Fig. 5.1
Example of HPV testing using computer assisted analysis of HPV-PCR fragments obtained from skin lesions. Lanes: M DNA molecular weight marker (ϕx174 RF DNA), R reagent control, S1 and S2 samples from the patient, P HPV positive control DNA HPV-PCR fragments generated by nested EV-HPV PCR assay system [17] can be seen in the specimens (S1 and S2) and in the positive DNA control. The HPV fragments from the specimens were cloned into a PCR cloning vector. Several HPV fragment containing clones were isolated and the purified plasmids were sent for sequencing. The clones from samples (S1 and S2) showed high homology (98 %) to the HPV 20 prototype DNA sequences (NCBI-GenBank). In accordance with established guidelines, a nucleotide sequence was identified as an HPV type if it shared over 90 % L1 region homology with a known type of HPV from the databank. Interpretation of the HPV testing result: Infection with HPV type 20 was detected in the skin lesions. (Courtesy of Dr. Stephen K. Tyring and Dr. Peter Rady, Center for Clinical Studies, Houston, TX)
The interpretation of a molecular-based positive test in a skin biopsy requires correlation with the histological appearance of the lesion. Of note, although the risk of development of cutaneous SCC is higher in the immunosuppressed population [25] and HPV DNA has been detected in primary and metastatic SCC [26], both facts indicating a possible pathogenic link between HPV and nonmelanoma skin cancers, the role of HPV in cutaneous SCC induction remains unclear [27].
Human Herpesvirus
Herpes Simplex/Varicella Zoster
Molecular techniques can be helpful in the diagnosis of atypical herpes virus infections. Conventional microscopic findings in these cases may not be specific and immunohistochemical studies depend on the presence of infected cells in the sections examined.
ISH techniques have been used for the identification of varicella zoster virus in FFPE tissue [28]. Routine PCR-based methods performed on biopsies [29] and swab samples are being increasingly used to detect herpes virus DNA [30]. A proposed algorithm is to use immunofluorescent tests—which are cheaper and faster—at first screening and then run PCR on negative samples [31]. The use of internal controls is emphasized in order to rule out false negative results [32].
Epstein–Barr Virus
Epstein–Barr virus (EBV, human herpesvirus 4) cutaneous infection is associated with multiple disorders, ranging from acute syndromes such as infectious mononucleosis, Gianotti–Crosti syndrome, and Kikuchi disease to chronic infections such as hydroa vacciniforme, hypersensitivity to mosquito bites, and oral hairy leukoplakia [35]. B-, T-, and NK-lymphoproliferative disorders and lymphomas are also associated with EBV infection.
Immunohistochemical studies for detection of EBV viral proteins (EBNA1, EBNA2, LMP1, LMP2, among others) are used in some instances. The interpretation of these stains should always be made in the context of the histological findings, since in some EBV-related conditions the expression of these proteins may be only focal or even undetectable by immunohistochemistry.
EBV-encoded RNAs (EBER1 and EBER2) are transcripts associated with latent infection that are expressed in very high levels in virtually all infected cells [36]. EBER ISH is regarded as the gold standard assay for defining a lesion as EBV-related [36] (Fig. 5.2). False positive interpretations have been related to nonspecific staining and cross-reactivity with other materials [37]. False negative results due to RNA degradation can be avoided by running a parallel internal control [38]. It is important to consider that rare conditions, such as oral hairy leukoplakia shows downregulation of EBER; [39] again, interpretation of the test should be done correlating the results with the histopathological findings.
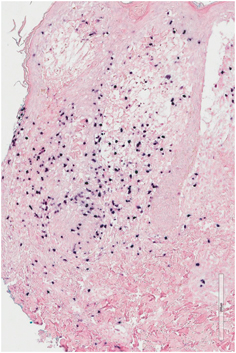
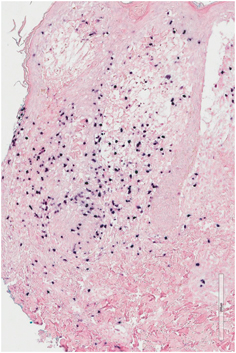
Fig. 5.2
Cutaneous extranodal NK/T cell lymphoma, nasal type. This skin biopsy shows positive cells for EBER, as occurs in most of these aggressive lymphomas (EBER in situ hybridization (ISH), 10x)
PCR amplification of latent EBV transcripts using RNA extracted from crusts of hydroa vacciniforme patients has been reported [40]. qPCR can be applied to identify EBV DNA; [41] the quantification of EBV DNA using qPCR on biopsies has been used for determination of viral load [42]. Detection of EBV DNA by PCR methods can be advantageous over EBER ISH in cases in which there is poor quality RNA or the EBV infection lacks EBER transcripts [36].
EBV episomal DNA, which is passed from infected cells to their daughters, is used as a marker for tumor clonality. Southern blot assays of the viral terminal repeat fragment (unique for every infecting EBV virion) will show a single band in cases of malignant neoplasms, thus demonstrating a monoclonal proliferation [43].
Human Herpesvirus 8
Immunohistochemical studies using monoclonal antibodies directed against human herpesvirus 8 (HHV-8) latent nuclear antigen-1 have been demonstrated to be 99 % sensitive and 100 % specific for Kaposi sarcoma lesions [44, 45].
PCR assays are both highly sensitive and specific for the detection of HHV-8 and permit quantitation of viral load [46, 47]. One caveat in the interpretation of these molecular tests is the detection of HHV-8 DNA in skin lesions due to concurrent viremia and not due to viral replication within lesional cells [48]. Correlation with histological and immunohistochemical findings is needed for an adequate interpretation of molecular tests results .
Human Polyomavirus
The demonstration of Merkel cell polyomavirus (MCV) DNA integration into about 80 % of Merkel cell carcinomas (MCC) [49] started a new exciting era for the virus-induced cancer pathogenesis field. It seems that MCC tumors may have different behavior depending on the presence or absence of MCV [50].
To date, nine human polyomaviruses are known. Besides MCV (the fifth identified polyomavirus), four other polyomaviruses have been identified: HPyV6 and HPyV7 as part of common skin flora, [51] TSaPyV in lesions of virus-associated trichodysplasia spinulosa, [52] and HPyV9 in serum of a kidney transplant patient [53].
Immunohistochemical methods can detect MCV proteins in most MCCs [54]. These methods use antibodies directed against MCV large T antigen (LT) [55]. Some cases, positive for MCV by PCR, may be negative for LT expression by immunohistochemistry [56]. A subset of these cases expresses MCV small T antigen (sT) [57].
Human T cell Lymphotropic Virus Type 1
Skin infection by human T cell lymphotropic virus type 1 (HTLV-1) is associated with infective dermatitis and adult T cell leukemia/lymphoma (ATLL) [60]. It is possible that HTLV-1-infected cells, along with activated T cells, infiltrate the skin of patients with infective dermatitis [61, 62]. This disease is considered as an early marker for the development of ATLL [63, 64].
The identification of integrated HTLV-1 proviral sequences in ATLL tumor cells has been extensively documented [65]. Cutaneous tumors in ATLL may be clinically and histopathologically indistinguishable from HTLV-1-negative lymphomas [66]. Besides serology, demonstration of monoclonal HTLV-1 proviral integration by Southern blot can be helpful in the diagnosis of ATLL [67]. Other cutaneous T cell lymphomas have been shown to lack HTLV-1 proviral DNA integration [68]. HTLV-1 proviral DNA load seem to be higher in smoldering ATLL than cutaneous ATLL, the last possibly representing a separate clinical entity [69]. RT-PCR can be used to identify the HTLV-1 tax and basic leucine zipper factor (HBZ) transcripts [70].
ISH using a HTLV-1 proviral probe has been applied to FFPE tissue sections to identify HTLV-1 proviral DNA, with reported better results than PCR [71]. More recently, ISH using a peptide nucleic acid (PNA) probe directed against the HTLV-1 HBZ gene (whose transcripts have been detected in all ATLL cells [72]) has been found to be a reliable test for HTLV-1 detection in pathology samples [70].
Bacterial Infections
Mycobacteria
Mycobacterium tuberculosis
Tuberculosis continues to be a health problem around the world, affecting not only developing countries but also showing increasing incidence in countries like England [73]. Diagnosis is always based on the isolation of Mycobacterium tuberculosis on culture, not a difficult task if the material is sputum, but quite difficult in the case of tegument tissues such as pleura or skin. Even the visualization of the bacteria on regular tissue cuts becomes problematic in such organs; therefore it is clear that better diagnostic methods are required in particular cases.
Nucleic acid amplification tests (NAATs) are designed to detect the smallest amount of either DNA or RNA through the amplification of specific sequences to the point of allowing detection by blotting [73]. PCR-based methods are now commercially available for the diagnosis of tuberculosis. These include the Roche Amplicor Mycobacterium tuberculosis test (PCR target amplification of part of the 16S rRNA gene, followed by the colorimetric detection of the PCR product) and the Gen-Probe Amplified Mycobacterium tuberculosis Direct Test (MTD), which is an isothermal transcription amplification method using ribosomal RNA (rRNA) as the target. A third method, called BD Probe Tec (multiple strand displacement system) uses isothermal amplification with DNA as substrate. Many institutions have also developed their own tests based on the IS6110 insertion sequence. Unfortunately, use of these tests also carries potential lack of specificity (since the same sequence is also present in other Mycobacterium) and sensitivity (since not all M. tuberculosis subtypes carry this sequence.) Other sequences used as target include MBP64, rpob and hsp65. Many of the methods now available also provide information regarding the sensitivity to drugs, such as rifampin, which is used as a criterion for the diagnosis of multidrug-resistant tuberculosis (MDRTB) .
Most NAATs have used sputum samples and none of them is currently approved for its use in fixed tissue. Even more, lack of standardization of PCR methods performed of FFPE tissue makes interpretation of the results quite difficult and may explain the variability in sensitivity and specificity when these methods are applied to the diagnosis of tuberculosis in extrapulmonary sites such as lymph nodes [74, 75], pleura [76, 77], and skin [78–80]. Noteworthy is the fact that PCR may lack sensitivity under these circumstances, a concept not usually associated with the method. Possible explanations may include the presence of tissue inhibitors and lost of viability of the genetic material in old formalin-fixed tissue [81].
All said, the study of entities, such as tuberculides, by using PCR-based methods has been crucial for a better understanding of the physiopathology of tuberculosis affecting non-pulmonary organs. The study by Baselga [82] on PCR in the diagnosis of erythema induratum of Bazin helped to develop the concept of cutaneous tuberculosis as a spectrum whose ends are the multibacillary and paucibacillary forms, with distinct clinical presentations. Molecular biology applied to the study of tuberculosis helps us not only as a diagnostic test but also as a tool to reformulate our concepts of the disease .
Mycobacterium leprae
Molecular studies are especially helpful in the diagnosis of Mycobacterium leprae infections, since the bacteria cannot be cultured in artificial media. Clinical presentation and demonstration of acid-fast bacilli in smear preparations or skin biopsies are routinely used for diagnosis. Traditional methods, however, require at least 104 organisms/g of tissue to obtain reliable results [83]. In paucibacillary presentations of the disease (tuberculoid, some indeterminate forms) therefore, the bacilli are particularly difficult to demonstrate. PCR-based methods have long been used for detection of M. leprae [84, 85].
A nested PCR assay developed and tested at the Centers for Disease Control utilizing four nested primers, designed to amplify portions of the M. leprae groEL gene (corresponding to sequences found in M. leprae gene but not in M. tuberculosis) produced a result within 8 h. Positive signals for M. leprae could be achieved with just 0.003 pg of genomic DNA and as few as 20 organisms in crude lysates. The use of nested primers reduces nonspecific amplification, with increased sensitivity due to replenishment of reagents after every 25 cycles, and increased specificity as four primers need to bind for there to be successful amplification [84]. An RT-PCR detection system targeting the 16S rRNA of M. leprae improves on this sensitivity, making it possible to detect as few as 10 organisms in crude lysates. In this system, 53 % of specimens negative by smear are positive by RT-PCR. Furthermore, due to the rapid degradation of 16S rRNA on mycobacterial cell death, an rRNA-based detection system can distinguish between viable and nonviable M. leprae, and can thus be helpful in assessing the efficacy of instituted medications [86]. This is a key concern in leprosy where active mycobacterial infection versus persistent skin changes in the absence of infection with improved immunity can be challenging to discern (type 1 reaction). Furthermore, nested PCR protocols have been utilized in the differentiation between M. leprae and the newly described Mycobacterium lepromatosis, of clinical importance due to the tendency for the angioinvasive M. lepromatosis to cause a type of diffuse lepromatous leprosy with severe vascular involvement, namely Lucio phenomenon, which is usually associated with higher mortality [87, 88] .
Staphylococcus aureus
Staphylococcus aureus is a pathogenic bacteria, with a wide variety of presentations in the skin, including superficial infections such as impetigo and folliculitis and deep infection including ecthyma, cellulitis, and abscess formation [89]. S. aureus is an angioinvasive bacteria which causes secondary skin involvement in bacteremia and is frequently implicated in chronic inflammatory conditions, such as dissecting cellulitis of the scalp and hidradenitis suppurativa, contributing to the morbidity of the disease. Targeting bacterial resistance mechanisms and testing drug susceptibility is key to the management of S. aureus infections. Methicillin-resistant S. aureus (MRSA) was first described in 1961, and its prevalence in both community and healthcare settings continues to increase [90]. The mecA gene encodes PBP-2a, a penicillin-binding protein that confers resistance to methicillin and related beta lactam antibiotics [91, 92]. The mecA gene belongs to a chromosomal element, the staphylococcal cassette chromosome (SCCmec). The protein A (SpA) virulence factor is encoded by the spa gene, enabling S. aureus to evade host immune responses. There is high variability of gene sequences within the Xr region of the spa gene, which is thus used to type strains [93]. Multiple qPCR kits are commercially available for the detection of MRSA, such as the MRSA diagnostic assay for Skin and Soft Tissue Infection (Xpert™, Cepheid, Sunnyvale, California) [94]. In bone and joint infection samples tested with Xpert™, the sensitivity and specificity for the detection of MRSA were both 100 %, with Gram stains positive in only 33 % of cases, and a median turn around time of 72 min compared to 79 h for culture [95]. The Xpert™ kit is a multiplex PCR assay that simultaneously detects three targets (spa, SCCmec, and mecA) within MRSA. It is able to distinguish between staphylococcal infections (MRSA, methicillin sensitive S. aureus, coagulase negative staphylococci) with its main limitation being its primer sets limited to staphylococcal organisms and thus inapplicability to a wider range of bacterial infections .
Treponema pallidum
The rate of primary and secondary syphilis in 2013 was double the lowest ever rate in 2001, representing a reemergence of this disease [96]. The causative agent, Treponema pallidum cannot be cultured in vitro. Darkfield microscopy for direct visualization of spirochetes and serologic antibody tests during early phases of disease, have low sensitivity and specificity [97]. Immunofluorescence staining methods have long been used in the detection of T. pallidum. A fluorescent antibody test applied to tissue fixed in formalin has significantly improved the detection of T. pallidum, but its limitation is that it requires experienced analysts for interpretation [98, 99]. Immunohistochemical assays utilizing monoclonal or polyclonal antibodies are sensitive, but can produce false positives in the setting of other spirochetes including Borrelia species with increased specificity when anti-T. pallidum antibodes are used [100, 101]. Molecular methods that amplify PCR products have been examined, but are limited by poor specificity [102]. In one such study the sensitivity for PCR detection of T. pallidum was 75 % compared to 91 % with immunohistochemistry [101, 103]. This study used primers to amplify a 260 based pair (bp) fragment of the gene encoding the integral membrane lipoprotein of T. pallidum, with PCR products hybridized to the tp47 gene (which does not have homology to any bacterial or eucaryotal proteins) between the 658 and 648 bp by Southern blot. PCR and immunohistochemistry were performed in the same sample in this study, indicating that combining the two methods may optimize sensitivity and specificity of T. pallidum detection.
Fungal Infections
Superficial Fungal Infections
Dermatophytoses are common superficial fungal infections limited to the keratinized integument, that is, the nail, stratum corneum of skin and hair. They are caused primarily by fungi of the genera Trichophyton, Epidermophyton, and Microsporum. Regardless of the causative species, dermatophytoses are characteristically either pruritic or scaly eruptions, although more protean and widespread presentations may be seen in the immunocompromised host. Whereas dermatophytes have low pathogenicity, persistent infection has adverse effects on the quality of life [104]. Furthermore, dermatophyte infection frequently results in a compromised skin barrier creating a portal of entry for other pathogens, with tinea pedis strongly associated with bacterial cellulitis of the lower limb [105]. It can be particularly difficult to identify the causative microorganism in nail fungal infections which are exquisitely recalcitrant to topical therapy. Traditional methods for detection include light microscopic analysis of wet preparations of involved tissue, such as nail clippings, incubated with potassium hydroxide to digest keratin, leaving the fungal chitinous wall intact. Artifacts are difficult to distinguish from fungal elements complicating interpretation, with a sensitivity between 83 and 88 % and specificity of 84–95 % in some studies [106, 107]. Another popular method is to expose nail clippings treated with KOH, or biopsy specimens stained with the PAS to the calcofluor white or blankophor fluorescent stains which bind to the fungal wall polysaccharide chitin, made of glucosamine residues, and enhance the contrast between fungal walls and artifactual debris. The reported sensitivity and specificity with calcofluor white with these methods ranges from 80 to 92 % and 72 to 95 % respectively [106–109]. A pitfall is that protozoa are also highlighted by calcofluor white, although morphology is helpful in distinguishing the two entities [110]. Culture remains the gold standard for fungal speciation. The problem with culture as a gold standard is the possibility of fungal contaminants during culture, fastidious and slow growing fungi resulting in false negatives (as high as 30 %), and delayed results following laborious culture protocols [111]. The correct identification of the causative microorganism in onychomycosis, for example, is of importance, as Trichophyton rubrum is susceptible to oral terbinafine, whereas Candida spp. are not. Fluconazole is efficacious for candidal onychomycosis and itraconazole is recommended for onychomycosis due to nondermatophyte molds such as Scytalidium spp. [112].
Trichophyton rubrum
T. rubrum is most frequently implicated in superficial fungal infections [113]. Timely and targeted therapy would be aided by rapid and accurate diagnostic techniques. A two step, 15-min procedure for extraction of DNA from nail specimens followed by multiplex PCR has been designed [5, 114]. This duplex protocol uses two primers: one detecting the conserved DNA fragment encoding chitin synthetase 1 against dermatophytes in general, designed based on nucleotide sequencing of different dermatophytes found in the NCBI nucleotide database, and the second against the internal transcribed spacer 2 (a species specific ribosomal transcript region) for T. rubrum, and tested on dermatophyte and nondermatophyte reference strains and clinical isolates [115, 116]. Thus, a dermatophyte will show a positive band at the pandermatophyte 366 bp, and if that dermatophyte is T. rubrum, there will also be a strong positive band at the 203 bp. This PCR protocol has been externally validated, with 44 % of samples positive for dermatophyte by PCR compared to 34 % by culture. PCR also confirmed T. rubrum in 98 % of previously cultured cases. The kit is commercially available with positive and internal controls provided by the manufacturer (Dermatophyte PCR kit, Statens Serum Institut, Copenhagen, Denmark) requiring 1 day for laboratory diagnosis [117].
Deep Fungal Infections
Deep fungal infections can be categorized as primary pathogens that are inherently virulent, and secondary or opportunistic pathogens that are pathogenic in the context of an immunocompromised host. Timely diagnosis is crucial and targeted therapy based on species identification may be life saving. Concomitant culture and culture independent assays, such as galactomannan and (1→3)-β-D-glucan antigen testing, of blood (unfortunately frequently negative in invasive fungal infection) and other accessible compartments in conjunction with biopsy of involved skin are key, with the goal being rapid detection and source control. For evaluation of cutaneous infection, whether primary or secondary, the conventional diagnostic approaches are tissue culture and histopathological examination. The same limitations identified in superficial fungal infections regarding sensitivity, specificity, interobserver variability, and lead time to diagnosis apply when evaluating deep fungal infections [118]. Successful identification on histopathology alone often depends on the abundance of organisms and the stage of infection. Additionally, a fungal organism may be visible on histopathology, but its identification may be limited if fresh tissue was not submitted for culture simultaneously. In cases where there was no isolation of organism on culture or there are significant discrepancies between the organism growing in culture and that seen on histopathology, doubts may rise about the certainty of the actual pathogen. Molecular techniques that can be evaluated in conjunction with skin biopsies are thus attractive .
Aspergillus spp
Analysis from 23 transplant centers in the USA revealed invasive aspergillosis to be the most commonly encountered invasive fungal infection in the hematopoetic stem cell transplant population, surpassing invasive candidiasis [119]. Of note, non-fumigatus Aspergillus species resistant to conventional amphotericin, such as Aspergillus terreus, are becoming increasingly prevalent [120]. A plethora of publications exist evaluating molecular methods for rapid detection of Aspergillus spp. Nucleic acid amplification based methods including nested PCR, qPCR, multiplex PCR followed by DNA microarray, and sequencing based methods in theory allow detection, identification, and strain typing [118].
These methods are designed as broad spectrum fungal detection systems, using conserved sequences to identify a broad range of fungal species, yet enough polymorphisms to simultaneously allow species identification [121]. The source material can be fresh tissue or paraffin embedded tissue but processing unavoidably destroys architecture and morphology (e.g., pattern of septation and branching of filamentous fungi). In practice, these methods often rely on mechanical methods to disrupt the fungal cell wall to access DNA for extraction that pose a risk of carry over contamination and the potential for contaminated reagents, and are not standardized for clinical sample size required for sensitivity of detection, test performance (sensitivity, specificity), and interpretation of results [122]. Combinations of analyte specific reagents (Luminex Molecular Diagnostics, Toronto, Canada) can be customized to test for a panel of regionally relevant fungi and can be tailored for testing of solid and liquid media. For example, an 11 plex panel representing 11 common clinically relevant molds, 4 of which were Aspergillus spp., has been tested using the Luminex multiplex PCR incubated with primer pairs for the 11 molds, followed by hybridization to microbead bound capture probes for fluorescence-based detection from fresh tissue samples [123]. Coinfection with multiple fungi can be detected by this method, if they are represented in the primer sets .
Fungal identification by comparison of the test fungus’s characteristic protein expression pattern with species specific pattern expression through matrix assisted laser desorption/ionization time of flight mass spectrometry(MALDI-TOF-MS) is commercially available (Bruker Maldi Biotyper CA System, Bruker Daltonik, Germany) [124, 125]. This technique relies on standardized protein extraction practices to generate reproducible profiles [126]. This technique fails to adequately address mixed fungal infections, but can be used to assess drug sensitivity, by comparing changes in the spectra on exposure to the drug [127]. Fluorescence in situ hybridization (FISH) methodologies to localize fungal rRNA to pathology on FFPE tissue are being explored and are highly attractive for their ability to examine pathogens in histopathologic context with preservation of tissue architecture and pathogen morphology. However, current data suggest that PCR techniques are more sensitive than FISH in detecting invasive fungi. Current FISH methodologies broadly target fungal rRNA, the most conserved nucleic acid sequences, with modifications, such as utilization of locked nucleic acids which hybridize strongly to their complements and are thermally stable [128]. Weak signaling when rRNA is not abundant or probes are inadequately developed are potential pitfalls. Poor performance of ISH methods in necrotic tissue is also a limitation, however, FISH remains a promising option [129].
Alternaria spp
Alternaria is a ubiquitous pigmented fungus, found as spores in soil, air, and plants that typically do not cause infection in humans, with infection often occurring in the setting of traumatic inoculation [130]. It is an emerging fungal pathogen, rapidly fatal in immunosuppressed hosts although itraconazole may be effective when rapidly initiated. A rapid growing mold which may or may not be pigmented is identified on culture; however, failure to sporulate or pigment on multiple media is a frequently encountered problem, making culture-based identification almost impossible [131]. Furthermore, the most clinically relevant species, Alternaria alternata and Alternaria infectoria are not pigmented in tissue, thus a phaeohyphomycosis is unlikely to be considered even if the large yeast or septate hyphae are identified on histopathological examination. Successful identification of Alternaria spp. by amplification and subsequent sequencing of the Internal transcribed spacer (ITS) of the rRNA after failure to identify the mold evident on culture or on special stain analysis with PAS and GMS has been reported in multiple cases and illustrates the pivotal diagnostic role molecular methods can play [130–134].
Parasitic Diseases
Leishmaniasis
A great example of the utility of molecular techniques in diagnosing infectious diseases applies to leishmaniasis, the third most common vector transmitted disease worldwide. When dealing with tegumentary leishmaniasis, one has to confront a variety of clinical presentations, some of them easily confused with various other processes, infectious or not. To construct a solid diagnosis of tegumentary leishmaniasis it is required to visualize the parasite, either by direct exam of a smear, isolation in culture or by conventional histology in biopsy material. Indirect techniques, such as the intradermal test or Montenegro reaction, are significant when dealing with patients that are foreign to the endemic areas, but not in natives. The interpretation of smear preparations requires expertise, something expected in laboratory workers of endemic areas but unlikely in other locations. This last situation is far from being exceptional due to the increasing number of cutaneous leishmaniasis seen in travelers, such as tourists or army personnel, thus the increasing importance of molecular methods for diagnosis.
The molecular technique most commonly used is PCR amplification of specific primers targeting either the kinetoplast or rRNA gene (Fig. 5.3), ITS, mini-exon genes, and specific gene sequences (e.g., glycoproteins, heat shock proteins, and cysteine proteinases) [135]. Multiple publications have corroborated the validity of the method for diagnostic purposes [136, 137]. The technique has been simplified to make it more accessible to the caregivers in primary care settings [138–141]. qPCR can provide additional information, such as parasitic load or determination of species, contributing to the treatment strategies as well as to epidemiology studies on this subject [142, 143]. As the response to medical treatment may vary depending on the species of Leishmania involved, the utility of molecular biology becomes more manifest when assisting such species identification [144, 145].
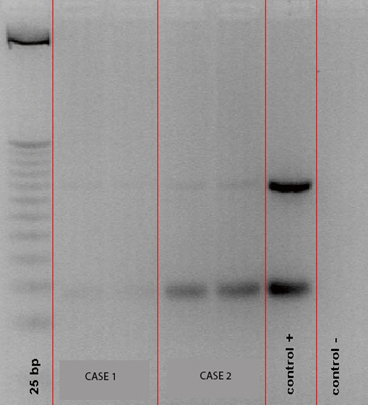
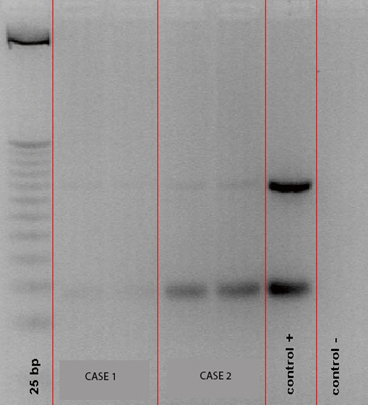
Fig. 5.3
Gel electrophoresis showing PCR amplification of kinetoplastid DNA (kDNA) specific for Leishmania spp. Lane 1 shows molecular weight control, lanes 2 and 3 are the patient samples, lanes 4 and 5 are positive and negative controls (Courtesy of Dr. J. Arevalo, Universidad Peruana Cayetano Heredia, Lima, Peru)
Onchocerciasis
Filariasis is caused by nematodes (round worms) which parasitize humans, residing in the lymphatics and subcutaneous tissue with resultant lymphedema and cutaneous lesions. Over 120 million people worldwide are affected by filariasis which can be extremely disfiguring and disabling [146]. Early institution of diethylcarbamazine during the microfilarial phase can effectively eradicate the nematode Loa Loa, whereas treatment with diethylcarbazine can cause life threatening systemic collapse when the infecting nematode is onchocerca, an adverse reaction known as the Mazotti reaction [147]. Thus, speciation of the causative nematode in filariasis is key for administration of the correct treatment. Traditional diagnosis of nematodes depends heavily on morphology, specifically, by recognition of microfilaria on skin biopsies and thick blood smears. These methods are labor intensive, time consuming, and require experienced microscopists who can discern between the multiple species present in a region and between coinfecting nematodes in the same individual, making accuracy challenging [148, 149]. Antibody testing has its pitfalls, for example, an enzyme linked immunosorbent assay (ELISA) was unsuccessful in Brazil due to cross reactivity between Onchocerca volvulus and Mansonella ozzardi [150]. A nested PCR protocol tested on skin biopsies and whole blood samples holds promise. In this protocol, four PCR primers with partial sequences for 18S (small subunit rRNA), ITS-1, 5.8S and ITS-2 are used, thus amplifying the ITS of filarial species [151, 152]. Differentiation between species and detection of coinfection is possible as the ITS regions vary between species and thus yields amplicons of different sizes on gel electrophoresis, for example, O. volvulus
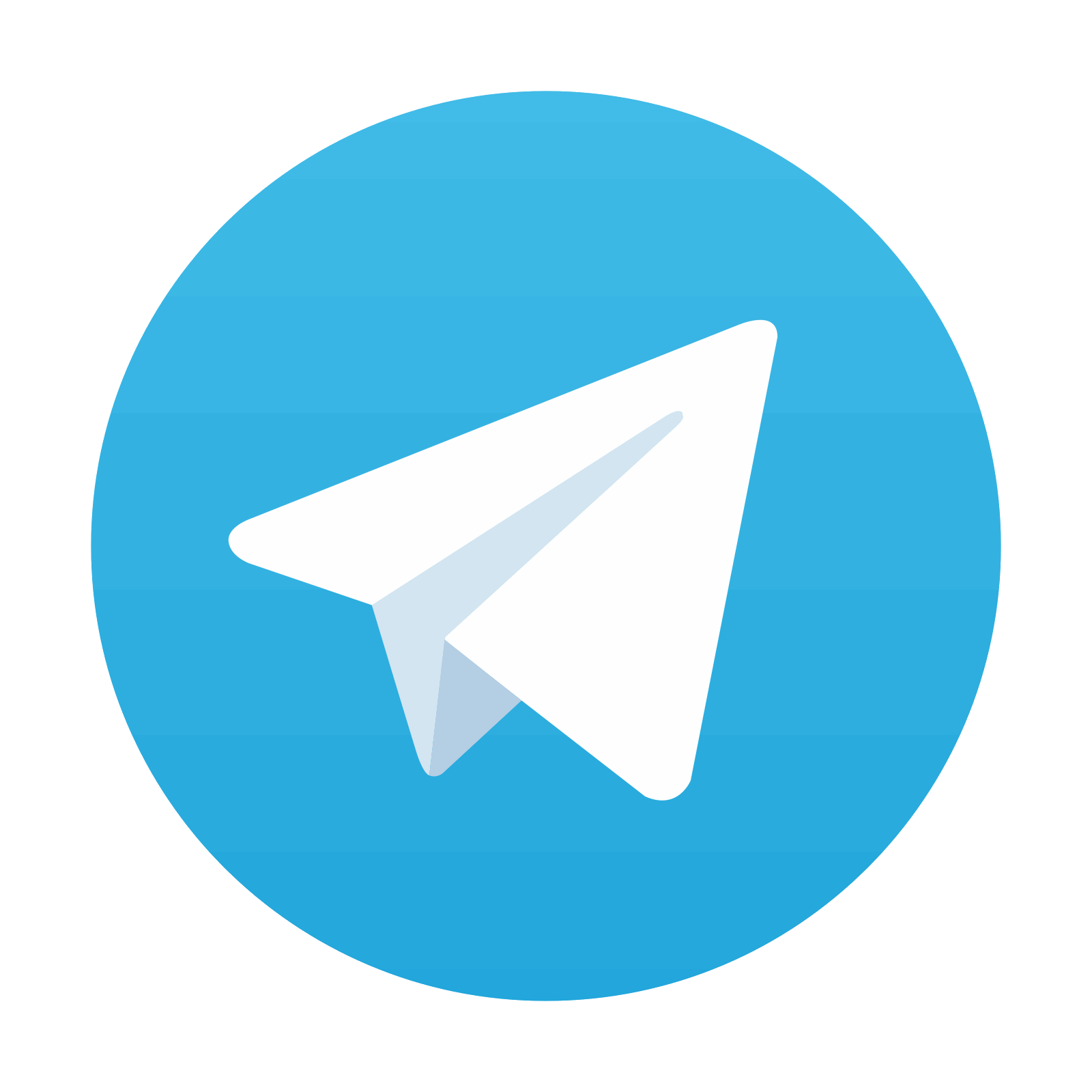
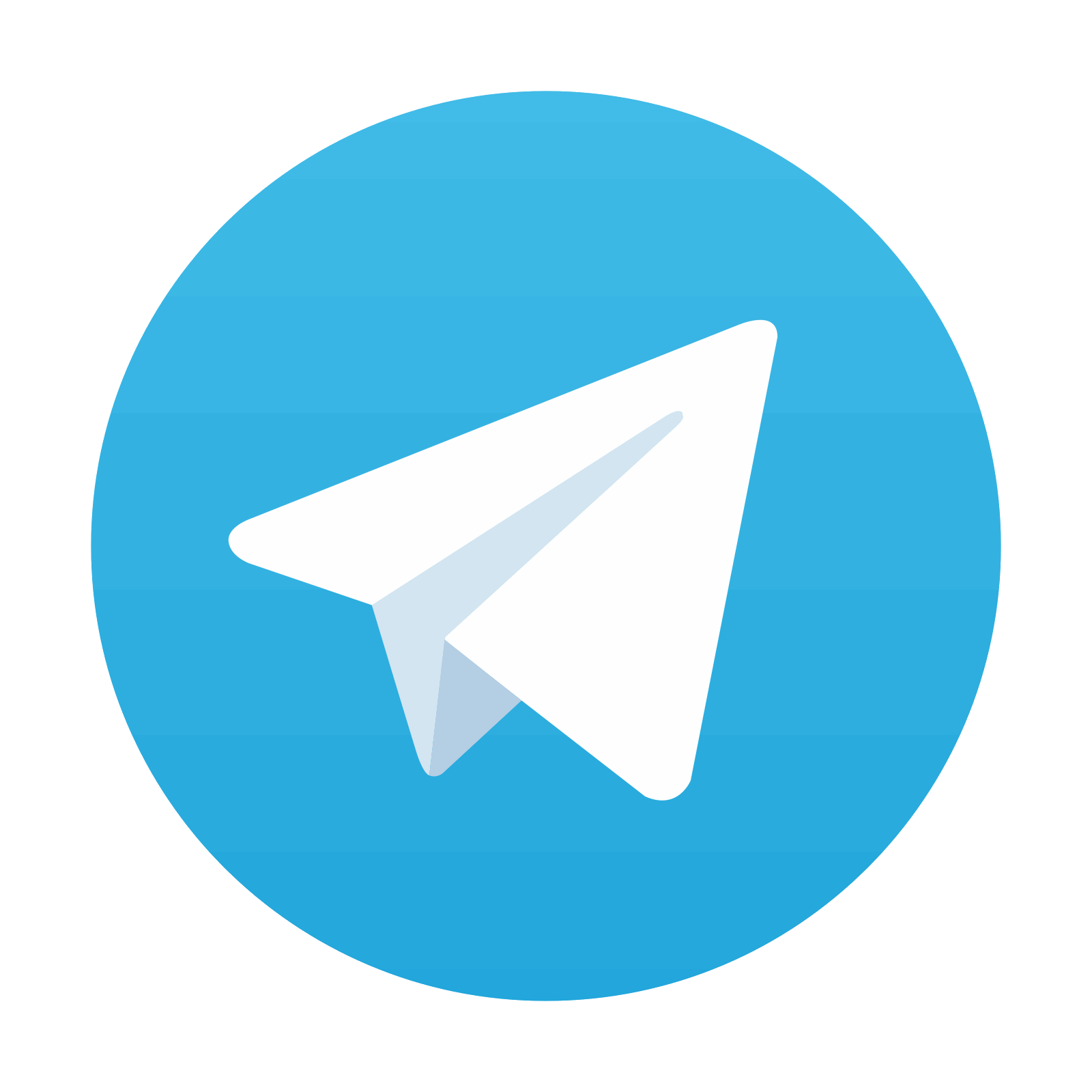
Stay updated, free articles. Join our Telegram channel

Full access? Get Clinical Tree
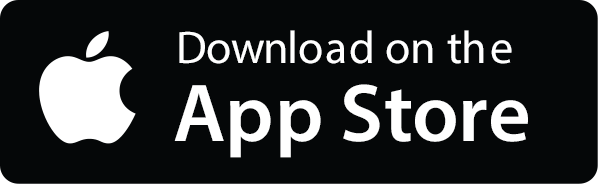
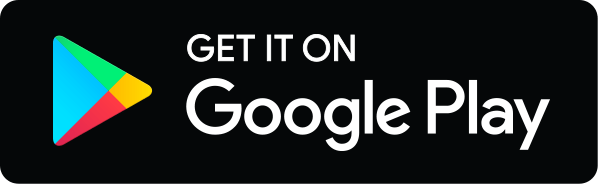