Fig. 11.1
a Immunocompetent cells within the skin: Langerhans cells (LC) form a network within the epidermis. Dermal dendritic cells (DC) reside below the basal membrane. T lymphocytes migrate through the skin. b The leukocyte adhesion cascade: Loose bindings via selectin-PSGL-1 interactions lead to rolling of the leukocyte. Further adhesion results in activation and firm attachment to the endothelial wall, and, lastly, extravasation. PSGL-1 P-selectin glycoprotein ligand-1, LFA-1 lymphocyte function-associated antigen 1, MAC-1 macrophage-1, TCR T cell receptor, CLA cutaneous lymphocyte-associated antigen, ICAM intercellular adhesion molecule, VCAM vascular cell adhesion molecule, JAM junction adhesion molecule
The leukocyte adhesion cascade is not specific for leukocyte recruitment to the skin. The process of leukocytes homing to solid organs follows the same steps, with minor exceptions: No ICAM-1 is necessary for adhesion to neutrophils on hepatic sinusoids [33], and in the heart, neutrophils leave the circulation from larger coronary veins instead of postcapillary venules [34].
One molecule that distinguishes skin-homing lymphocytes from others is the surface receptor cutaneous lymphocyte-associated antigen (CLA). It contains the glycoprotein Sialyl-LewisX, and readily binds to selectins [35]. CLA+ T cells are found in the skin of inflammatory dermatoses in high numbers [35]. Apart from their contribution to skin autoimmunity and defense, Clark et al. have found a high number of CLA+ T cells in normal steady-state human skin, which even exceeded the number of circulating cells [7]. It is proposed that skin-homing T cells, apart from their detrimental effects in inflammatory skin diseases, are an important factor of immune surveillance in the steady state. Most of these isolated CLA+ T cells from noninflamed skin showed high levels of CCR4 and CCR6, and 50 % stained positive for CCR8 and CXCR6. The isolated cells contained a very diverse T cell receptor repertoire, suggesting a broad array of pathogen defense. Another recent finding was that almost all peripheral blood CD4+CD25hiFoxp3 regulatory T cells (Tregs) expressed high levels of the skin-homing receptor CCR4; 80 % were found to express CLA and 73 % CCR6 [8].
The high number of skin-resident T cells under normal conditions also indicates that these skin-homing cells are already sufficiently arrested by baseline endothelial adhesion molecule expression and do not depend on inflammation-induced upregulation. When skin from psoriatic patients was transplanted into immunodeficient mice, the number of skin-resident T cells sufficed to cause psoriatic lesions in the host [36].
Taken together, these findings indicate that migration of T cells into the skin is a physiologic and frequent process necessary for defense as well as tolerance towards pathogens and injury. Skin-homing T cells express a homing marker signature that distinguishes them from T cells that are affiliated with other organs [37].
Leukocyte Adhesion Molecules in Skin Rejection
Evaluation of the expression of adhesion molecules in skin biopsies from five hand/upper extremity transplant patients indicated that a specific pattern of adhesion molecules was upregulated upon rejection. The markers LFA-1, ICAM-1, and E-selectin were highly upregulated during rejection, and expression of those markers also correlated well with the severity of rejection in immunohistochemical stains. In samples of nonrejecting skin, none of these markers was upregulated [16].
Blockade of adhesion molecules seems an attractive approach to treat skin inflammatory conditions of all kind. While some approaches have proven ineffective due to the redundancy of these pathways [38], others have revealed substantial effects. For example, the small molecule inhibitor for E- and P-selectin, efomycine M, was effective in the treatment of plaque psoriasis [39]. In a rat hind limb transplantation study by our group, efomycine M was able to significantly prolong allograft survival when administered subcutaneously into the transplanted limb [16]. In the same experimental model, antibodies against ICAM-1 and LFA-1 were able to delay or even prevent graft rejection (unpublished results).
Other blockers of adhesion molecules, which have been applied clinically for the treatment of various inflammatory diseases, have shown ambivalent success: Natalizumab, a monoclonal antibody against the alpha-4 integrin, is approved for the treatment of severe multiple sclerosis. While causing substantial symptom relief in many patients, the side effect of progressive multifocal leukoencephalopathy was reported [40]. The same was true for efalizumab (anti-LFA-1), a substance for severe psoriasis treatment [41], which led to its withdrawal from the market.
In summary, a pattern of adhesion molecules has been shown to be upregulated during skin rejection, representing novel and interesting targets for treatment and prevention of skin rejection in RT.
In Vivo Assessment of Immune Cell Trafficking
Recently, in vivo imaging techniques have enhanced the ability to investigate immunological mechanisms in transplantation. Because of its accessible location, the skin has become a commonly studied subject in two-photon and single-photon microscopy [42, 43].
Horner et al. have imaged vascularized versus conventional, nonvascularized skin grafts with intravital confocal microscopy [44]. They report a fundamental difference in the kinetics and distribution of infiltrating host cells comparing vascularized versus nonvascularized skin: In nonvascularized skin grafts, the infiltrating cells are localized at the edges of the graft [45]. In primarily vascularized flaps, the cells tended to distribute more densely at the center, and were twice as many as in nonvascularized grafts. In both transplant types, the cellular infiltrate was localized in the upper dermis, clustered around hair follicles in both graft types, and additionally was cuffed around blood vessels in the vascularized skin grafts. A positive major histocompatibility complex II (MHC-II) staining could be detected in dermal endothelia of primarily vascularized grafts, but was absent in nonvascularized skin grafts. Along with their observation of the infiltrate localized primarily in the upper dermis and around hair follicles, the authors argue that not the epidermis, but different antigens might be the main target of the alloimmune response [44]. The observation period of the trial was only 4 days posttransplant and the infiltrate might have been in an early stage of rejection; any comparison with the Banff classification system [20] is therefore not meaningful.
Another in vivo imaging study using two-photon microscopy has characterized the contributions of both donor and host cells to acute rejection in a mouse model of nonvascularized ear skin transplantation [45]. Soon after transplantation, a burst of host CD11c positive cells infiltrated the graft; among those were mainly neutrophils (at earlier time points) and monocytes (the majority after day 9). This early influx of cells was the same in allogeneic and syngeneic transplants, indicating it to be antigen unspecific. Dermal dendritic cells (dDCs) from the graft were shown to rapidly migrate from the graft to the draining lymph nodes. In the allogeneic setting, however, the morphology of the dendritic cells resembled those of dead cells when they had reached the lymph nodes. LCs did not seem to contribute at all to the immunologic processes, but rested immobile in the epidermis until rejection was complete. Additionally, the study revealed that graft-infiltrating recipient cells were able to reach the draining lymph node and cross-prime CD8+T cells.
These findings strongly point to the importance of the indirect pathway even at very early phases of the alloimmune response. Although the study was performed in nonvascularized skin grafts and it might be delicate to draw the same conclusions also for vascularized skin, there are other studies that advocate the importance of the indirect presentation pathway in the allogeneic response. Natural killer cells were shown to rapidly kill graft dendritic cells once they had reached the lymph node [46]. In solid organ transplantation and bone marrow transplantation , similar findings have been reported: Immunosuppressive dendritic cells (ISDC) have been cultured as a tool to prolong allograft survival, which was actually demonstrated in some animal models [47–50]. While it was long assumed that these dendritic cells would perform a “tolerogenic” direct antigen presentation to host T cells, this paradigm was recently challenged by a study, which showed that these ISDCs quickly vanished from the circulation and were uptaken by recipient dendritic cells. Depletion of recipient dendritic cells at the time of ISDC application abrogated their previously permissive effect on cardiac graft survival [51]. Such findings need further confirmation in the setting of RT, but might have an impact on the way future immunosuppressive strategies are designed.
Antigen-Presenting Cells in Skin Rejection
APCs form the bridge between innate and adaptive immunity. Knowledge about different subpopulations of APCs within the skin has only recently accumulated . Our overall understanding of APC function and their characteristic maturation and migration cycle was postulated following in vitro experiments. In brief, antigen uptake by the immature APC leads to maturation of the cell, downregulation of adhesion molecules, and detachment. The maturating cell travels via the lymphatic vessels to a draining lymph node, enters, and primes T cells with the help of newly upregulated molecules such as CCR7 [52–54].
In general, two major populations of resident dendritic cells have to be distinguished within the skin: the dDCs and epidermal LCs . Currently, a major debate concerning their immunogenic versus tolerogenic functions is ongoing—while some investigators use dendritic cells to induce tolerance, there are other studies that indicate their potent immunostimulatory functions. A critical confounding factor for any APC study is the environment—whether those cells are investigated in or ex vivo, under inflammatory or tolerogenic conditions, and if they are immature or mature [55]. A substantial part of APC research is done in the context of contact hypersensitivity (CHS). Recently, an array of transgenic animal models for skin APCs has been developed, in most studies leading to inconsistent results [56]. However, a comprehensive analysis of the current data on skin APCs is beyond the scope of this chapter, which will focus only on the aspects concerning RT .
In both hand and face transplantation, there is little but some information on the contribution of skin APCs. LCs were found to have surprising longevity in transplanted skin—in a hand transplant patient, they were isolated 10 years post transplant, where they seemed to form a stable population and renew from epidermal precursor cells [57]. Upon major destructive stimuli, such as UVC irradiation, LCs have been shown to be replenished from bone marrow precursors [58]. From an academic point of view, it would be interesting to evaluate how this replacement happens in a bone marrow containing allograft—from host monocytes, host LC precursors, or actually viable donor LC precursors from the bone marrow niche? .
Skin APCs have been exploited for their abilities to induce tolerance within the skin. In a recent study, LCs showed only a weak capacity to uptake, process, and present bacterial antigen on MHC-II molecules. Compared to dDCs, immature LCs possess a restricted repertoire of toll-like receptor (TLR) for bacterial uptake. They only poorly activated bacteria-specific T cells, as compared to dDCs. On the contrary, these bacteria-primed LCs induced Foxp3+ cells [59]. Studies like this indicate that the epidermis, although representing the “first barrier” for invaders, has a tolerogenic function that allows commensal, noninvasive bacteria and fungi to reside. Parallel hypothesis exist for the gut flora, where a specialized CD103+ dendritic cell type has been reported to induce bacteria-specific Tregs [60].
Conclusive Remarks
Specific ways to overcome skin rejection require more in-depth knowledge about mechanisms of rejection within the skin. Many of our theories to date are extrapolated from dermatology research, in particular from chronic inflammatory dermatoses. Inflammation in such conditions, however, is always a result of an orchestrated communication between skin-resident and skin-invading cells. In the setting of allotransplantation, the skin-resident cells are donor cells, whereas skin-invading cells are recipient cells. It was noted in a recent in vivo imaging study that dDCs from allogeneic skin grafts did not prime host T cells in the lymph node (i.e., via the direct pathway), but were dead by the time they had reached the draining node [45], indicating that the mechanisms triggering the immune response may differ in this setting.
The relevance and contribution of APCs such as LCs in skin transplantation remain unclear at this point. The long-lived, very resistant cells seem to interact with the commensal bacterial flora [59], but their contribution to skin rejection remains unclear.
T regulatory cells have been identified in hand allografts [12, 16], and like for solid organ transplantation, a role for peripheral tolerance has been implemented. Still, neither in solid nor vascularized composite allotransplantation strategies to locally enhance and enrich those cells are available.
Blocking leukocyte extravasation into the skin seems a promising approach. Leukocyte adhesion molecules are upregulated in hand allograft rejection [16], but the side effects of adhesion molecule blockers in psoriasis or multiple sclerosis limit their application in skin rejection.
Even though the immunology of transplanted skin is starting to be better explored, we do not have means to specifically target the alloimmune response towards the skin. However, the skin offers the advantage that treatment can be applied locally. We believe that local concepts for diagnosis and therapy of skin rejection have to be further exploited.
References
1.
Nestle F, Di Meglio P, Qin J, et al. Skin immune sentinels in health and disease. Nat Rev Immunol. 2009;9:679–91.PubMedCentral
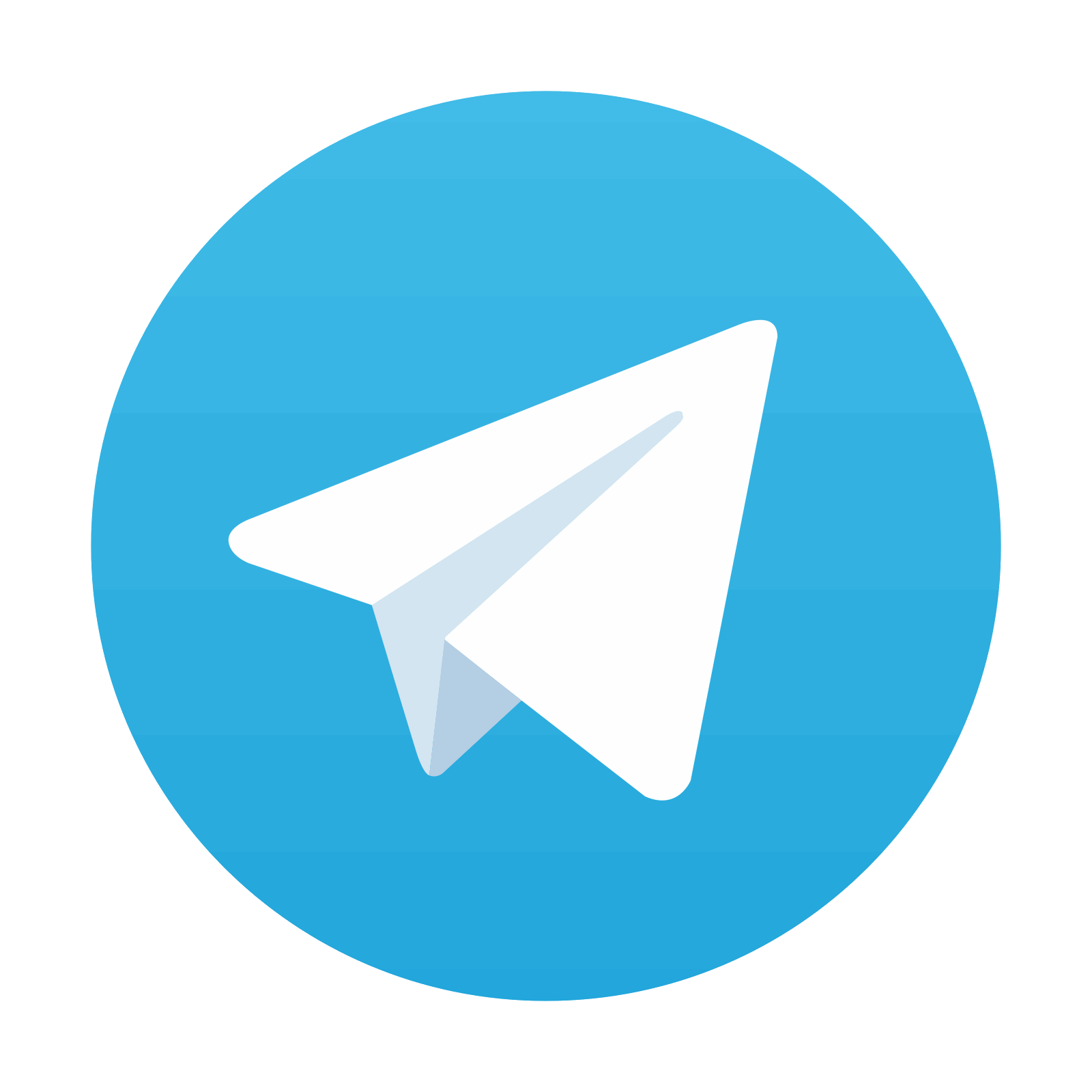
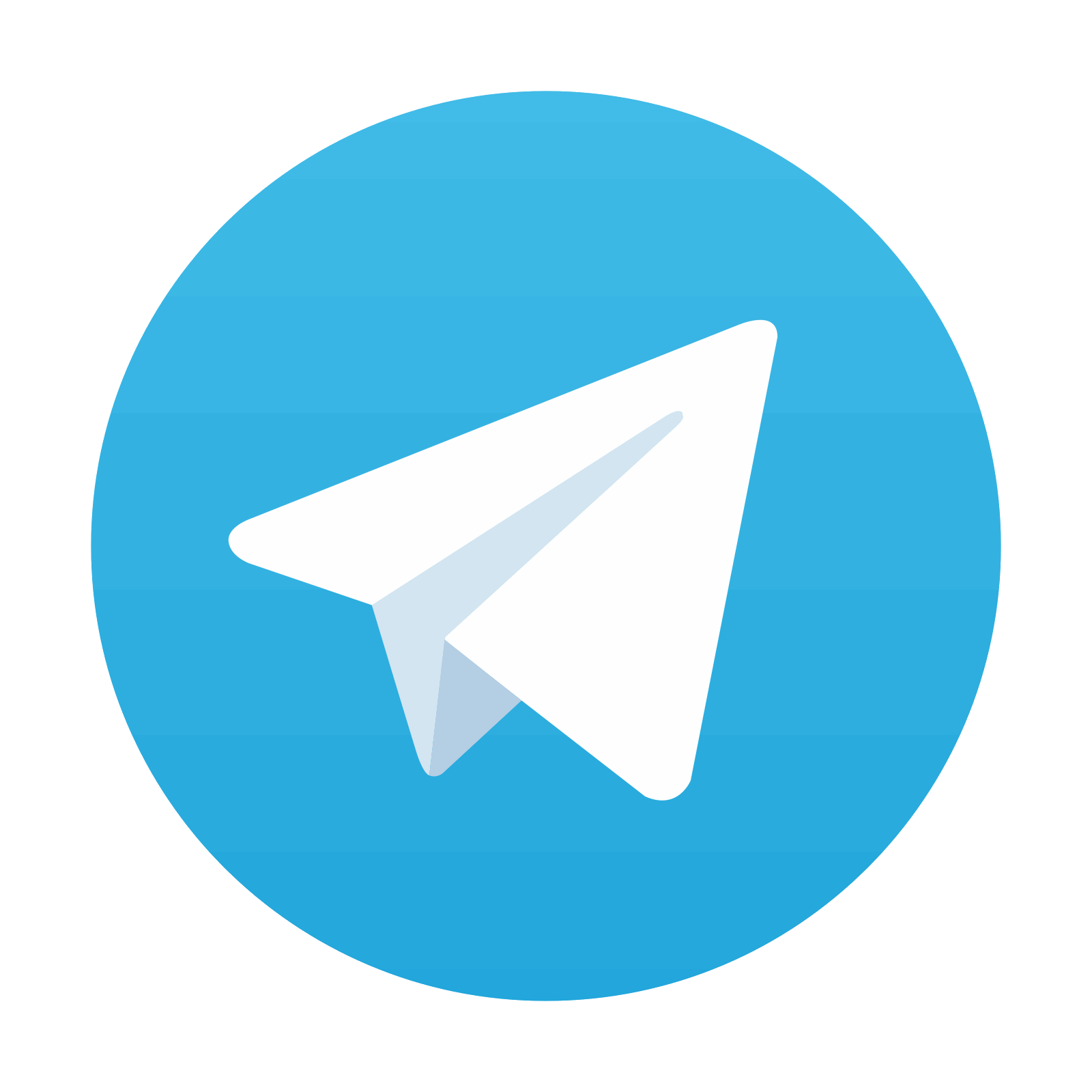
Stay updated, free articles. Join our Telegram channel

Full access? Get Clinical Tree
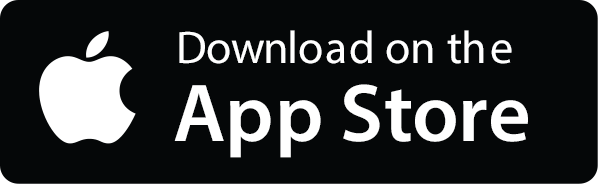
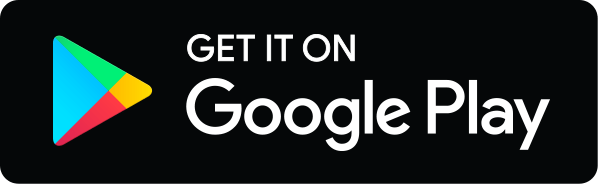
