40 It has been said that “necessity is the mother of invention” and in this new millennium of spine care, there is a need for finding a way to provide a better cure for back pain without predisposing patients to future spinal problems. Invariably, much of today’s painful spinal conditions are centered on the functional spinal unit and the degenerative processes that occur to the intervertebral disk. Subsequently, before one could “invent” a theoretical instrumentation construct designed both to address spinal pain and to slow the process of disk degeneration, one must first understand the time-dependent cascade of events that leads to the physiological deterioration of the intervertebral disk. There exists a certain group of people that will experience disk degeneration. As with any other degenerating joint, a specific subset of this group will become symptomatic from this degenerative process. Kirkaldy-Willis et al1 was a pioneer in categorizing the three stages of this disk degeneration process. In stage one, there appears to be some unknown initiating event that causes end plate calcification of the vertebral body, limiting nutrient diffusion and subsequently causing adverse changes in the biochemistry and physiology of the nucleus pulposus (NP) within the intervertebral disk.2 The nucleus is a thick and highly viscous gel-like substance consisting primarily of water-laden proteoglycans whose main function is to absorb shock and transmit loads. Age-related changes to the disk cause it to become a more fibrous material with abnormal viscoelastic properties. These property changes in turn lead to asymmetrical load distributions through the end plates and annulus, which in turn can cause pain as described by Henry Crock in 1970 as “internal disk disruption.”3 This may be the first source of pain experienced due to the abnormal loading pattern seen within the disk. It is theorized that as axial loads are applied, due to the altered material properties of the disk, asymmetrically distributed localized “high spot loading” occurs.4,5 This “high spot loading” causes localized regions of excessive stress amplitudes and consequently excessive localized deformations, which in turn lead to pain. On a longer-term basis, load shifting (caused by the degenerated and abnormal NP) asymmetrically shifts forces to both the vertebral end plates and the annulus fibrosus of the disk. This leads to end plate alterations and annular fissuring.5 At this stage the radiographic appearance of the disk may essentially be normal, but magnetic resonance imaging (MRI) of the disk may show annular tears and be dark on T2 sequences, hence the name dark disk disease. At this point the evolving failure of the disk transitions to the annulus fibrosus and its decreased ability to endure torsional, shear, and axial compressive loading. This subsequently heralds stage two of the degenerative cascade noted by Kirkaldy-Willis; namely, increasing instability of the functional spinal unit. Instability here means “a loss of spinal motion segment stiffness such that the application of force to a particular motion segment produces greater overall displacement (s) than would occur in a normal structure.”6 This forms the basis for another source of pain, as well as the potential for progressive deformity, and also puts selected neurological structures at risk. Furthermore, the inability of the nucleus to tolerate normal loads and subsequent abnormal motion leads to a compensatory response. The previously described sequence of events (stages one and two) inevitably leads to Kirkaldy-Willis’s third and final stage of the degenerative cascade. This stage begins with the musculoskeletal system’s ultimate attempt at stabilization of the abnormally loaded, unstable, and nonprotective (of neural structures) functional spine unit. Evidence for this is clearly seen with ligamentum flavum hypertrophy, facet arthropathy, reduced disk height, and osteophyte formation. These biological responses collectively act to stabilize, or in some cases, pseudofuse the functional spine unit to limit motion and thereby reduce pain. Recent literature4 suggests that there may be a “safe window” in which a functional spine unit may be spared the degenerative cascade if the mechanical loads placed on it remain within a physiological range.4 When load amplitude and motion are limited to an as yet incompletely understood physiologically benign “window” of load amplitudes, there is evidence that some regeneration of the disk may occur. Overloading may lead to additional tissue trauma or adaptive changes that initiate or exacerbate disk degeneration. Applied loads or motion outside this “window” may create damage exceeding the reparative abilities of the disk, and thus damage accumulation will occur faster than repair can occur. This rate of discrepancy (repair versus damage) will inevitably culminate in failure of the disk. This is the physiological basis of Stokes’s working hypothesis describing the mechanobiological homeostasis of disk tissue.4 In addition to the various aspects of the degenerative cascade describing how the functional spine unit fails over time, the therapeutic options available to the spine surgeon to treat the aforementioned conditions also impose their own load and motion alterations on the functional spine environment. Thus, in a sense, surgical treatment can “add insult to injury,” by destabilizing the functional spinal unit. It is particularly evident in the postlaminectomy patient who can experience as much as a 40% reduction in the stiffness of the functional spine unit and possibly back pain.7 Of more recent concern is the issue of postfusion adjacent level disk degeneration. Many articles have documented that the disk and end plate adjacent to a fusion, especially at the cephalad level, experience one or more of the following: increased stress, increased mobility or segmental displacement, and increased intradiskal pressure.8–10 Because of the nonphysiological and apparently unfavorable environment induced at the cephalad level adjacent to a fusion, the literature reports increased rates of symptomatic degeneration that require surgery. As noted, there is good clinical evidence of disk degeneration in segments adjacent to the fusion. Complete removal of all stresses, loads, and motion at a disk segment suggests there are altered spinal column mechanics with increased stresses occurring at and concentrated in the adjacent levels. These increased loads, motion, and subsequent stresses and pressures in the adjacent disk are affected by the length, location, and stiffness of the fusion mass.4 Gillet11 reported that out of 37 subjects who had a one-level fusion, 32% experienced adjacent level degeneration and 11% needed a reoperation. In the same article, of the 26 subjects who had two-level fusions, 31% of them had degenerative changes and 27% required reoperation. Moreover, when they considered 27 subjects who had three- and four-segment fusions, 66% of them experienced adjacent-level degeneration and 33% required reoperation at the adjacent level. They concluded that we “need to find more reconstructive surgical procedures for the management of symptomatic degenerative spines that are resistant to conservative treatment.” With regard to the transitional segment predisposed to degeneration, Gillet also stated that a possible solution to address that entity was to do some kind of “preventative reinforcement” of the adjacent level. Fortunately, a paradigm shift in the approach to treating spinal problems is occurring that will help advance the technology of spinal treatments to become more on par with those used to treat other degenerated musculoskeletal joints. This paradigm shift is based upon the change in design philosophy from fusion and arresting spinal motion, toward instrumentation designs that decompress the stenotic motion segment and allow controlled movement of the functional spine unit while simultaneously unloading and protecting it from excessive forces or motion. The age of dynamic instrumentation of the spine has arrived. Dynamic instrumentation denotes the current physical embodiment of an implant designed with three contemporary realizations in mind. First, fusion changes the natural anatomy, eliminates motion, and may lead to adjacent-level degeneration. Second, due to these changes and the less than ideal successes that fusion confers, the spine community now recognizes that the methods of treating spinal degeneration and instability must change. Third, these changes are manifested in new methods of conceptualizing the surgical processes of stabilization, which in turn have inspired new instrumentation designs aimed at achieving enhanced clinical outcomes while simultaneously minimizing the drawbacks of current technologies. The principal goal of dynamic instrumentation is to achieve stabilization while more closely replicating the natural function of the lumbar spine, as well as providing the patient with symptomatic relief. The instrumentation design allows a predetermined range of motion to achieve symptom relief yet preserve some of the anatomical load-bearing functions of a particular motion segment. Dynamic stabilization has been thought to be safe and effective in the treatment of lumbar degenerative disk disease through early clinical studies in Europe.12 This instrumentation design is an effective means of decreasing the neohinge effect at the adjacent level by increasing the load and motion at the superior level of a fusion. This then led to the belief that dynamic stabilization may also be used to decrease the incidence or severity of adjacent level disk degeneration. Dynamic instrumentation has two important design elements, one intended to change the loading, the other intended to change the motion. Both of these elements work hand in hand to achieve the intended effect. Practically, instability is defined as abnormal patterns of movement within the motion segment that cause altered loading to the spine unit such that pain, deformity, or neurocompromise occurs. It is the combination of both abnormal loading and abnormal motion that leads to a painful motion segment. Therefore, it is for this reason that the ideal design characteristics of dynamic instrumentation must address both abnormal loading and abnormal motion. Prior surgical treatment of lumbar degenerative disk diseases altered both load and motion in nonphysiologically relevant ways. Decompression procedures removed some of the anatomical motion constraints, whereas fusion eliminated all motion and shifted the loads to the adjacent level disk. This subsequently led to an increased likelihood for adjacent level disk degeneration because the stress amplitudes and distributions in the adjacent level disk were altered. Furthermore, the loss of anatomical constraints of the motion segment allowed pathological displacement. The resulting altered loads and motions are the fundamental bases by which pain is generated in the lumbar spine (Fig. 40–1). Figure 40–1 Pain generation in the lumbar spine. Thus the design goals of dynamic instrumentation are to: (1) provide adequate stability to provide symptomatic relief to a painful motion segment, (2) allow some controlled motion to the segment and thereby prevent substantial departure from the normal biomechanics of this segment, and (3) share the loads and stresses applied to that motion segment so that abnormally large loads are not created at the adjacent levels (Fig. 40–2). The ideal dynamic system should unload the disk and provide adequate stability so that symptomatic relief is obtained, yet the system must also simultaneously distribute the load so that the adjacent-level disks are not excessively stressed and thus forced down the degenerative path, which culminates in their subsequent failure. As part of this designed stability with controlled range of motion feature, this ideal system would also preserve the natural anatomy by maintaining lordosis and would incorporate an instantaneous axis of rotation (IAR) that is more anatomically correct than rigid instrumentation techniques provide. All of these features will act in concert to reduce significantly the morbidity and invasiveness of traditional fusion surgery. In addition, the design of the system should be such that the surgeon would find the approach familiar and easy to use. Finally, in the event of an undesirable outcome, the salvage surgery must be simple, with no “burning of bridges.” Figure 40–2 Ideal dynamic system. Figure 40–3 Isobar TTL design. Today, the current embodiment of this system, developed as noted from the work of French engineer Albert Alby and subsequently refined in design and materials, is currently produced from titanium alloy (Ti6Al4Va) by Scient’x USA (Scient’x USA, Maitland, FL). This system, denoted Isobar TTL (Fig. 40–3
Isobar TTL Dynamic Instrumentation
Dynamic Instrumentation and Adjacent Level Disk Degeneration
Biomechanical Performance of the Isobar TTL System
Finite Element Modeling and Analysis
Technical Notes for Placement of the Isobar TTL Dynamic Rods
Dynamic Instrumentation and Adjacent Level Disk Degeneration
Stay updated, free articles. Join our Telegram channel

Full access? Get Clinical Tree
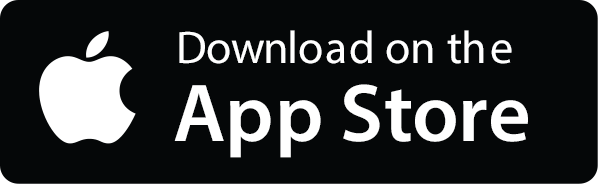
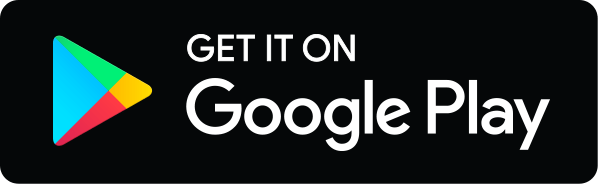