Fig. 4.1
Cutaneous dendritic cells in the steady state and in the inflammatory state. LCs Langerhans cells, dermal DCs dermal dendritic cells, IDECs inflammatory dendritic epidermal cells, Tip-DCs tumor necrosis factor, and inducible nitric oxide synthase-producing dendritic cells, pDCs plasmacytoid dendritic cells
Recently, several studies have revealed the complexity and functional specialization of the cutaneous DC network, showing that as in the lymphoid organs, multiple subsets of DCs coexist in the dermis, and that skin DC subsets exhibit specific immune functions.
This review defines the functions of each cutaneous DC compartment and discusses the potential implications of these functions in the development of skin immune responses.
4.2 Dendritic Cells (DCs) of the Skin
4.2.1 Langerhans Cells
4.2.1.1 Localization
The skin can be broadly classified into two distinct regions, the epidermis and the dermis. The epidermis is derived from ectoderm and presents as an epithelial layer composed mainly of keratinocytes. Keratinocytes provide skin integrity and produce the stratum corneum, which is the water-impermeable outermost layer of the skin.
LCs are the only DCs that are found in the epidermis during the steady state. LCs are generally found in the basal and suprabasal layers of the epidermis, where they form a dense network of cells that account for approximately 2–4 % of the total epidermal cell population in the epidermis of mice and humans [8]. LCs are the only hematopoietic cells in the epidermis of human skin in the steady state, whereas mice have an additional population: epidermal γδ T cells [9].
LC precursors first populate the epidermis on embryonic day 18 and proliferate in situ to form a radio-resistant, self-renewing population [10, 11]. They are characterized by a unique cytoplasmic organelle known as a Birbeck granule . Although the precise function of Birbeck granules remains unclear, they are thought to play a role in receptor-mediated endocytosis and the transport of cellular materials into the extracellular space [12].
4.2.1.2 Surface Marker
Human and murine LCs are easily identified in the epidermis by the expression of CD45 and major histocompatibility complex (MHC) II molecules. They also constitutively express the lection receptor, namely, langerin/CD207 , which is capable of binding sugar moieties commonly found on a variety of microorganisms [13]. Human and murine LCs express the adhesion molecules E-cadherin and epithelial-cell adhesion molecule (EpCAM) , which anchor LCs to keratinocytes [14, 15], and CD205 that is implicated in antigen capture and antigen processing [16, 17]. Human LCs highly express CD1a, a member of the group 1 CD1 protein, which is capable of presenting microbial lipid antigens to T cells [18] (Table 4.1). Although the majority of LCs are thought to be derived from bone marrow precursors, recent studies have identified a novel pathway by which CD14+ cells resident in the dermis acquire LC features following treatment with TGF-β [19, 20]. In humans, CD34+ hematopoietic progenitor cells [21, 22], monocytes [23], and dermal CD14+ DCs [19] were shown to give rise to LC-like cells in vitro. TGF-β is a critical factor for LC development both in vitro and in vivo, as LCs are absent in the epidermis of TGF-β-deficient mice [14]. LC differentiation may thus be somewhat dependent on the cytokine microenvironment of the skin at any given point in time.
Table 4.1
Dendritic cell population in the skin of humans and mice
Localization | Cell type | Cellular markers | |
---|---|---|---|
Human | Epidermis | LC | CD45, MHC classII, CD1a, CD207 (Langerin), E-cadherin, EpCAM |
IDEC | CD1a, CD1b, CD1c, CD11c, FcεRI, CD23, HLA-DR, CD11b, CD206, CD36 | ||
Dermis | CD1c+ dDC | CD1c, CD1a+/−, CD45, CD11b, CD11c, MHC classII | |
CD14+ dDC | CD1c, CD45, CD11b, CD11c, CD14, MHC classII, CD209 (DC-SIGN) | ||
CD141+ dDC | CD1c, CD45, CD11c, CD141 | ||
pDC | CD303 (CLEC4C), CD304 (neuropillin), CD123 (IL-3R) | ||
Tip-DC | CD11c, TNF-α, iNOS | ||
Mouse | Epidermis | LC | CD45, CD11b, CD11c, CD24, MHC classII, CD205, CD207, E-cadherin, EpCAM |
Dermis | Langerin− dDC | CD45, CD11bhi, CD11c, CD24, MHC classII, CD205, SIRP-1α | |
Langerin+ dDC | CD45, CD11bdim, CD11c, CD24, CD103, MHC classII, CD207 | ||
pDC | B220, SiglecH, PDCA-1 |
Upon encountering pathogens, LCs can capture antigens and undergo a maturation that involves upregulation of MHC class I and class II molecules—costimulatory molecules including CD40, CD80, and CD86—and chemokine receptors such as CCR7, as well as downregulation of E-cadherin that allows them to migrate out of the skin to draining LNs, where they present antigens to T cells [24]. However, despite intensive studies to reveal the functional role of LCs over the past several decades, the in vivo function of LCs remains not fully understood, as discussed later.
4.2.1.3 Homeostasis of LCs
Homeostasis of LCs in the inflamed skin depends on the type and the strength of inflammation. In severe inflammatory conditions, such as ultraviolet (UV) light exposure or cutaneous graft-versus-host disease, LCs are replaced by circulating blood precursors [11, 25]. In contrast, in less severe inflammatory conditions that lead to moderate LC loss, where the epidermal–dermal barrier integrity is preserved and is not accompanied by the release of inflammatory chemokines that recruit blood monocytes, the remaining LCs have the potential to repopulate themselves locally and achieve complete recovery in 1 to 4 weeks after tissue injury. These findings suggest that inflammatory signals may control the dynamics of LC proliferation.
4.2.2 Dermal Dendritic Cell
The dermis is a relatively cell-sparse tissue that is derived from mesoderm, which forms a stromal layer immediately below the epidermis. It is populated by fibroblasts that secrete components of the complex extracellular matrix. In the steady state, a variety of immune cells can be found in the dermis including memory T cells, mast cells, and DCs [26, 27].
4.2.2.1 Components and Surface Markers of Dermal DCs
Dermal DCs (dDCs) consist of dermal-resident DCs and migratory LCs on their way to the LNs [28]. Until recently, human and murine dermal-resident DCs were thought to form a homogeneous population that is easily distinguishable from migratory LCs based on the absence of langerin/CD207 expression [13]. However, recent studies have shown that murine langerin-positive dendritic cells in dermis are composed of both LCs and a novel population of dDCs (langerin+ dDCs ) [29–31]. The langerin+ dDC population represents 10–20 % of the total dDCs pool. In contrast to LCs, langerin+ dDCs express the integrin αEβ7 (also called CD103) [32], lack the adhesion molecules E-cadherin and EpCAM, and express low levels of the integrin CD11b. Langerin+ dDCs arise from bone marrow precursors in an FMS-like tyrosine kinase 3 (FLT3)-dependent manner [29, 30, 33]. Langerin+ dDCs express the same high level of CD24 as LCs, but do not express CX3CR1, F4/80, or signal-regulatory protein alpha (SIRPα), and express low levels of CD11b and EpCAM [33] (Table 4.1).
The classical langerin− dDCs represent the majority (up to 70 %) of the dDC pool and express high levels of the integrin CD11b and several macrophage markers such as F4/80, CX3CR1, and SIRPα [33]. Interestingly, their expression of CD24 is heterogeneous, suggesting that dermal langerin− dDCs might not represent a homogeneous subset of dDCs, either in origin or in maturation status [34].
DCs were thought to be terminally differentiated cells, with no proliferative capacity. However, recent studies have revealed that in lymphoid organs DCs actively proliferate in the steady state [35, 36]. All dermal DC subsets proliferate, although CD103+ dDCs do so at a higher rate than the CD11b+ subset [33, 34].
The chemokine receptor CCR7 regulates the constitutive migration of all cutaneous DCs to the skin-draining LNs [37, 38]. In the inflammatory state, tissue-resident DC migration to the draining LNs increases markedly [39]. Interestingly, cutaneous DC subsets migrate differently from the skin-draining LNs in response to the sensitization with hapten. Migration of CD11b+ and CD103+ dDCs reaches a peak 1 day later, followed by LCs at 4 days postsensitization [40, 41]. It is also important to note that cutaneous DCs undergo phenotypic changes upon migration from the skin to the LNs [34].
4.2.3 Plasmacytoid Dendritic Cells
Plasmacytoid DCs account for 0.2–0.8 % of all peripheral blood mononuclear cells [42]. Under steady-state conditions, pDCs are absent from the skin and other peripheral nonlymphoid tissues, but they have been shown to infiltrate into the dermis in viral infection [43] and chronic inflammation as seen in psoriasis [44–46], lupus erythematosus [47], and AD [44, 48, 49]. Plasmacytoid DCs lack myeloid antigens CD11b, CD11c, CD13, and CD33, but express CD45RA, variable CD2 and CD7, and may harbor T-cell receptor and immunoglobulin rearrangements. They are now separable from mDCs by positive markers CD123 (IL-3R), CD303 (CLEC4C; BDCA-2), and CD304 (neuropilin; BDCA-4) [50] (Table 4.1).
The propensity of pDCs to release type I interferon in response to viruses is one of the first specialized pDC functions to be described [51–53]. They express very high levels of Toll-like receptors (TLR) 7 and TLR9 , which transduce signals from viral and self-nucleic acids [42, 54]. After stimulation by viral or other microbial products, pDCs produce large quantities of type I interferons, triggering pDC maturation and migration to the LNs. PDCs become activated by a regulated expression of antimicrobial peptides (AMPs) in damaged skin. However, overexpression of AMPs leads to the initiation of an autoimmune reaction, through an excessive sensing of self-nucleic acids and uncontrolled secretion of type I IFNs by pDCs, as seen in psoriasis.
4.2.4 Inflammatory Dendritic Cells (Tip-DCs and IDECs)
4.2.4.1 Tip-DCs
A newly identified DC subpopulation referred to as TNF-α and inducible NO synthase (iNOS)-producing DC (Tip-DC) has been reported to be critical in bacterial infections, as demonstrated in a model with Listeria monocytogenes [55]. Tip-DCs express CD11c, TNF-α, and iNOS (Table 4.1). Evidence that TNF-α inhibitors were able to reverse disease activity in psoriasis suggests that Tip-DC may represent major inflammatory effector cells in psoriasis [56]. Pathogenicity of Tip-DCs in psoriasis is suggested by the rapid downmodulation of Tip-DC products TNF-α, iNOS, IL-20, and IL-23 during treatment with effective therapies [57, 58]. They have the capacity to skew T cells into Th1 and Th17 T cells, which are a new set of T cells associated with autoimmune inflammation in many disease models [59].
4.2.4.2 IDECs (Inflammatory Dendritic Epidermal Cells)
Inflammatory dendritic epidermal cells (IDECs) were found in the epidermis of atopic dermatitis (AD) skin lesions. Compared to LCs, they lack Birbeck granules and langerin expression, but show expression of the mannose receptor (MMR)/CD206, providing IDECs with the ability to take up mannosylated protein antigens [60]. They also express CD1a, CD1b, CD1c, CD11c, CD23, HLA-DR, CD11b, CD206, and the low-affinity receptor for IgE (FcεRII/CD23; Table 4.1). In lesional skin of AD, both IDECs and LCs show expression of the high-affinity receptor for IgE (FcεRI) and CD36; however, expression of both receptors is stronger on IDECs. Peripheral blood monocytes as well as dermal DCs are regarded as potential precursor cells of IDECs [61].
4.3 Roles of Cutaneous DCs in Skin Immune Responses
4.3.1 The Role of Each Cutaneous DCs Subset in the Murine CHS Model
CHS is a murine model for allergic contact dermatitis, and has become a widely used technique to analyze adaptive skin immune responses. Mice are sensitized by application of hapten such as 2-dinitrofluorobenzene (DNFB) onto shaved abdominal skin. Five to six days later, the same hapten is applied to the ear. The extent of ear swelling that develops after 24 h correlates with the magnitude of the effector response [62].
Until recently, LCs have been considered to play central roles for antigen presentation in the sensitization phase of CHS. Because they have strong antigen-presenting ability in vitro, they reside abundantly in the basal layer of the epidermis, and their artificial removal by drugs, such as gliotoxin application to the skin, results in impaired CHS response [63]. However, novel depletion systems of LCs (langerin–diphtheria toxin receptor knock-in mice ) have revealed that langerin+ dDCs, but not LCs, may have a crucial role during the sensitization phase [29, 40, 64] (Table 4.2). Other groups have recently reported that these two populations work in a compensatory manner during the initiation of the sensitization phase [65, 66]. In line with this result, Batf3-deficient mice which lack langerin+ dDCs exhibited a normal CHS response [67]. These findings indicate that the function of langerin+ dDCs in the sensitization phase of CHS is compensated by other DC subsets (Table 4.2). On the other hand, the depletion of skin DCs in hapten-sensitized mice enhanced the effector phase of CHS [68]. This suggests that there exist other DC subsets that regulate the elicitation phase of CHS.
Table 4.2
Dendritic cell ablation systems
Mouse lines | LCs | Langerin+ dDCs | Langerin− dDCs | CD8+ DCs | |
---|---|---|---|---|---|
Constitutive systems | Langerin-DTA | Absent | Normal | Normal | Normal |
Batf3−/− | Normal | Absent | Normal | Absent | |
Inducible systems | Langerin-DTR | Absent | Absent | Normal | 25 % |
(1 day after DT injection) | |||||
Langerin-DTR | Absent | 20–50 % | Normal | Normal | |
(7–13 days after DT injection) |
It has also been reported that LCs have a regulatory role, rather than a stimulatory role, during the sensitization phase of CHS. Research on another LC depletion system that uses the human langerin promoter combined with diphtheria toxin A (the active subunit of diphtheria toxin [69]) revealed that congenitally LC-depleted mice exhibited an exacerbated CHS response, suggesting that LCs may have a suppressive function in CHS [70] (Table 4.2). When the CHS response was induced with a hapten through full-thickness grafted skin, the development of CHS was suppressed. A high expression of receptor activators of nuclear factor κ-B ligand (RANKL) was observed in the grafted skin, and recombinant RANKL stimulated LCs to produce IL-10 , suggesting that the LCs play important roles for regulating the peripheral inflammation in CHS [71].
Thus, although the function of LCs in the sensitization phase of CHS remains controversial, langerin+ dDCs probably affect stimulatory effects during sensitization. The function of langerin− dDCs in sensitization has not yet been fully investigated; however, langerin− dDCs also appear to have stimulatory functions in the sensitization phase, because ablation of both LCs and langerin+ dDCs before sensitization impairs CHS response but is unable to abrogate it completely [29, 64, 66].
4.3.2 The Role of Cutaneous DCs in Atopic Dermatitis (AD)
AD is a pruritic chronic retractable inflammatory skin disease that is induced by the complex interaction between susceptibility genes encoding skin barrier components and stimulation by protein antigens [72, 73]. Upon protein antigen exposure, DCs acquire antigens and stimulate the proliferation of T cells to induce distinct T helper cell responses to external pathogens [2]. Therefore, it has been suggested that DCs initiate AD in humans [74]; however, it remains unclear which cutaneous DC subset initiates epicutaneous sensitization to protein antigens. The application of large molecules is localized above the size-selective barrier, tight junction (TJ) , and activated LCs extend their dendrites through the TJ to take up antigens [75]. Therefore, it can be hypothesized that it is not dermal DCs but rather LCs that initiate epicutaneous sensitization with protein antigens, as in the development of AD.
In keeping with this, skin DCs elicit a Th2 response in the presence of mechanical injury by inducing cutaneous thymic stromal lymphopoietin (TSLP) [76]. TSLP is a cytokine that is produced mainly by nonhematopoietic cells such as fibroblasts and epithelial cells, including epidermal keratinocytes. TSLP plays an important role in the induction of Th2 responses through the activation of DCs via the expression of OX40L [77]. Previous reports have shown that TSLP is highly expressed in the lesional skin of AD patients and that it activates human epidermal LCs and DCs in vitro [78–80].
In the murine AD model, which is induced by epicutaneous sensitization with protein antigen, LCs are the essential cutaneous DC subset in the induction of IgE upon epicutaneous sensitization with protein antigens. Moreover, TSLP receptor (TSLPR) expression on LCs is enhanced upon protein antigen exposure to the skin and TSLPR signaling on LCs plays an important role in this process. TSLP stimulation causes LCs to express OX40L and BMDCs to induce Th2 chemokines while suppressing Th1 chemokines, which may shift the immune environment to a Th2 milieu [81]. These findings suggest that TSLPRs on LCs can be a therapeutic target of skin inflammatory reactions induced by epicutaneous sensitization with protein antigens, such as in the development of AD (Fig. 4.2).
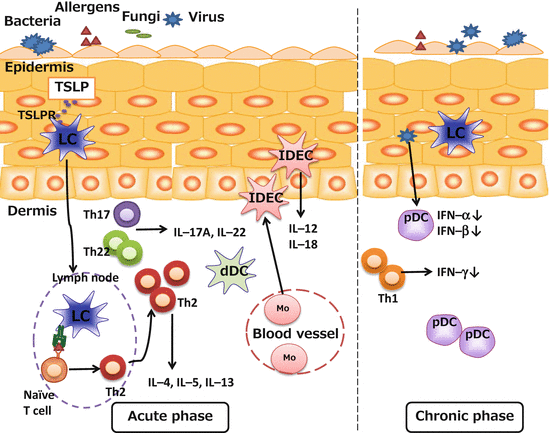
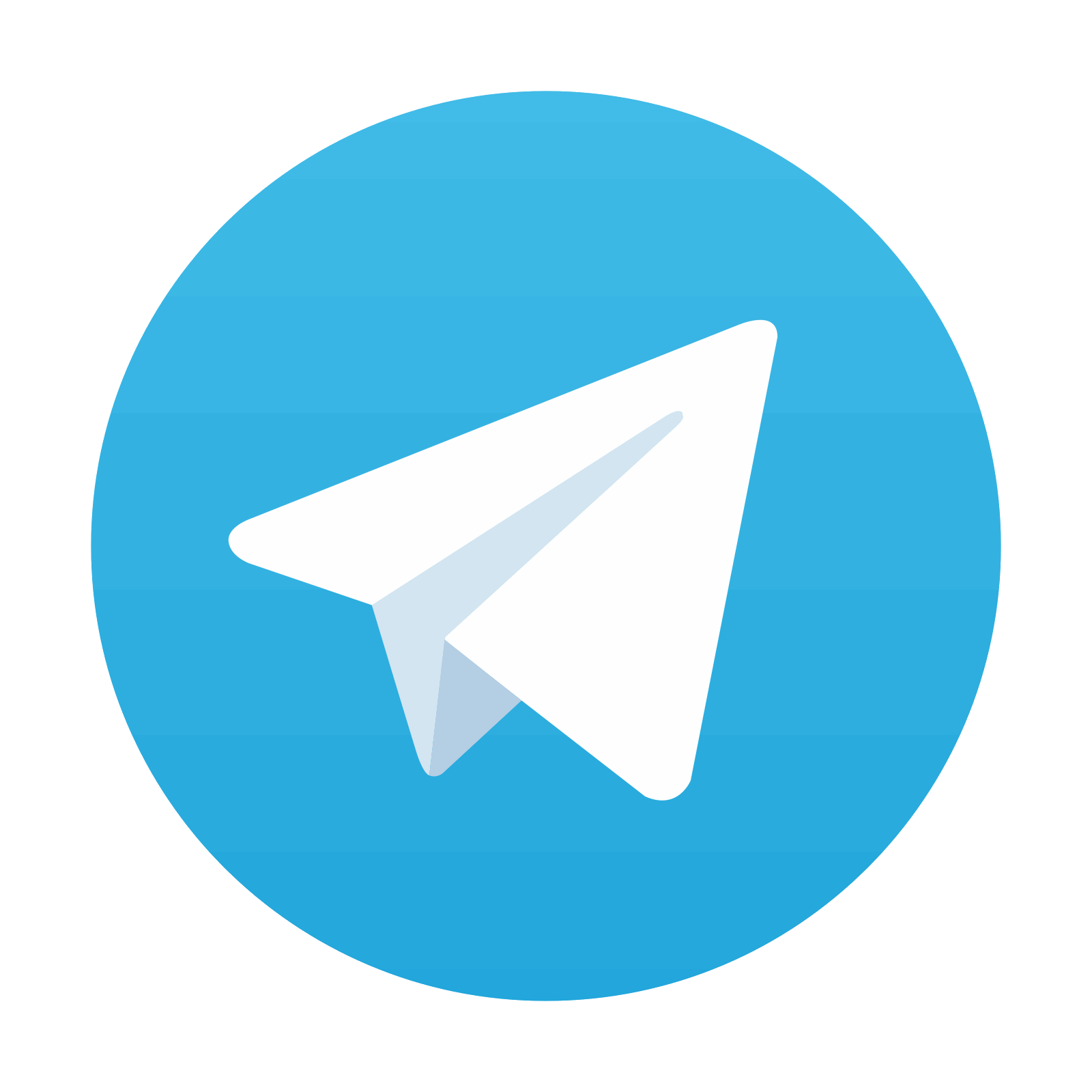
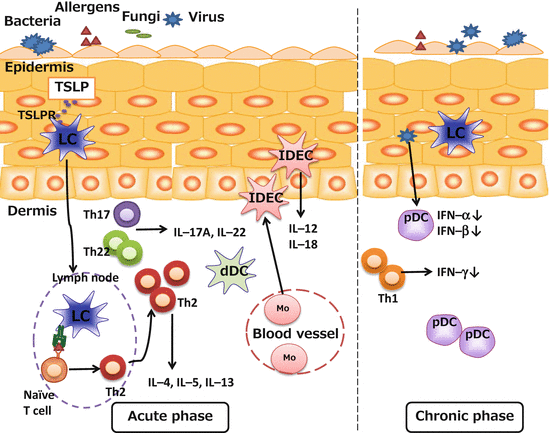
Fig. 4.2
Role of cutaneous DC subsets in atopic dermatitis. LC Langerhans cell, IDEC inflammatory dendritic epidermal cell, pDC plasmacytoid dendritic cell, dDC dermal dendritic cell, Mo monocyte, IL interleukin, IFN interferon
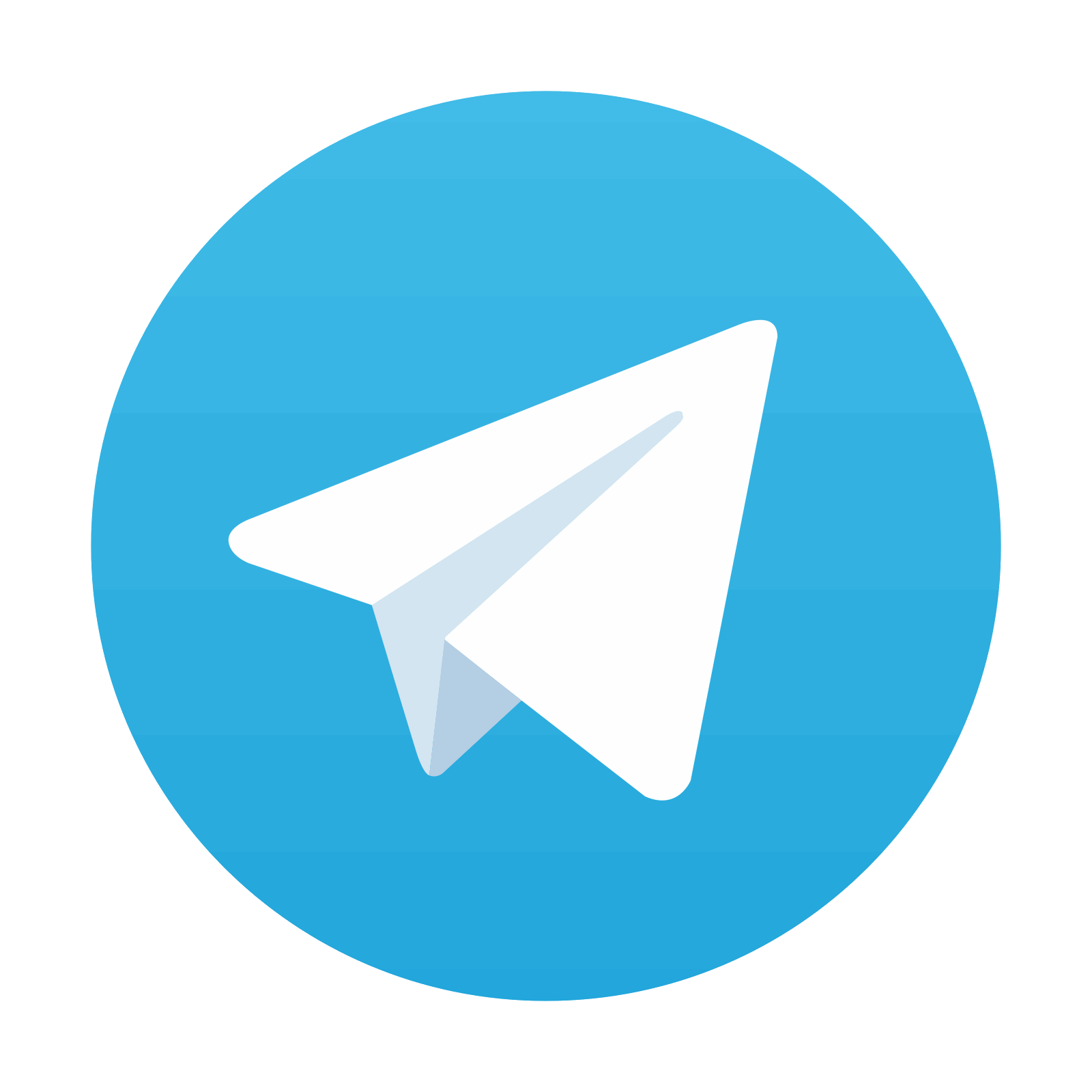
Stay updated, free articles. Join our Telegram channel

Full access? Get Clinical Tree
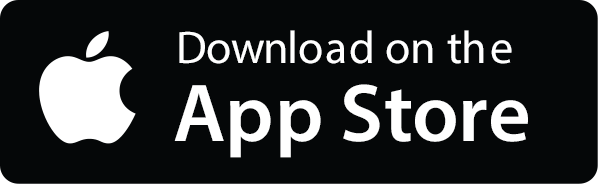
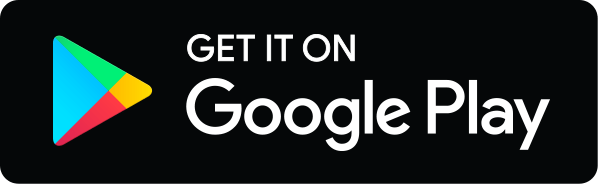