Fig. 18.1
(a) Optical appearance and cryo-TEM image of a translucent nanoemulsion (Reprinted from Sonneville-Aubrun et al. (2004), p. 146, with permission of Elsevier). (b) Cryo-TEM image of nano-sized oil droplets (homogeneously filled circles) and vesicles (unfilled circles); the scale bar represents 200 nm (Reprinted from Norden et al. (2001), p. 400, with permission of Elsevier). (c) Freeze-fracture TEM micrograph of an amphotericin B nanoemulsion (Reprinted from Benita and Levy (1993), p. 1078, with permission of Wiley). (d) Nanoemulsion morphology visualised by atomic force microscopy (Reprinted from Marxer et al. (2011), p. 433, with permission of Elsevier)
Apart from analysing droplet size distributions, the droplet surface charge as an indicator for electrochemical stabilisation can be determined by laser Doppler electrophoresis (Mueller 1996). Stability investigations are usually performed by monitoring parameters such as the mean droplet size, the polydispersity index, droplet surface charge and pH value of the nanoemulsions in question. In our experience, the physical stability of classical nanoemulsions produced by high-pressure homogenisation can be expected to be around 2 years or longer at slightly refrigerated storage. However, the chemical stability of individual compounds has to be taken into account as well. Oil, surfactants and incorporated actives may be subjected to chemical degradation by oxidation or hydrolysis, potentially leading to an unpleasant optical and olfactory appearance despite unchanged droplet size (Wabel 1998; Baker and Naguib 2005). Optical monitoring of the formulations, assessment of drug stability and monitoring of both zeta potential and pH are useful tools in detecting such changes (Klang et al. 2011a).
18.1.2 Dermal Application: Advantages and Limitations
Most marketed nanoemulsion products can be found in the realms of intravenous nutrition and drug delivery as well as in dermal drug delivery and cosmetics. In terms of dermal drug delivery, nanoemulsions offer distinct advantages: high physical stability compared to conventional emulsions, high skin friendliness due to the low amount and the mild nature of the employed surfactants and last but not least the ease of preparation and scale-up. They avoid the limitations of other colloidal drug delivery systems, such as the limited drug loading and stability issues of liposomes and the potentially irritating compounds required for the production of nanoparticles or microemulsions. Depending on composition and the nature of the employed drug, nanoemulsions may achieve higher rates of skin penetration and drug accumulation within the skin than lipid nanoparticles (Calderilla-Fajardo et al. 2006). In a recent study investigating the dermal delivery of lutein, a more rapid release in case of nanoemulsions was found, which achieved higher skin permeation rates than nano-structured lipid carriers and solid lipid nanoparticles (Mitri et al. 2011).
Classical nanoemulsions prepared by high-pressure homogenisation are usually stabilised by skin-friendly surfactants such as lecithins and do not require synthetic surfactants. Thus, skin lipids are not washed out during cleaning. Lipophilic drugs can be incorporated into the oil phase according to their solubility. The release of the drugs from the system is generally acknowledged to be quite rapid; however, attempts towards retarded or controlled release as well as site-specific targeting have been reported as well (Yang and Benita 2000; Eskandar et al. 2009). Nanoemulsions support the penetration of incorporated actives into the skin and may thus promote their accumulation in the skin. In addition, the cosmetic effect of the basic vehicles is of further interest. In recent approaches, the possibility to incorporate hydrophilic drugs into nanoemulsion systems is being investigated with the aim of promoting the incorporation efficiency, stability and penetration of water-soluble actives (Anton et al. 2010; Schwarz et al. 2012).
Retarded drug delivery from nanoemulsions is difficult to achieve because of the low viscosity of the systems. When compared to aqueous solutions or dispersions, retarded drug release from nanoemulsions may of course be observed. In general, a retarded release may be achieved for very lipophilic drugs with high affinity to the oil phase.
From another viewpoint, the low viscosity of nanoemulsions is an advantageous feature: it renders them attractive systems for development of aerosol sprays such as sunscreens that show no phase separation during storage. If a higher viscosity is required, modification of the nanoemulsion is possible by incorporation of gelling agents. Successful reports on the development of thickened nanoemulsions can be found in the literature (Alves et al. 2005; Mou et al. 2008).
Regarding transdermal penetration, there is a certain amount of recent literature proposing nanoemulsions for this task. However, a closer look at these articles reveals that the systems in question are exclusively dealing with thermodynamically stable microemulsion phases. Nanoemulsions in the sense of skin-friendly submicron-sized emulsion systems are not specifically designed for the purpose of delivering drugs transdermally. To allow for the passage of intact nanovectors across pores of the skin, much smaller and deformable carriers are required since the skin is a strong and complex barrier (Cevc and Vierl 2010). Small drug amounts may of course be found in deeper skin layers after application of a nanoemulsion system due to mechanisms such as penetration across hair follicles (Lademann et al. 2001) or a general penetration enhancement caused by the involved excipients, in particular the involved lecithin phospholipids (Yu et al. 2009). In general, however, nanoemulsions are not employed for transdermal drug delivery, but rather for targeting the outermost skin layers, for instance, in case of fungal infections or inflammation. The versatile nature of nanoemulsions is highlighted by another recent study by Spagnul et al., who designed a calixarene nanoemulsion aimed at chelating uranium molecules at the skin surface (Spagnul et al. 2011). The patented formulation is a promising approach towards treating cutaneous uranium contamination if the formulation is applied quickly. This study shows that nanoemulsions exhibit advantageous properties for different applications on skin and can be tailor-made according to the envisioned task.
In another recent study, a lecithin-based nanoemulsion containing 5-aminolevulinic acid (5-ALA) was developed for dermal application in photodynamic therapy (Maisch et al. 2010). Significantly deeper skin penetration was found for the nanoemulsion when compared to a conventional creamy emulsion (Fig. 18.2). As intended in localised therapy, no penetration beyond the basal cell membrane was observed. The increased drug transport into the epidermis may be ascribed to a stabilising effect of the nanoemulsion on 5-ALA, thus preventing dimerisation. Furthermore, the nanoemulsion formulation seemed to support the cellular uptake of 5-ALA. Recently, the pivotal phase III studies were completed for the developed system. The results support the claims of improved stability and skin penetration when tested against a conventional cream and placebo (Dirschka et al. 2012). The corresponding product has successfully been marketed (Ameluz®, Biofrontera Pharma GmbH).


Fig. 18.2
Distribution of protoporphyrin IX after application of a nanoemulsion-based formulation containing 10 % 5-ALA hydrochloride (left column) or a commercial cream containing 16 % w/w aminolevulinate methyl ester hydrochloride (right column). Scale bars represent 100 μm. The images were taken after incubation of the skin with the respective formulation. The blue line represents the basal membrane, i.e. the border between epidermis and dermis. Samples were removed after 3, 8 and 12 h, and the induction of protoporphyrin IX was determined (coloured areas) (Image reprinted from Maisch et al. (2010), p. e304, with permission of Wiley)
Regarding the mechanism of nanoemulsion penetration, it can be summarised that no evidence exists that conventional colloidal systems such as nanoemulsions may penetrate into the skin as intact structures. It may thus be assumed that their skin penetration is related to penetration of the system into shunt pathways such as hair follicles, accumulation of the system between corneocyte clusters or in furrows to interact with skin lipids or merging of the system into extended lipidic structures on the skin surface. In this context, penetration enhancement of drugs may be caused by the occlusion of the skin surface (Eskandar et al. 2009; Zhou et al. 2010) as well as the interaction of nanoemulsion excipients such as phospholipids with skin compounds. The systems may alter the thermodynamic activity of incorporated drugs on the skin surface as well (Cevc and Vierl 2010). According to their composition, nanoemulsions may thus be employed to enhance the penetration of incorporated actives into the skin. Different aspects may influence the success of this strategy, such as the exact formulation morphology, the droplet surface charge and the nature of the involved compounds. The role of these factors will be discussed in the following sections.
18.2 Penetration Enhancement Strategies
18.2.1 Role of Droplet Size
The large surface area and low surface tension of small nanoemulsion oil droplets has been described to improve skin interaction and thus dermal drug delivery (Benita 1999; Klang et al. 1998). An enhanced local therapeutic effect of incorporated drugs due to prolonged residence time in the uppermost skin layers may thus be expected. One of the earliest studies dealing with the effect of nanoscale droplet size in topical emulsions was conducted by Friedman et al. (1995). Both steroidal and nonsteroidal antiinflammatory drugs including betamethasone valerate and dipropionate, naproxen, diclofenac, indomethacin and piroxicam were incorporated into nanoemulsions and regular creamy emulsions of identical composition. The antiinflammatory efficacy of the systems was compared against each other and to established marketed formulations by using the carrageenan-induced paw edema rat model. Significantly improved antiinflammatory efficacy was found for the nanoemulsion systems, especially in case of indomethacin, diclofenac and betamethasone esters. Noticeable systemic activity of antiinflammatory drugs formulated in nanoemulsions was observed as well. Preliminary in vivo studies on human volunteers showed good acceptability and comparable properties to marketed products. The authors hypothesised that lipid disruption within the stratum corneum caused by the nanoemulsion phospholipids, the formation of gaps and the increased swelling of the skin may favour the penetration of lipid droplets with diameters below 100 nm. Under these circumstances, shunt pathways such as hair follicles or sebaceous channels may lead to enhanced penetration as well. It should be kept in mind, however, that these conditions may be particularly encountered in the employed edema model. In a more recent study, Kotyla and co-workers compared the bioavailability of tocopherol from a nanoemulsion and a micrometre-sized emulsion of the same composition. After dermal application of the nanoemulsion in vivo on golden hamster skin, a 2.5-fold increase in plasma tocopherol levels was determined (Kotyla et al. 2008).
These studies held aside, literature about the role of droplet size on the skin penetration of otherwise identical emulsions is scarce. However, by experimenting with certain sucrose ester surfactants with peculiar gelling behaviour, we were able to create both fluid nanoemulsions and semisolid emulsions of identical composition. When evaluating the skin penetration potential of these systems in vitro (Klang et al. 2011b) and in vivo (Klang et al. 2012b), we found a highly similar penetration behaviour irrespective of the respective droplet size distribution. This may however be related to the specific nature of the developed emulsions, in particular the hydrophilic gel network of the semisolid emulsions. For aqueous dispersions based on the same network, the microviscosity and thus the drug transport were found to be comparable to that of corresponding fluid nano-sized systems (Ullrich et al. 2008). For the developed sucrose stearate emulsions, droplet sizes around 120–150 nm did not significantly affect the skin penetration of incorporated drugs when compared to corresponding emulsions with droplet sizes in the micrometre range. Further comparative studies in this direction using nanoemulsions with droplet sizes below 100 nm would be of interest to gain further information on this matter.
In another recent study, lecithin nanoemulsions for topical delivery were developed using snake oil, soybean lecithin, glycerol and purified water (Zhou et al. 2010). When applied in an O/W cream, the nano-sized droplets were shown to enhance skin hydration and skin penetration of the incorporated model dye nile red into the dermis when compared to a control O/W cream. The authors observed increased skin adhesion of the nanoemulsion formulation to the skin surface and subsequent formation of an occlusive film, thus increasing skin hydration and consequently skin penetration of the model dye. The increased skin penetration can therefore be ascribed to physical effects caused by the presence of small oil droplets. In addition, the authors assumed that an increased partitioning of lecithin molecules into the stratum corneum might be responsible for subsequent changes in the barrier properties, i.e. that the observed effects could be ascribed to the employed nanoemulsion compounds.
Another study reported increased in vitro skin permeation rates of camphor, menthol and methyl salicylate through rat skin when the substances were applied in form of a hydrogel-thickened nanoemulsion instead of a control gel (Mou et al. 2008). The authors speculated that different factors, e.g. high concentration gradients, formation of drug reservoirs or embedding of the small oil droplets within the stratum corneum lipids, might account for the observed enhancement effect.
In another recent study, penetration enhancement of glycyrrhetic acid incorporated into a nanoemulsion was observed in vitro when compared to a conventional oil-in-water emulsion of different composition (Puglia et al. 2010). In addition, an increased antiinflammatory activity in vivo was observed for the nanoemulsion. However, the authors stated that the nature of the employed excipients for the different nanoemulsion and emulsion systems may have influenced the obtained results. This again confirms that the beneficial effects obtained with nanoemulsion depend not only on the droplet size but on the nature of the developed systems as well as the employed compounds.
18.2.2 Role of Droplet Surface Charge
The surface charge of nanoemulsion oil droplets in dispersion, often described by the zeta potential, is an important aspect that may strongly affect the interaction between formulation and skin and drug penetration. A positive droplet surface charge has been observed to enhance penetration of drugs into the nasal and ocular mucosae as well as the stratum corneum of the skin (Yang and Benita 2000; Mou et al. 2008). A high absolute surface charge of the nanoemulsion droplets is also a crucial prerequisite to ensure physical stability upon storage. From this viewpoint, a high positive surface charge may fulfil both the tasks of enhancing stability and promoting skin penetration.
A positive zeta potential can be induced by using cationic excipients such as lipids, polymers and surfactants, which can result in an improved interaction with negatively charged membranes such as the skin or corneal membrane. Negatively charged protein or fatty acid residues of the skin as well as the presence of selective active ion pumps render the skin surface selective to positively charged substances (Yang and Benita 2000; Piemi et al. 1999). It has been established by Benita and co-workers that positively charged nanoemulsions exhibited better wettability on the cornea compared to saline or negatively charged systems (Yang and Benita 2000). In dermal drug delivery, the same strategy was followed in several studies. Piemi et al. found that the in vitro penetration of econazole and miconazole nitrate into rat skin was higher from positively charged nanoemulsions containing stearylamine than from negatively charged formulations containing deoxycholic acid (Piemi et al. 1999). The binding of surfactants to the skin can be attributed to non-specific hydrophobic interactions with keratin as well as to specific electrostatic interactions in case of charged surfactants; the latter phenomenon is strongly dependent on the pH of formulation and skin. The obtained results suggest that the surface charge of nanoemulsion droplets may affect skin penetration of drugs due to increased interaction with the target site. Similar results were reported in subsequent studies for stearylamine (Fang et al. 2004) and phytosphingosine (Hoeller et al. 2009; Klang et al. 2010), although the observed enhancement effects were not as pronounced in case of the latter. Baspinar and co-workers likewise employed phytosphingosine to produce a positively charged nanoemulsion for dermal application of prednicarbate. Higher drug release was observed for negatively charged nanoemulsions in vitro when using diffusion cells and a synthetic model membrane. However, significantly higher amounts of the active ingredient were found to penetrate into the skin from the positively charged nanoemulsion when using excised human skin (Baspinar and Borchert 2012). These results suggest that a positive surface charge in nanoemulsions may indeed be of value for in vivo skin penetration.
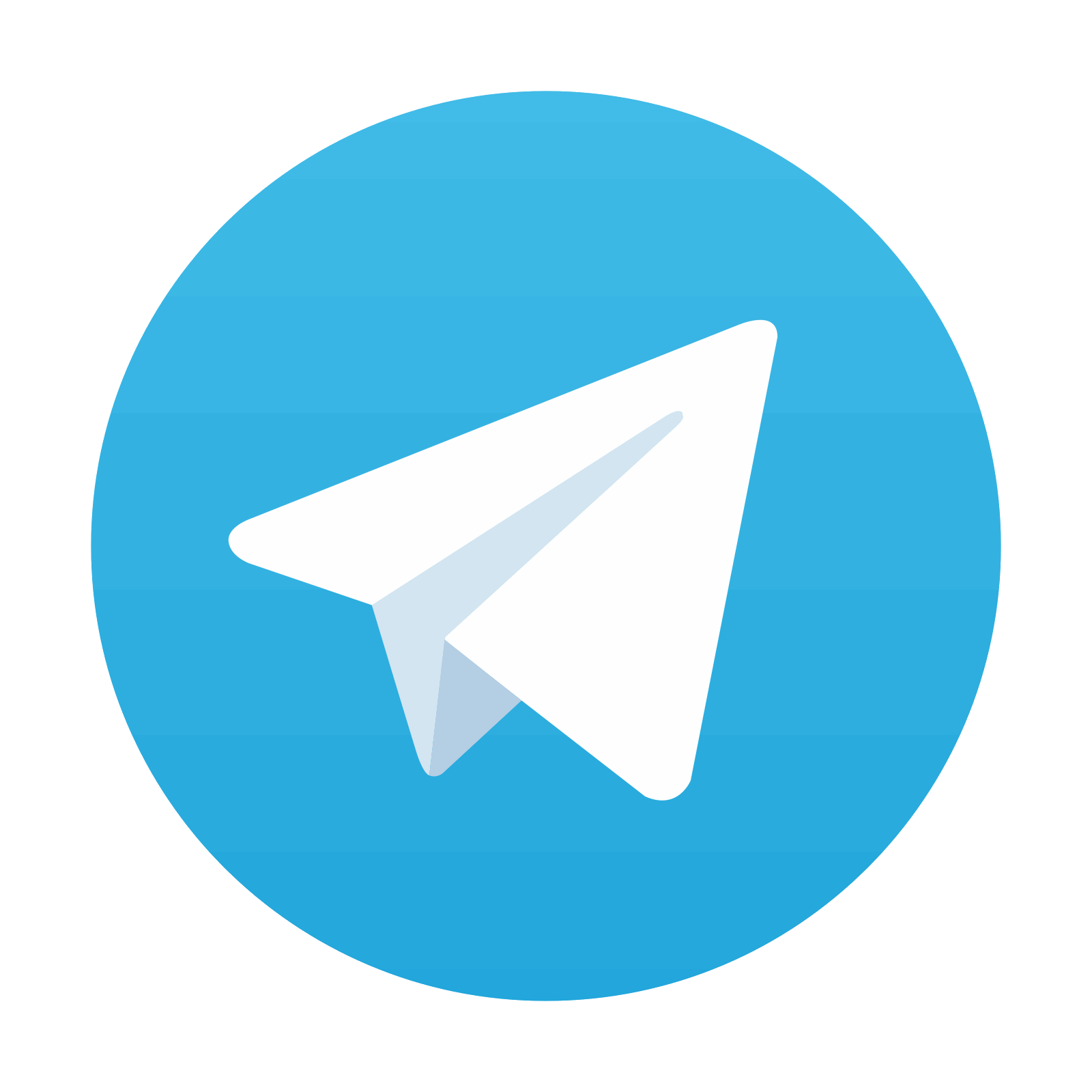
Stay updated, free articles. Join our Telegram channel

Full access? Get Clinical Tree
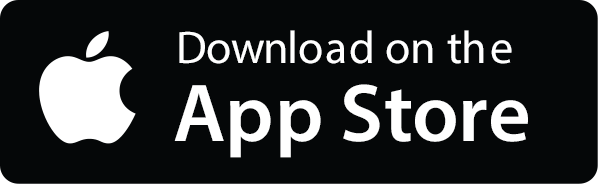
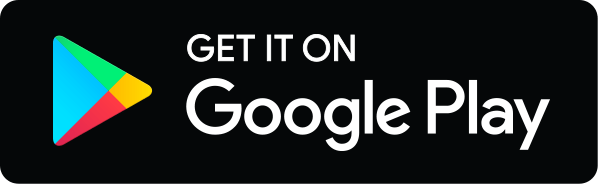