Fig. 2.1
First scans during surgery show reduced perfusion with manual graft compression at the medial portion covering three digits. Note the fourth digit and metatarsal head region which showed clinical signs of necrosis (Reprinted with permission from Perry et al. [1])
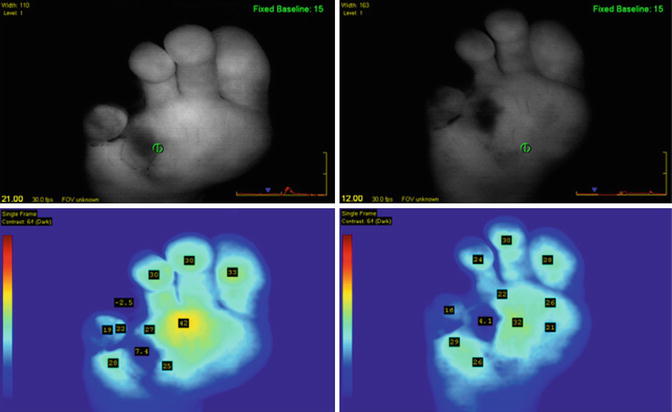
Fig. 2.2
Two follow-up SPY sequences following bypass procedure. Note that the fourth digit continues to demonstrate signs of necrosis and was eventually amputated (Reprinted with permission from Perry et al. [1])
2.3 Ischemia and Ischemia/Reperfusion Injury
Since the macro- and microvascular beds are connected in series, a reduction in macrovascular flow will lead to a decrease in microvascular flow unless compensatory mechanisms are stimulated. The term ischemia is used to denote reduced blood supply due to obstruction of the arterial inflow and was first used in the early nineteenth century. During prolonged ischemic periods, adenosine triphosphate (ATP) levels along with intracellular pH decrease as a result of anaerobic metabolism and lactate accumulation. Tissue injury and cell death are related to the duration and magnitude of the ischemia. The downstream biochemical effects include cellular swelling, increases in intracellular calcium levels, the generation of reactive oxygen species, and mitochondrial dysfunction [2] (Fig. 2.3). There are organ-specific differences that influence the extent, severity, and reversibility of organ damage after an ischemic event. Single-organ ischemia and reperfusion injury can occur in the heart, kidney, intestine, and brain. The brain, for example, is the most sensitive organ to reductions in blood supply due to its underlying high metabolic rate per unit weight. The brain also has an absolute requirement for glucose as an energy substrate and lower levels of protective antioxidants. As the largest organ in the body, the skin has not been studied as extensively as other organs in relation to ischemia and reperfusion injury. Ischemia reperfusion injury can have an effect on remote organs as well as the ischemic local organ. Multiple organs are injured in clinical conditions such as circulatory arrest, sickle cell anemia, sleep apnea, and during trauma and resuscitation. The mechanism of action for remote organ injury has been identified as the same factors implicated for local organ dysfunction such as reactive oxygen species formation, leukocyte activation, and inflammatory mediators. These circulating factors are responsible for the distant organ effects. The overall total organ injury sustained during prolonged ischemia followed by reperfusion is therefore attributable to an ischemic component, followed by a second component after reestablishing blood flow [2] (Fig. 2.4). Tissue-specific tolerances and confounding clinical conditions account for the variable responses noted in individual patients.
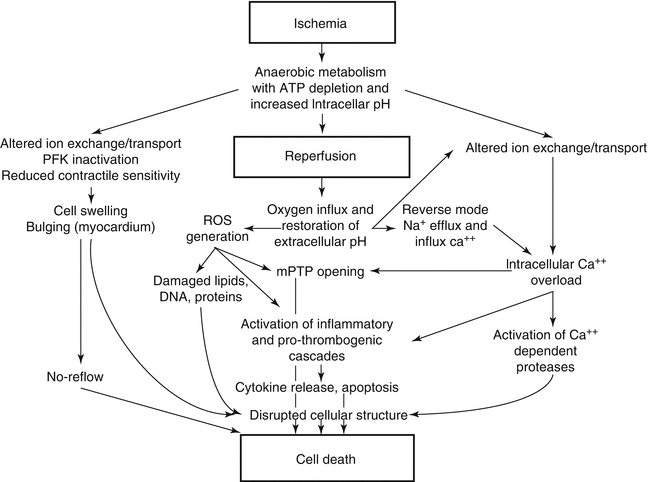
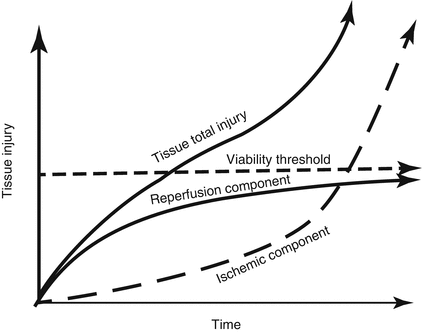
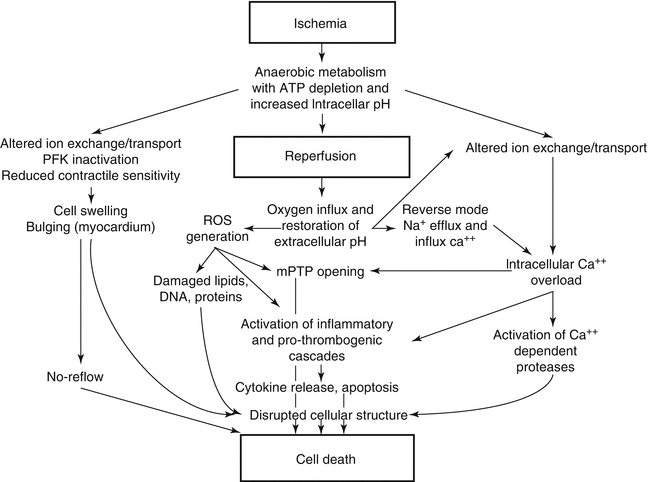
Fig. 2.3
Major pathologic events contributing to ischemic (upper panel) and reperfusion (middle panel) components of tissue injury, with overall integrated responses to I/R injury summarized in the bottom panel (Reprinted with permission from Kalogeris et al. [2])
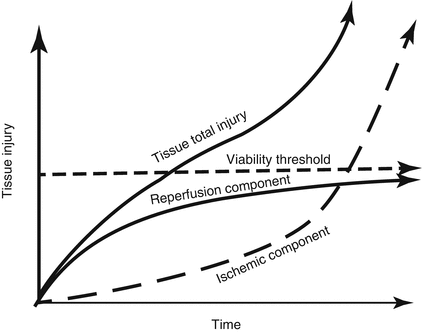
Fig. 2.4
Total injury sustained by a tissue subjected to prolonged ischemia followed by reperfusion (I/R) is attributable to an ischemic component and a component that is due to reestablishing the bold supply. At the onset of prolonged ischemia, two separate pathologic processes are initiated. The first are processes of tissue injury that are due to ischemia per se. The second are biochemical changes during ischemia that contribute to the surge in generation of reactive oxygen species and infiltration of proinflammatory neutrophils when molecular oxygen is reintroduced to the tissues during reperfusion particularly the initial phases. For a treatment to be effective when administered at the onset of reperfusion, reestablishing the blood supply must occur before damage attributable to ischemia per se represents a major component of total tissue injury. Therapeutic approaches that target pathologic events contributing to both the ischemic and reperfusion components of total tissue injury, such as ischemic or pharmacologic preconditioning, should be more effective than therapies administered when the blood supply is reestablished, which limit only the progression of reperfusion injury (Reprinted with permission from Kalogeris et al. [2])
The skin and chronic wounds are dependent on the microcirculation for oxygen, nutrients, and the elimination of metabolic wastes. Decreased quantities of oxygen lead to decreased bacterial killing by leukocytes, decreased collagen production, and decreased epithelialization. A patient may have compromised macroflow but, due to compensatory mechanisms such as the development of collateral flow, may be able to heal a wound. In a study of 111 patients with non-reconstructable vascular disease, the microcirculatory assessment was predictive of ultimate limb salvage [3]. The clinician can treat the microcirculation through the use of various energy-based modalities (i.e., ultrasound, electrical stimulation) that can increase angiogenesis and local blood flow to the wound bed [4]. A concept known as the push-pull theory has been presented by the authors as a working theoretical construct [5]. The push is achieved by the macrovascular-based arterial reconstruction. Other forms of “push” include increasing cardiac output, volume resuscitation, and the use of medications in the treatment of shock. Regardless of any potential negative side effects from revascularization, the first treatment option is to rapidly restore flow. The “pull” is essentially created by decreasing peripheral resistance and increasing the quantity of available capillaries, a process known as capillary recruitment. After the initial increase in microcirculatory flow, mediated by nitric oxide release from the endothelium within the microcirculation, a second phase of increased microcirculatory flow is achieved through the process of angiogenesis. Local microcirculatory perfusion can also be influenced by both vasoconstriction and adequate volume status. Noxious stimuli such as hypothermia, stress, pain, and depression can all lead to increased sympathetic tone and subsequent decreased tissue perfusion. Smoking, through the action of nicotine, can also result in decreased microcirculatory flow.
After a successful vascular intervention, the wound team needs to monitor the healing trajectory and measure the microcirculatory status. The improvement in macro-level perfusion can be the result of bypass surgery, an interventional vascular procedure, medical management of fluid status or blood pressure, or improvement in cardiac function. The presence of chronic ischemia results in an adaptive peripheral arteriolar vasodilation in various organ systems and the lower extremity. In fact it has been demonstrated both in human and animal modeling that small episodes of ischemia can actually improve tissue tolerance to reperfusion, a concept known as ischemic preconditioning. The microcirculation can become both structurally and physiologically altered during the ischemic state. Reestablishing macroflow results in a large volume of blood entering a dysfunctional microcirculation with resulting problems such as “revascularization edema,” which can further compromise tissue perfusion. Caselli demonstrated, for example, that transcutaneous oxygen levels increase over a 4-week period after successful revascularization but do not demonstrate consistent elevations except for a brief initial rise, when revascularization is unsuccessful [6].
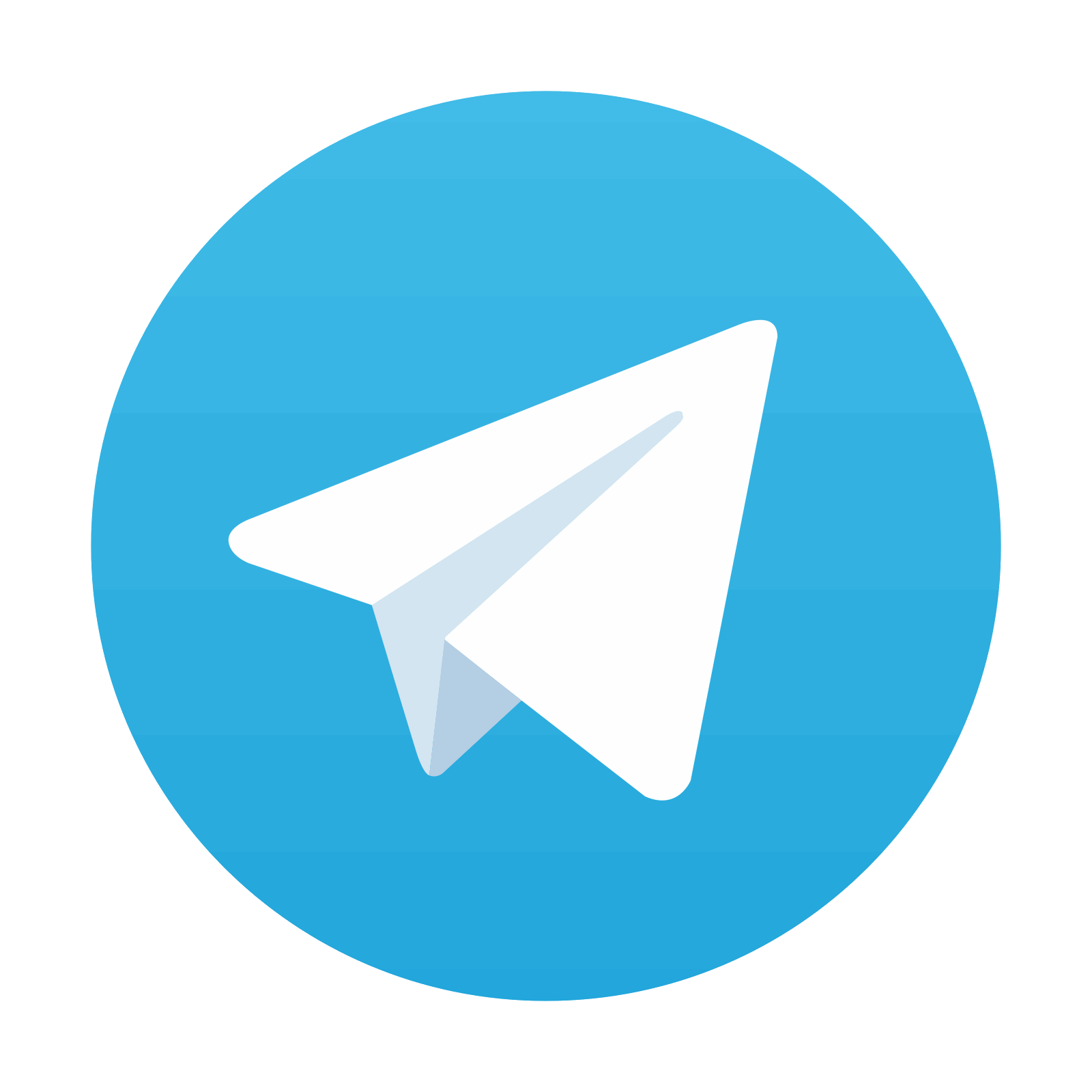
Stay updated, free articles. Join our Telegram channel

Full access? Get Clinical Tree
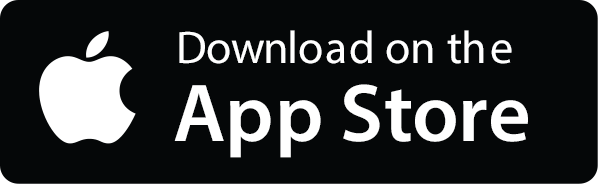
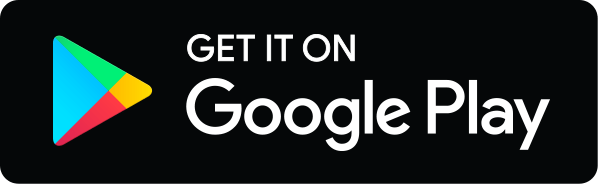