric knees. A cortical bridge of 1 mm between the tunnels produced inferior structural properties compared with a 2-mm or 3-mm bridge. When performing a double-bundle reconstruction, consideration needs to be given to the size of the femoral insertion area, which must be large enough to accommodate both tunnels with at least a 2-mm cortical bridge to reduce the risk of early graft failure.
Graft material/quality
The use of allograft tissue to perform an ACL reconstruction has increased over the past decade. Although satisfactory outcomes have been reported, concerns exist regarding disease transmission, slower biologic incorporation, incomplete ligamentization, and possible immunogenicity.111–114 Several studies have reported high failure rates after allograft reconstructions when performed in young (<25 years) active patients and in those making an early often unsanctioned return to sport.38,45 The allograft source and graft preparation method may be important, with irradiated grafts demonstrating a higher early failure rate (33%) than nonirradiated grafts (2.4%).115 Recent systematic reviews by Carey and colleagues114 and Foster and colleagues,116 however, comparing outcomes after allograft and autograft reconstructions reported no significant differences in failure rates.
Most outcome studies investigating various autografts have failed to demonstrate a difference in graft failure rates. A systematic literature review by Spindler and colleagues23 showed no difference in failure rates between BTB and hamstring grafts and concluded that autograft type may not be the primary determinant for successful ACL reconstruction. This finding was supported by Magnussen and colleagues,117 who conducted a systematic review of studies with a minimum 5 years’ follow-up. They reported no difference in failure rates between those graft choices. A level 1 systematic review by Reinhardt and colleagues,118 however, which applied strict inclusion criteria and classified graft failure as those having undergone a revision procedure or with a documented positive pivot shift, reported a higher failure rate (15.8%) in the hamstring group compared with BTB reconstructions (7.2%).
Most hamstring double-bundle techniques recommend using the gracilis tendon to reconstruct the PL bundle.33,34,119 The size of this tendon is reported in the literature as between 5 mm and 7 mm in diameter. Most graft sizing sets, however, have a minimum diameter of 5 mm, which may introduce a degree of measurement error. The doubled gracilis tendon often slides easily through the 5-mm sizing cylinder; therefore, unless the gracilis tendon is tripled, it may often be less than 5 mm in diameter. This combination of inadequate tissue and increased in situ forces in the PL bundle may lead to increased PL bundle rupture rates. In support, Kondo and Yasuka,120 in a second-look arthroscopy study of double-bundle reconstructions where gracilis was used to create the PL bundle, graded the PL bundle as of intermediate or poor quality in 24% cases.
Failure to address concomitant factors
Failure to recognize and correct concomitant factors before ACL reconstruction may lead to increased tensile forces placed through the ACL graft, which may result in graft failure. Gersoff and colleagues121 reported unrecognized PL laxity in 10% to 15% cases of chronic ACL deficiency. Graft failure secondary to untreated ligamentous laxity was reported in 15% revision cases by Getelman and colleagues122 whereas in a recent study by the MARS Group at least 7% of technical errors were attributed to failure to treat malalignment or ligamentous laxity.69 The following factors have been recognized as important secondary stabilizers:
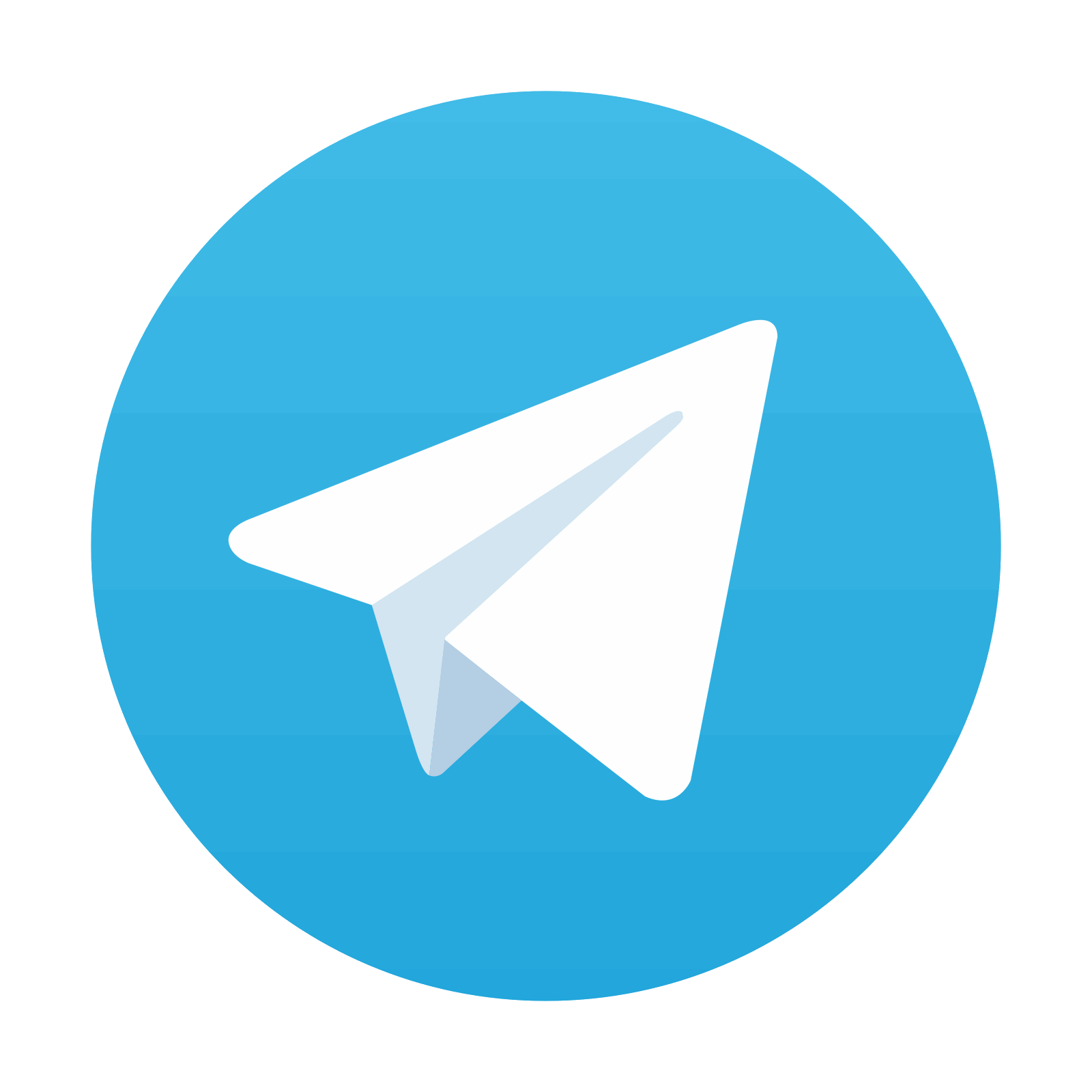
Stay updated, free articles. Join our Telegram channel

Full access? Get Clinical Tree
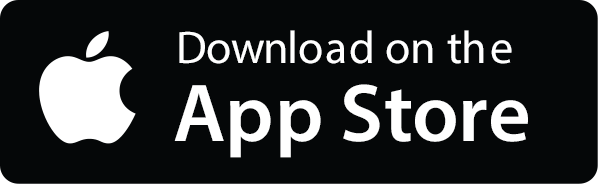
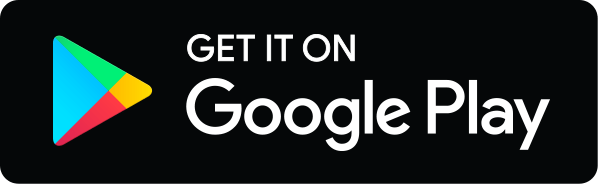