Fig. 9.1
Neutrophils in the skin. Schematic representation of molecular and cellular processes initiated during neutrophil antibacterial response in the dermis. The antibacterial response is initiated by sensing of DAMPs and PAMPs by resident cells in the interstitium (a) resulting in rapid recruitment of neutrophils from circulation via leukocyte adhesion cascade (b). These recruited neutrophils initiate multiple antibacterial responses (c) and activate endothelial cells for recruitment of monocytes and other immune cells (d). In addition to these functions, neutrophils can also transport microbial pathogens to draining lymph nodes via afferent lymphatics
Neutrophil recruitment into the dermis, as in other organs, occurs through postcapillary venules in a process that closely follows the steps of the classical ‘leukocyte adhesion cascade’ [64, 69, 96]. Under inflammatory conditions endothelial cells increase their expression of E- and P-selectins . This allows circulating neutrophils, which express P-selectin glycoprotein ligand-1, to interact with endothelial selectins, and results in neutrophil tethering and rolling along the blood vessel wall (Fig. 9.1b). Neutrophils are then able to interact with chemokines that are presented on the surface of endothelial cells. This triggers conformational changes in integrins, such as LFA-1 and Mac-1 , on neutrophils resulting in high affinity binding of integrins to ICAM1/2 on endothelial cells and, consequently, firm arrest (Fig. 9.1b). Additionally, under some experimental conditions, VLA-4 is also induced on neutrophils and this can interact with VCAM-1 expressed on the endothelium [60]. Thereafter, neutrophils crawl along the luminal surface of the venules in an integrin-dependent manner and search for a preferred point of transmigration. Focal deposits of chemokines along the blood vessel wall are thought to play a role in directed movement, although the precise mechanisms underlying this process are incompletely understood. Firmly adherent neutrophils then traverse the blood vessel wall and enter the dermal interstitial space. This involves negotiating multiple barriers. Initially, the penetration of the endothelial barrier requires neutrophils to either pass through the body of endothelial cells (transcellular pathway), or through the tight junctions between them (paracellular pathway). Upon reaching the subendothelial space, neutrophils migrate along pericyte processes in an integrin-dependent manner before exiting through gaps between pericytes [100]. These gaps become enlarged in response to cytokine stimulation via remodelling of basement membrane proteins [128, 131] and are associated with increased expression of integrin ligands and neutrophil-attracting chemokines on the pericyte [100].
Upon entering the dermis, neutrophils show strong directional migration towards gradients of chemotactic molecules emanating from the site of injury [79, 118]. Capillary and arteriolar pericytes have also been implicated in guiding neutrophils to the site of injury by upregulating ICAM-1 on the pericyte cell surface and releasing macrophage migration-inhibitory factor (MIF) [118]. Depending on the type of noxious insult, a vast array of chemotactic molecules may be present in the interstitium; although activation of endothelial cells and macrophages induces production of various chemokines, a large number of DAMPs and PAMPs emanate from damaged cells or pathogens at the site of injury. It is important to note that recent studies have shown the existence of positive feedback loops which augment the attraction of neutrophils towards the site of injury. For example, neutrophil sensing of nicotinamide dinucleotide (NAD+), which is released from dying cells and acts as a DAMP, has been shown to amplify the accumulation of neutrophils following sterile injury in the dermis [90]. Furthermore, neutrophil-derived leukotriene-B4 (LTB4) acts in a feedforward manner to increase the radius of attraction of the neutrophil population [67, 92]. The multiple chemoattracting cues provided to neutrophils in the interstitial space are integrated, and their migratory behavior is determined by a hierarchical arrangement of signalling patterns [79, 96]. For instance, signalling via formyl peptide receptor-1 supersedes signalling induced via chemokine receptor CXCR2 [79, 96]. Such a hierarchical organisation of signalling patterns assists in the guidance of neutrophils to the precise region of insult.
9.4 Neutrophil Functions at the Site of Tissue Injury
Neutrophils arriving from circulation need to neutralize invading microorganisms rapidly and clear tissue debris. This is achieved via particle uptake (phagocytosis), release of prestored granule contents, generation of reactive oxygen species (ROS), and the deployment of neutrophil extracellular traps (NETs ; Fig. 9.1c). Circulating neutrophils are transcriptionally inactive but their interaction with pathogens reactivates transcription and triggers the secretion of chemotactic factors that are crucial for recruitment of other immune cells [107]. The amount of chemokines or inflammatory mediators secreted per neutrophil is relatively small compared to other resident immune cells, but their significantly higher numbers at the site of injury compensate for this low expression [88].
9.4.1 Phagocytosis
During inflammation, neutrophils take up particulates such as microorganisms into membrane-bound vesicles (phagosomes) in a process termed phagocytosis, and particles are then destroyed inside these organelles. The initial process of uptake is strongly enhanced by soluble factors such as antibody or activated complement that mark (‘opsonise’) particulates for phagocytosis. Neutrophils express a range of Fc receptors, in particular FcγIIA and FcγIIIB [42], and aggregation of these receptors by antibodies that are presented in multimeric arrays, such as on microbial cell surfaces, causes neutrophil activation [84, 103]. Additionally, binding of complement-opsonized particles to CR3 (αMβ2-integrin/CD11b/Mac-1) leads to integrin ‘outside-in’ signalling and induction of phagocytosis [72]. Furthermore, phagocytosis can also be initiated nonopsonically through receptors that bind directly to microbial components, the details of which depend on the specific microorganism and the surface products that it expresses. In particular, recognition of microbial carbohydrate structures by neutrophil membrane receptors that contain lectin domains, such as dectin-1, Clec-2, or CR3 can mediate phagocytosis of a range of microorganisms [47, 63, 104]. Once internalised, the antimicrobial arsenal of the neutrophil is focused on the particulates by fusion of the phagosome with neutrophil granules.
9.4.2 Granule Composition and Release
The direct antimicrobial activity of neutrophils is largely due to the coordinated release of their granule cargo either into the extracellular milieu or the nascent phagosome. In both cases, high concentrations of antimicrobial proteins, as well as reactive oxygen species, are unleashed [2]. The formation of granules and synthesis of their contents occurs in the bone marrow and is tightly regulated during the development and maturation of neutrophils. A list of granules and their antimicrobial contents as well as the mechanism of action are detailed in Table 9.1. The release of these granules is a tightly regulated process that ensures safe delivery of their content only in close vicinity of pathogens, thereby limiting collateral damage to host cells. The mechanism regulating differential mobilization is incompletely understood though the role of intracellular calcium levels has been implicated in the process [110]. Another type of vesicle is secretory vesicles, which are not strictly granules, but are formed by endocytosis of plasma membrane during neutrophil maturation and contain proteins crucial for homing and sensing pathogens [2].
Granules | Antimicrobial agents | Mechanism of actions |
---|---|---|
Primary/azurophilic | Lysozyme | Degrades bacterial cell walls |
Myeloperoxidase | Generation of reactive oxygen species | |
Defensin | Disruption of membrane bilayers. Inhibition of DNA, RNA, protein and cell wall synthesis | |
Neutrophil elastase and cathepsin G | Cleaves virulence factors as well as membrane proteins | |
Bactericidal/permeability-increasing protein (BPI) | Increases bacterial permeability and hydrolysis bacterial phospholipids | |
Other proteins are azurocidin, silaidase, β- glucuronidase | ||
Secondary/specific | Lysozyme | Degrades bacterial cell walls |
Lactoferrin | Binds to iron rendering it unavailable for bacterial absorption and utilization. Increases membrane permeability by binding to LPS | |
Other proteins are Gp91phox/p22phox, hCAP 18, CD11b, collagenase, NGAL, B12BP, SLPI, haptoglobin, pentraxin 3, oroscomucoid, heparanase, β2 microglobulin, CRISP3 | ||
Tertiary/gelatinase | Gelatinase | Metalloprotease and can degrade extracellular matrix to increase neutrophil migration |
Other proteins are Gp91phox/p22phox, CD11b, MMP25, arginase-1, β2 microglobulin, CRISP3 | ||
Secretory vesicles | Complement receptor 1 | Phagocytosis of complement opsonized microbes |
FcγRIII (CD16) | Phagocytosis of immunoglobulin opsonized microbes | |
Other proteins are Gp91phox/p22phox, CD11b, MMP25, C1q-R, FPR, alkaline phosphatase, CD10, CD13, CD14, plasma proteins |
Although neutrophils possess a striking range of granule proteins, their biological activities can be summarised largely based on their mechanism of antimicrobial action [2]. The first group includes cationic proteins that bind directly to bacterial membranes and mediate lysis; these include α-defensins [44], LL37 [125], and bactericidal/permeability-increasing protein (BPI) [68]. In contrast, a number of proteins with enzymatic activity, such as lysozyme [44], neutrophil elastase (NE) [8, 124], and cathepsin G [124] exert antimicrobial activity through enzymatic degradation of microbial products. The products targeted by these enzymes may be essential components of the cell itself (e.g. lysozyme M activity against the Micrococcus luteus peptidoglycan cell wall [45]; neutrophil elastase-mediated degradation of the Escherichia coli outer membrane protein OmpA [8]), or may represent extracellular virulence factors (e.g. neutrophil elastase-mediated degradation of S. flexerni secreted proteins/toxins [132]). Lastly, antimicrobial activity may also be mediated by sequestration of essential nutrients; a key illustration of this is the multiple approaches used by neutrophils to limit iron availability. Lactoferrin, a component of secondary granules, has direct iron-binding activity, which limits free iron available to growing microorganisms [78, 91]. Alternatively, lipocalin (NGAL) is able to bind to a range of bacterial siderophores and inhibits bacterial iron uptake [39]. These multiple proteins act in concert with reactive oxygen species, which are also generated during degranulation, and exert potent microbicidal activity [89].
The second major antimicrobial component of the degranulation response is the production of reactive oxygen species (reviewed in [7, 32, 134]). The assembly of functional NADPH oxidase (phagocytic oxidase/phox) enzyme from individual components is the initiating step in ROS formation. Under resting conditions, Gp91phox and p22phox reside within secondary granules and the other key components of the enzyme, including p47phox, p67phox, and p40phox and the small GTPase rac2, are present within the cytoplasm. Upon neutrophil activation, the cytoplasmic components are translocated to the phagosome that has formed by the fusion of the plasma membrane and granules (or to the plasma membrane), thereby bringing all components of the enzyme together [7, 134]. On becoming functional, NADPH oxidase catalyzes the key initial step in the generation of ROS, which is the conversion of molecular oxygen (O2) to superoxide (2O2 −). Once formed, superoxide rapidly dismutates to hydrogen peroxide (H2O2) and this is acted upon by myeloperoxidase (MPO) , which is also released into the phagosome during degranulation, to form hypochlorous acid (HOCl−). In addition, a range of oxidant species including chloramine, hydroxyl radicals (OH•), singlet oxygen (1O2), and ozone (O3) is formed [127]. Although all of the different ROS produced have varying degrees of antimicrobial activity, hypochlorous acid is particularly potent at causing oxidative damage to most biological molecules. It is generally believed that this powerful oxidation is one of the key mediators of neutrophil antimicrobial activity [32].
9.4.3 Neutrophil Extracellular Traps (NETs)
Neutrophils can also ‘ensnare’ pathogens by releasing DNA-based molecular traps (referred to as neutrophil extracellular traps or NETs ). These released DNA NETs are associated with histones, myeloperoxidase, neutrophil elastase, lactoferrin, pentraxin-related protein 3 (PTX3), matrix-metalloproteases (MMPs), and other granule proteins all of which can orchestrate antimicrobial functions [15, 16, 137]. DNA NETs can act as molecular scaffolds that entrap pathogens and enhance their interaction with microbicidal proteins. Although neutrophils mostly expel genomic DNA for NET formation [15], mitochondrial DNA can also be used for this process [139]. The process of NET formation is generally considered to be ‘suicidal’ and is also referred to as ‘NETosis ’, similar to other cell death mechanisms such as apoptosis, necrosis, and pyroptosis [16, 137].
The molecular processes required for NET formation are incompletely understood. It has been shown that activation of NADPH oxidase and subsequent generation of ROS is crucial for NET formation [15, 40, 80]. Upon ROS generation, the granule enzymes NE and MPO translocate to the nucleus and degrade histones [93]. Uncondensed nuclear material is then released by perforation of the cell membrane leading to cell lysis [15, 16, 137]. Furthermore, there is increasing evidence for a second type of NETosis that does not require cell membrane perforation and lysis but instead uses vesicular exportation of DNA for release, referred to as ‘vital NETosis ’ [137]. The released ‘anuclear’ neutrophil cytoplast retains pathogen-sensing, chemotactic migration, and phagocytosing capabilities [138]. These differences between ‘suicidal’ and ‘vital’ forms of NETosis could be due to differences in the activating signal or in the ‘maturity’ of neutrophils. Nonetheless, both forms of NETosis are important for mediating neutrophil antibacterial responses [137].
9.5 Neutrophil Recirculation and Transport of Antigen
Increasing evidence suggests that neutrophils may enter peripheral tissues, including the skin, even under noninflammatory conditions, and that they may traffic into lymphatic vessels to draining lymph nodes both during steady state and inflammation. During their classic lymphatic cannulation studies in sheep, Morris and colleagues observed neutrophils in lymph fluid, in both the lymphatics draining the hind limb [52] and in the efferent lymph of the popliteal lymph node [50]. It was, however, not until the advent of multiphoton microscopy that such ‘scouting’ neutrophils were directly observed within the skin during steady state [90]. Labeled neutrophils intravenously injected into sheep could be recovered from the lymphatics within 3–4 h of intravenous injection, indicating that neutrophil recirculation from the blood into the lymph occurs rapidly, in contrast to the delayed kinetics (6–20 h) of lymphocytes [90]. Although it remains uncertain what proportion of neutrophils recirculates under physiological conditions, a clear consequence of this phenomenon is that, in addition to their role as rapid responders, neutrophils may also serve as a primary sensor population for damage and infection [90].
In addition to this physiological recirculation, numerous studies have reported the trafficking of pathogen-bearing neutrophils from the site of infection to draining lymph nodes, including from the skin to cutaneous lymph nodes [1, 11, 73, 121, 136], and that such migration facilitates antigen delivery for ongoing adaptive immune responses [1, 6, 121]. Only a relatively small proportion of recruited neutrophils appears to traffic into draining lymphatics [121], and it remains unclear what differentiates this population from the majority that remain and die within the infected tissue. Similarly, the cellular and molecular pathways that regulate neutrophil entry into the lymphatics remain largely unknown. Although it has been reported that neutrophil migration to the lymph node is CCR7-dependent [5], the potential role for other chemokine receptors has yet to be investigated. CXCR2 and CXCR4 are of particular interest, given their well-documented role in coordinating neutrophil trafficking from the bone marrow [35, 76]. Moreover, CXCR2 has already been implicated in neutrophil trafficking directly into the lymph node from the blood during inflammation [14], whereas CXCR4 is upregulated on the surface of neutrophils following their extravasation into inflammatory tissue [135].
9.6 Neutrophil Apoptosis and Resolution of Inflammation
Neutrophils are generally regarded as pro-inflammatory cells but a large body of evidence indicates that they also have a crucial role in resolving inflammation. During the resolution phase, due to their phagocytic activity, neutrophils remove cellular debris from the site of injury, paving the way for tissue repair [64]. MMP-9 released by neutrophils can degrade various intracellular cytoskeletal components released from damaged cells, thereby clearing DAMPs [64], and can also promote vascularisation of tissue by activating vascular endothelial growth factor (VEGF) [9, 25]. Neutrophils also initiate recruitment of monocytes via activating endothelial cells to produce CCL2 or by releasing azurocidin, LL37 and cathepsin G that can activate monocyte formyl peptide receptors (FPRs) (Fig. 9.1d) [114, 116, 117]. These monocytes then phagocytose apoptotic neutrophils during tissue resolution.
Although neutrophils are short-lived cells, factors released at the site of injury can enhance their viability. For example, the presence of LPS or GM-CSF increases the expression of antiapoptotic proteins Mcl-1 and A1, thereby leading to an extended neutrophil lifespan (detailed in [2, 62]). In contrast, the neutralisation of noxious insults and microbes leads to decreased signalling from these pathways and results in increased neutrophil apoptosis. At the initial stages of apoptosis neutrophils secrete ‘find me’ signals to guide monocytes and macrophages to the site of apoptosis and later display ‘eat me’ signals for rapid phagocytic uptake [115]. Phagocytosis of apoptotic neutrophils by tissue-resident macrophages results in decreased production and release of IL-23 leading to decreased IL-17A levels. The reduced IL-17A results in less secretion of G-CSF by bone marrow stromal cells, decreased granulocytopoiesis, and increased retention of mature neutrophils in the bone marrow [119].
Soluble lipid mediators are important both in neutrophil recruitment and in termination of the acute inflammatory response. At early stages of inflammation, prostaglandins (prostaglandin I2) and leukotrienes (LTB4) are produced, resulting in rapid recruitment of neutrophils, but as inflammation progresses, a molecular switch in lipid metabolism results in production of lipoxins, resolvins, and protectins [2, 64, 111]. These mediators assist in monocyte influx and suppress neutrophil recruitment and antimicrobial functions. In addition, lipid mediators result in nonphlogistic uptake of apoptotic cells by monocytes and macrophages resulting in IL-10 and TGF-β production. Together, these processes promote the resolution of inflammation and tissue restitution.
9.7 Neutrophil Deficiencies
Deficiencies in either the absolute number of circulating neutrophils or their correct functioning typically lead to enhanced susceptibility to infection, particularly within the skin. This is highlighted in acquired neutropenia, such as in patients undergoing chemotherapy, where the severity of neutrophil deficiency is an important determinant of susceptibility to infection with many bacterial and fungal pathogens [10, 74]. A number of molecular deficits that affect neutrophil development or release from the bone marrow can lead to primary neutropenia or functional defects (reviewed in [34]). Such genetic deficiencies include: chronic granulomatous disease (CGD) or MPO deficiency in which ROS generation is affected [54, 127], compromised granule formation/function (e.g. Chédiak–Higashi syndrome) [4], or leukocyte adhesion deficiency syndromes, which result in decreased neutrophil recruitment [51]. Furthermore, defects in cytokine pathways that contribute to the recruitment of neutrophils also manifest as increased susceptibility to cutaneous infection. For example, mutations that lead to either diminished IL-17 production or IL-17 signalling, including in IL17RA, TYK2, STAT3, or STAT1, predispose to chronic mucocutaneous candidiasis [83, 101, 126]. Taken together, the increased susceptibility to infection, particularly to a subset of microorganisms that includes Staphylococcus aureus, Pseudomonas aeruginosa, Candida albicans, and Aspergillus spp, highlights the critical importance of neutrophils in protection from these pathogens.
9.8 Neutrophilic Dermatoses
Although neutrophils are central to the acute inflammatory response that is initiated following tissue damage or pathogen entry, in some individuals inappropriate recruitment and activation of these cells may occur. This can then contribute to pathology in the absence of an identifiable cause. In some diseases, for example, psoriasis, neutrophils are found in large numbers, but it is unclear whether this represents an epiphenomenon or a true pathogenic event [95, 123]. Alternatively, autoimmune conditions such as lupus erythematosus, cutaneous vasculitides, and neutrophilic dermatoses are prominent examples of conditions where neutrophils appear to contribute to pathology. In vasculitides, the skin can either be the primary organ of involvement, for example, in small vessel leukocytoclastic vasculitis, or part of systemic syndromes, such as in Wegener’s granulomatosis where autoantibodies are directed against neutrophils [19, 59]. These conditions have diverse aetiology and their detailed discussion is beyond the scope of this chapter.
Neutrophilic dermatoses are a set of clinical entities that share the common feature of neutrophil influx within the skin in the absence of infection. Several conditions are recognised, but those most commonly described are Sweet’s syndrome (SS), pyoderma gangrenosum (PG), and subcorneal pustular dermatosis (SCD; reviewed in [28]). In SS and PG, neutrophil influx occurs predominantly within the dermis, although in PG it may extend to subcutaneous tissues. Variable influx of other inflammatory cell populations, including eosinophils, lymphocytes, and giant cells may also occur depending on the subclassification. In contrast, in SCD neutrophils are confined primarily to the subcorneum [27, 28, 105].
The underlying aetiology of neutrophilic dermatoses is unclear; however, there are a number of striking disease correlations. These include, depending on the classification: prior infections, drug treatment, inflammatory bowel disease, autoimmune conditions, and malignancies [27, 28]. A number of lines of evidence implicate Th1 and/or Th17 inflammatory cytokines in mediating disease, at least in SS and PG. Observations supporting Th1 involvement include increased serum IL-2 and IFNγ [46], whereas elevated levels of IL-1, IL-3, IL-6, and IL-8 have also been noted [27, 77]. A clear pro-pathogenic role for TNF, in particular, has been reported, and a number of studies have shown the benefit of anti-TNF therapy [17, 28]. More recently the expression of IL-17 and/or IL-23 in SS and PG has been reported, suggesting potential involvement of this cytokine axis in pathology [38, 49, 77]. Consistent with this, case studies that report the successful use of anti-IL-12/23 therapy for the treatment of PG have begun to emerge [37, 49]. Whether this cytokine–disease link holds true in larger studies remains to be seen.
9.9 Neutrophils During Cutaneous Infection: S. aureus as a Model Pathogen
The distinct subset of pathogens that cause disease in individuals with neutrophil deficiencies suggests that there are shared protective pathways involved in the responses to these species. In the following section we use the major human pathogen S. aureus as an example to illustrate the role of neutrophils in cutaneous immunity.
Data from clinical, rodent, and in vitro studies show remarkable agreement in support of the essential role of neutrophils in protection from S. aureus. As described in Sect. 9.7 above, a range of defects in either neutrophil number or function is associated with increased susceptibility to cutaneous S. aureus infection. Note that this is mirrored in rodents, as mice deficient in NADPH-oxidase components develop spontaneous cutaneous lesions, or fail to control experimental infection [36, 57, 99]. Moreover, depletion of circulating neutrophils prevents control of cutaneous S. aureus infection [85]. In vitro, phagocytosis of S. aureus by neutrophils is enhanced by serum opsonins [3, 61, 94], and their subsequent killing is mediated by the NADPH-oxidase dependent production of ROS [36, 75]. A number of granule proteins have direct anti-Staphylococcal activity in vitro. These include the α-defensins HNP1-3, as well as LL-37, PG-1, S100A8/9, and lactoferrin [21, 29, 125], whereas others, including azurocidin and lysozyme are ineffective [21]. Lastly, extracellular DNA NETs are released by neutrophils in response to S. aureus. The NETs are capable of trapping bacteria, and the NET-associated histones and granule proteins then mediate killing [15, 40, 98].
Rodent models have been particularly informative in identifying the pathways that lead to neutrophil recruitment. Initial recognition of S. aureus infection by resident immune sentinel cells occurs via TLR2, NOD2, and the NLRP3 inflammasome [55, 82, 112], resulting in the induction of pro-inflammatory cytokines. Dominant amongst the initial cytokines produced are IL-1β [81, 82] and IL-17A/F [23], both of which have been shown to drive neutrophil accumulation and activation in a range of disease models [65, 113]. Both humans [97, 129] and mice [22, 24, 81] with deficits in the TLR/IL-1β signalling pathway control S. aureus infection poorly. The initial source of IL-1β in the skin is unclear: whereas inflammasome-dependent IL-1β release in response to S. aureus in vitro is typically associated with monocyte/macrophages [30, 86, 112], in vivo much of the IL-1β produced appears to be derived from recruited neutrophils, at least during later stages [22]. As with IL-1β, deficiencies in IL-17 signalling (mutations in STAT3 and TYK2) also predispose to S. aureus infection [23, 33, 48, 56, 83, 87]. This IL-17A and IL-17F is produced by skin resident γδ-T cells in an IL-23–dependent manner, and during S. aureus infection γδ-T-cell–deficient mice show decreased neutrophil recruitment [23]. Ultimately, the combination of IL-1β and IL-17A/F stimulates local production of CXCL1 and CXCL2 from resident cells. These chemokines specifically recruit neutrophils to the infection site, and under normal circumstances this results in bacterial clearance [22, 23, 81, 82].
9.10 Concluding Remarks
The essential role of neutrophils as a key player in the innate immune response to injury/infection within the skin is well recognised, however, it is becoming clear that this is only one aspect in their short life span. Neutrophils under homeostasis perform myriad functions ranging from removing damaged endothelial cells [18] to regulating the stem cell pool in the bone marrow [20]. In particular, the identification of ‘scouting’ neutrophils that are capable of sensing the presence of tissue damage [90], and the finding that they can recirculate [50, 52, 90] and influence the development of immune responses is furthering our understanding of skin immunity. Although these findings suggest that neutrophils may have previously unrecognised functions in the initiation of both innate and adaptive immune responses, the fate of these neutrophils in the lymph nodes is not completely understood. Moreover the presence of these ‘scouting’ neutrophils in uninflamed dermis challenges our current understanding about neutrophil recruitment in the skin. Taken together, the increasingly apparent complexity of neutrophil biology argues that continued investigation into this often underestimated cell is likely to lead to improved strategies for treatment of a broad range of cutaneous diseases.
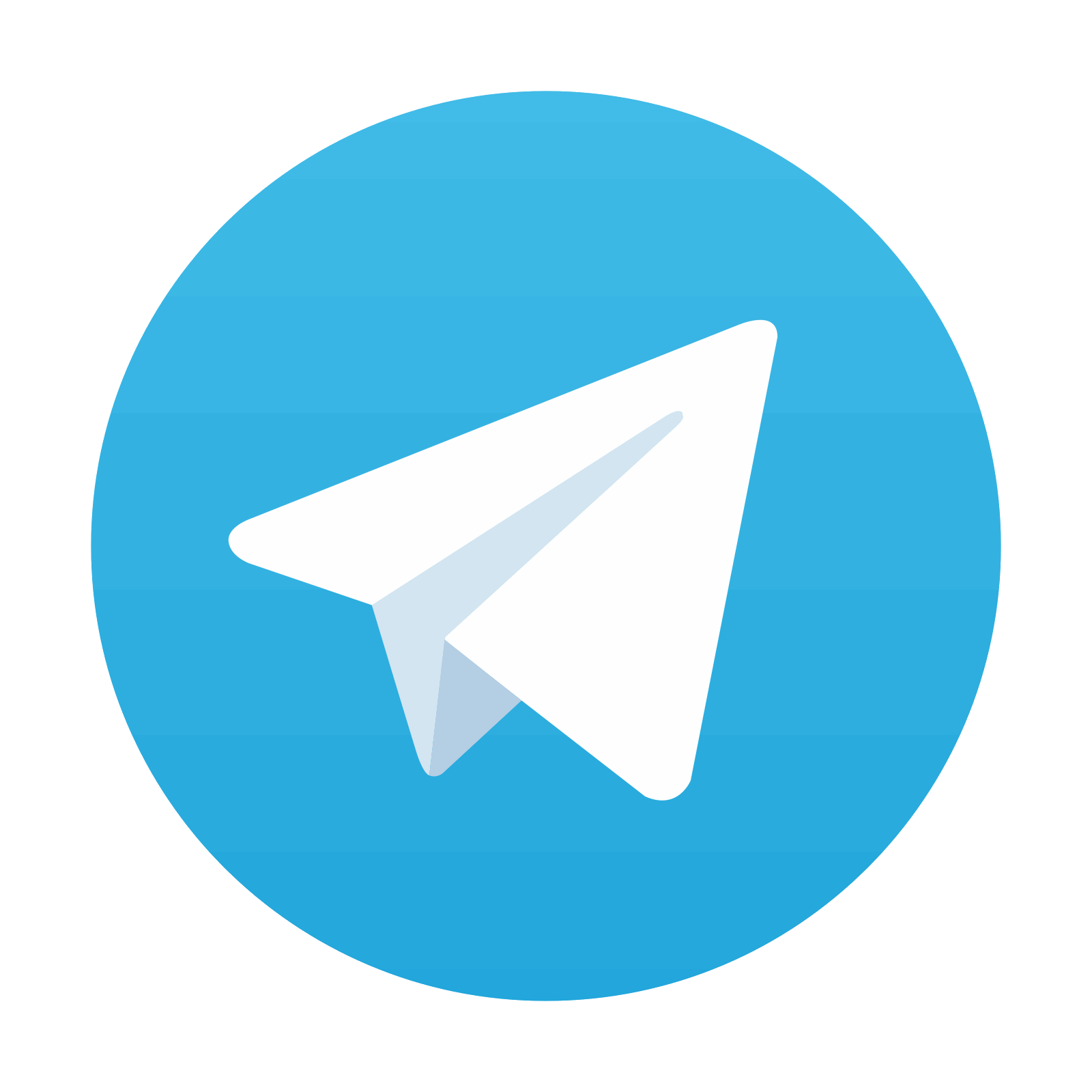
Stay updated, free articles. Join our Telegram channel

Full access? Get Clinical Tree
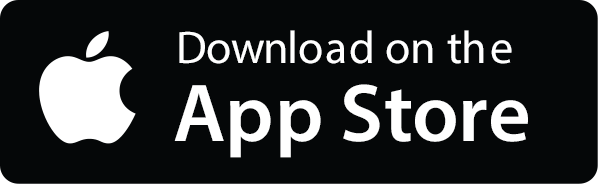
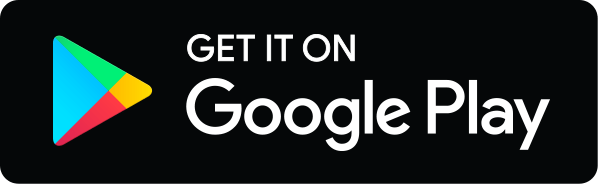